Abstract
Novel tamarind seed polysaccharide (TSP)-alginate mucoadhesive microspheres were prepared using TSP and alginate as blend in different ratios with different calcium chloride (CaCl2) concentration as a cross linker by ionotropic gelation. The prepared microspheres were of spherical shape having rough surfaces, and average particle sizes within the range of 752.12 ± 6.42 to 948.49 ± 20.92 µm. The drug entrapment efficiency of these microspheres were within the range between 58.12 ± 2.42 to 82.78 ± 3.43% w/w. Fourier transform infrared (FTIR) studies indicated that there were no reactions between gliclazide, and polymers (TSP, and sodium alginate) used. Different formulations of gliclazide loaded TSP-alginate microspheres showed prolonged in vitro release profiles of gliclazide over 12 hours in both stomach pH (pH 1.2), and intestinal pH (pH 7.4). It was found that the gliclazide release in gastric pH was comparatively slow and sustained than intestinal pH. These TSP-alginate microspheres also exhibited good mucoadhesivity. The in vivo studies on alloxan-induced diabetic rats (Animal Ethical Committee registration number: IFTM/837ac/0160) demonstrated the significant hypoglycemic effect of selected formulation of TSP-alginate mucoadhesive microspheres containing gliclazide on oral administration. This developed gliclazide loaded new TSP-alginate mucoadhesive microspheres may be very much useful for prolonged systemic absorption of gliclazide for proper maintaining blood glucose level and advanced patient compliance.
Introduction
Microspheres hold a unique and special position in the area of bioadhesive drug delivery systems (Nerkar & Gattani, Citation2011). These have been recognized as one of the particulate delivery systems used to achieve sustained or controlled drug delivery of drugs into specific or targeted sites, and thereby improve bioavailability of drugs. Microspheres also offer advantages such as limited fluctuation within a therapeutic range, reduction in side effects, decreased dosing frequency and improved patient compliance (Basu & Adhiyaman, Citation2008; Comoglu & Gomul, Citation2008). Still, the success of microspheres for sustained or controlled delivery is limited due to their short residence time at the site of absorption. Therefore, it would be highly beneficial if any system is adopted to provide an intimate contact for the drug with the absorbing surfaces of mucous membranes, that is, mucoadhesion (Vasir et al., Citation2003; Chowdary & Srinivas Rao, Citation2005; Cevher et al., Citation2008). It is mostly achieved by the use of mucoadhesive polymers. Recently, the mucoadhesive polymers have drawn a great interest in the designing of oral drug delivery systems to prolong the gastric residence time for the dosage forms as well as to facilitate the intimate contact with underlying absorptive surface to enhance the oral bioavailability of drugs (Chowdary & Rao, Citation2004; Jain et al., Citation2004; Nayak et al., Citation2010a, Citation2010b). The basic lies behind the fact that increased bioavailability may be due to the higher amount of flux generated due to intimate contact between two surfaces for a longer time to attain sustained release profile of drug delivery over that of conventional dosage forms (Andrews et al., Citation2009).
Recent trends towards the use of natural polymers as novel drug carriers primarily remain attractive because of their easy availability, cost effectiveness, capability of chemical modifications, excellent biodegradability and acceptable biocompatibility (Satturwar et al., Citation2003; Sachan & Bhattacharya, Citation2009). Amongst various natural polymers, alginates have been found extensively used as matrix in various drug delivery applications due to its hydrogel forming properties (El-Kamel et al., Citation2003). Alginates are polysaccharides obtained from marine brown algae (Laminaria hyperborean, Ascophullum nodosum, Macrocystis pyrifera etc.) (George & Abraham, Citation2006), which are the monovalent form of alginic acid belonging to the family of linear copolymers, composed of two monomeric units, β-D-mannuronic acid (M) and α-L-guluronic acid (G). These residues are arranged in homopolymeric blocks (GG and MM) and heteropolymeric blocks (MG) (Smidsrod & Draget, Citation1996). Alginates undergo ionotropic gelation in aqueous solution in the presence of divalent cations like Ca2+, Ba2+, Pb2+, Cu2+, Cd2+, Zn2+, and the like and trivalent cation like Al3+, due to the ionic interaction and intermolecular bonding between the carboxylic acid groups located on the polymer backbone and cations (Davis et al., Citation2003; Shilpa et al., Citation2003). Though alginates have mucoadhesive property, but, the cross-linked alginate beads are usually fragile (Kroll et al., Citation1996; Llanes et al., Citation2000). Therefore, to formulate various cross-linked alginate mucoadhesive microspheres, blending of different mucoadhesive polymers is one of the most popular approaches in this endeavor. Again, blending with suitable polymers may improve the drug entrapment efficiency, which is found comparatively lower in alginate microspheres prepared by ionotropic gelation method.
One cheap and naturally derived polymer is tamarind seed polysaccharide (TSP) obtained from the seeds of Tamarindus indica L., a common tree of India and South East Asia. TSP is composed of (1 → 4)-β-D-glucan backbone substituted with side chains of α-D-xylopyranose and β-D-galactopyranosyl (1 → 2)-α-D-xylopyranose linked (1 → 6) to glucose residues (Jana et al. Citation2010). TSP is noncarcinogenic, biocompatible and extraordinarily stable even in the acid pH range (Jana et al., Citation2010). It is used as binder, gelling, thickening, emulsifying, and suspending agent in different pharmaceutical formulations and acts as stabilizer in food and pharmaceutical industries (Jani et al., Citation2009; Sahoo et al., Citation2010). TSP has been described as a viscosity enhancer showing mucomimetic, and mucoadhesive property (Kulkarni et al., Citation1997). Again, due to its hydrophilic and mucoadhesive property, it finds its use in the development of mucoadhesive drug delivery systems (Patel et al., Citation2009). In the present investigation, we evaluated the utility of TSP, as a natural mucoadhesive polymeric blend with sodium alginate for the preparation of gliclazide loaded TSP-alginate mucoadhesive microspheres by ionotropic gelation technique.
Gliclazide, 1- (3- azabicyclo- [3, 3, 0] - oct- 3- yl) - 3- (p- tolyl sulfonyl) urea, is one of the second generation sulfonylurea used as oral hypoglycemic agent in the treatment of non-insulin dependent diabetes mellitus (NIDDM) (CitationTripathi, 1999). Previous reports showed that gliclazide possesses good general tolerability, and lower rate of secondary failure (Mailhot, Citation1993; Palmer & Brogden, Citation1993). However, the gliclazide absorption rate from gastrointestinal tract is slow and varied among the subjects (Young et al., Citation2009). Slower absorption of gliclazide has been suggested to be due to either its poor dissolution owing to its hydrophobic nature or poor permeability across the gastrointestinal membrane (Al-Kassas et al., Citation2007). Therefore, incorporation of gliclazide in gastroretentive sustained release dosage forms such as mucoadhesive microspheres may control its absorption from gastrointestinal tract and overcome the variability problems. Literature survey reveals that a few investigations have been carried out to formulate alginate-based mucoadhesive microcapsules or beads for oral gliclazide delivery (Al-Kassas et al., Citation2007; Prajapati et al., Citation2008; Nayak et al., Citation2010c; Pal & Nayak, Citation2011). But, no attempt has been taken to formulate gliclazide loaded alginate-based mucoadhesive microsphere or bead systems using TSP as a natural mucoadhesive polymer.
The objectives of the present investigation were (i) to isolate TSP from the seeds of Tamarindus indica L. seeds, (ii) to formulate various TSP-alginate microspheres by ionotropic gelation technique, (iii) to characterize the drug delivery, and (iv) to evaluate gliclazide loaded TSP-alginate microspheres in vitro and in vivo.
Materials and methods
Materials
Gliclazide (Lupin Ltd., India), Sodium alginate (Central Drug House, India), and calcium chloride (Merck Ltd., India) were used for this present investigation. Tamarind seeds were purchased from local market. All other chemicals, and reagents used were of analytical grade.
Isolation of TSP
Raw seeds of tamarind (Tamarindus indica L.) were cleaned with distilled water to remove any extra pulp. Two hundred fifty grams of cleaned seeds were broken into small pieces and grounded into fine powder. Powders were taken in a 1000 ml beaker containing 500 ml water and boiled on water bath at 80–100°C with a constant stirring till a viscous solution was obtained. The solution was filtered using muslin cloth to throw away the undissolved fraction, and the supernatant was dried at 40°C for overnight. Then the dried material was called as tamarind kernel powder. TSP was prepared from this tamarind kernel powder according the method described by Rao et al (Rao et al., Citation1946). In brief, 20 gm of tamarind kernel powder was added in 200 ml of distilled water, and slurry was prepared. The slurry was poured into 800 ml of distilled water. The solution was boiled for 20 minutes under stirring condition in a water bath. The resulting clear solution was kept overnight to settle most of the proteins and fibers. The solution was then centrifuged at 5000 rpm for 20 minutes. The supernatant was separated and poured into twice the volume of absolute ethanol with continuous stirring. The precipitate was dried at 40°C. The dried film obtained was crushed to fine powder, passed through sieve no. 12 and kept in air tight desiccators.
Preparation of gliclazide loaded TSP-alginate microspheres
Gliclazide loaded TSP-alginate microspheres were prepared using calcium chloride (CaCl2) as cross-linking agent by ionotropic gelation technique. Briefly, sodium alginate and TSP aqueous dispersions were prepared separately using distilled water. These dispersions were well mixed with stirring for 10 minutes at 1000 rpm using a magnetic stirrer (Remi Motors, India). Afterwards, gliclazide was added to the dispersion mixture. The ratio of drug to polymer was maintained 1:1 in all formulations. The final TSP-alginate dispersion mixture containing gliclazide was homogenized for 10 minutes at 1000 rpm using a homogenizer (Remi Motors, India) and ultrasonicated for 5 minutes for debubbling. The resulting dispersion was then added via a 26 gauge needle. The added droplets were retained in the CaCl2 solution for 20 minutes to complete the curing reaction and to produce spherical rigid microspheres. The microspheres were collected by decantation, and washed three times with distilled water and dried at 45°C for 12 hours. The dried microspheres were sieved and stored in desiccators until used. The formulation and processing conditions of gliclazide loaded TSP-alginate microspheres are shown in .
Table 1. Formulation and processing conditions of gliclazide loaded TSP-alginate microspheres.
Estimation of gliclazide
Gliclazide was estimated by UV-VIS spectrophotometer (Shimadzu, Japan) based on the measurement of absorbance. Absorbance values were measured for different concentrations of gliclazide in phosphate buffer (pH 7.4) at the maximum wavelength (λmax = 226.5 nm). A maximum wavelength (λmax) was obtained by scanning all samples within the range of 200 to 400 nm. The method obeyed Beer’s law in the concentration range of 2 to 10 µg/ml. The method was validated for linearity, accuracy and precision. The regression equation for the calibration curve was denoted as
Determination of drug entrapment efficiency
Accurately weighed, 100 mg of gliclazide loaded TSP-alginate microspheres were taken and crushed in pestle and mortar. The crushed powders were placed in 500 ml of phosphate buffer (pH 7.4), and kept for 48 hours with occasionally shaking at 37 ± 0.5°C. The polymer debris formed after disintegration of microcapsules was removed filtering through Whatman® filter paper (no. 40). The drug content in the filtrate was determined by UV-VIS spectrophotometer (Shimadzu, Japan) at 226.5 nm. The drug entrapment efficiency of gliclazide loaded TSP-alginate microspheres was calculated using the following formula:
Particle size measurement
Particle size of 100 microspheres from each batch was measured by optical microscopic method using an optical microscope (Olympus) and average particle size was calculated. The ocular micrometer was previously calibrated by stage micrometer.
Morphology analysis
Gliclazide loaded TSP-alginate microspheres were gold coated by mounting on a brass stub using double-sided adhesive tape under vacuum in an ion sputter (Hitachi E1010, Japan) with a thin layer of gold (3–5 nm) for 75 sec and at 40 W to make them electrically conductive. Their morphology was examined by scanning electron microscopy (SEM) (Hitachi S3400, Japan).
Fourier transform infrared spectroscopy
Samples were reduced to powder and analyzed with KBr pellets by using a Fourier transform infrared (FTIR) spectroscope (Perkin Elmer Spectrum RX I). The pellet was placed in the sample holder and spectral scanning was taken in the wavelength region ranging between 4000 and 400 cm−1 at a resolution of 4 cm−1 with scan speed of 1 cm/sec.
In vitro drug release studies
The in vitro release of gliclazide from various gliclazide loaded TSP-alginate microspheres were tested using a dissolution rate test apparatus (paddle type) USP/BP/IP (Campbell Electronics, India) in acidic (0.1N HCl, pH 1.2) as well as alkaline (phosphate buffer, pH 7.4) media. The dissolution rates were measured at 37 ± 1°C under 50 rpm speed. Accurately weighed quantities of TSP-alginate microspheres containing gliclazide equivalent to 100 mg were added to 900 ml of dissolution mediums. 5 ml of aliquots were collected at regular time intervals and the same amount of fresh dissolution mediums were replaced into the dissolution vessels to maintain the sink conditions throughout the experiment. The collected aliquots were filtered, and diluted suitably to determine the absorbance at 226.5 nm using a UV-VIS spectrophotometer (Shimadzu, Japan). The in vitro drug release experiments were conducted in triplicate (n = 3).
Evaluation of swelling behavior
A 100 mg of gliclazide loaded TSP-alginate microspheres were soaked in phosphate buffer (pH 7.4) and 0.1N HCl (pH 1.2) to evaluate swelling behavior. The swelled microspheres were removed at predetermined time interval and weighed after drying the surface using tissue paper. Swelling index was determined by following formula:
Mucoadhesion testing by in vitro wash-off method
The mucoadhesivity of gliclazide loaded TSP-alginate microspheres were evaluated by in vitro wash-off method. Freshly excised pieces of goat intestinal mucosa (2 × 2 cm) (collected from slaughter house) were mounted on glass slide (7.5 × 2.5 cm) using thread. About 50 microspheres were spread onto the wet, ringed tissue specimen, and the prepared slide was hung onto a groove of disintegration test apparatus. The tissue specimen was given a regular up and down movement at 37 ± 0.5 °C in a vessel containing 900 ml of phosphate buffer (pH 7.4) and 0.1 N HCl (pH 1.2), separately. After regular time intervals, the machine was stopped and the number of microspheres still adhering to the tissue was counted.
In vivo studies
In vivo studies were performed in alloxan-induced diabetic albino rats (Prajapati et al., Citation2008; Venkidesh et al., Citation2010) of either sex (weighing 275–338 gm). The acclimatized rats were kept fasting for 24 hours with water ad libitum. All experiments were performed between 8 am to 12 pm to minimize circadian influences.
The animal experimental protocol was approved by the Institutional Animal Ethical Committee and was cleared before starting. The experimental design was subjected to the scrutiny of IFTM University Ethical Committee (reg. no. IFTM/837ac/0160). The animals were handled as per the guidance of the committee for the purpose of control and supervision on experimental animals (CPCSEA), New Delhi, India. All efforts were made to minimize both the suffering and number of animals used.
The rats were made diabetic by intraperitoneal administration of freshly prepared alloxan solution at a dose of 150 mg/kg dissolved in 2 mM citrate buffer (pH 3.0). After 1 week, alloxanized rats with fasting blood glucose of 300 mg/dl or more were considered diabetic and were employed in the study.
The alloxan-induced diabetic rats were divided randomly into 2 groups of 3 rats each and treated as follow: Group A was administered with pure gliclazide in suspension form, and Group B was administered with best formulation of gliclazide loaded TSP-alginate microspheres, both at a dose equivalent to 2 mg/kg of gliclazide by using oral feeding needle. Blood samples were withdrawn (0.1 ml) from tail tip of each rat at regular time intervals under mild ether anesthesia, and were analyzed for blood glucose by oxidase-peroxidase method using commercial glucose kit.
Statistical analysis
The data were analyzed with simple statistics, while in vivo blood glucose level data were analyzed for significant differences (p < 0.05) by paired samples t-test. The statistical analysis was conducted using MedCalc software version 9.6.4.0. All measured data are expressed as mean ± SD. Each measurement was done in triplicate (n = 3).
Results and discussion
Isolation of TSP and preparation of gliclazide loaded TSP-alginate microspheres
TSP was isolated from seeds of tamarind (Tamarindus indica L.), and the average yield of dried TSP was found to be 47%. Gliclazide loaded TSP-alginate microspheres were prepared by varying two different ratio of isolated TSP, and sodium alginate blend (1:1, and 1:2) with different concentrations of CaCl2 solution (2–10% w/v) as cross-linking solution by ionotropic gelation technique (). Rigid TSP-alginate microspheres containing gliclazide were obtained, when dispersion mixture of sodium alginate, TSP, and gliclazide was dropped in the solutions containing calcium ions.
Drug entrapment efficiency
The drug entrapment efficiencies in gliclazide loaded TSP-alginate microspheres were within the range between 58.12 ± 2.42 (F1) and 82.78 ± 3.43 (F8) % w/w (). The highest drug entrapment efficiency in gliclazide loaded TSP-alginate microspheres was evidenced in case formulation F8 (82.78 ± 3.43% w/w), where TSP to sodium alginate blend ratio was 1:2 and the concentration of cross linker, CaCl2 was 10% w/v. The drug entrapment efficiencies were increased with decreasing TSP to sodium alginate blend ratios and increasing cross-linking concentrations. This may be due to the high degree of cross-linking as the amount of sodium alginate in the polymer blend, and concentration of cross linker (i.e. CaCl2) in cross-linking solution were increased. When sodium alginate containing polymer blend (TSP and sodium alginate) was placed into a solution of CaCl2, the calcium ions were found to replace the sodium ions of sodium alginate to form calcium alginate, which provided cross-linking tendency to form the cross-liked microspheres. Again, at lower concentration of CaCl2, the microspheres might have larger pores due to insufficient cross-linking that resulted in lower drug entrapment (Sharma & Bhattacharya, Citation2008).
Particle size and morphological characteristics
Particle size of gliclazide loaded various TSP-alginate microspheres for each formulation was measured by optical microscopic method. The particle size of these microspheres was within the range of 752.12 ± 6.42 to 948.49 ± 20.92 µm (). Elevations of the particle size of these microspheres were found with the increasing incorporation of TSP into formulations. This could be attributed due to the increase in viscosity of polymer blend solution with incorporation of TSP in increasing ratio that in turn increased the droplet size during addition of the polymer blend solution to the cross-linking solution. Again, the decrease in particle size of these formulated TSP-alginate microspheres was observed when more concentrated CaCl2 solution was used as cross-linking solution. This may due to shrinkage of polymeric gel by higher degree of cross-linking with the high concentration of cross linker (i.e. CaCl2).
It was also evidenced that the drug entrapment efficiencies of gliclazide loaded TSP-alginate microspheres were related with particle sizes. The drug entrapment efficiencies were appeared to decrease with increasing particle size. The morphological analysis of microspheres was done by SEM and presented in . The SEM photograph indicated that microspheres were spherical particles of rough surfaces with no tendency to aggregate.
FTIR spectroscopic analysis
FTIR spectroscopic analysis was performed to confirm the compatibility of gliclazide with polymers used to prepare gliclazide loaded TSP-alginate microspheres. The FTIR spectra of sodium alginate, TSP, TSP-alginate blank microspheres, gliclazide loaded TSP-alginate microspheres, and pure gliclazide were shown in . In the FTIR spectrum of sodium alginate, the characteristic peaks were appeared at 1420.70 cm−1, 1620.25 cm−1 and 3442.50 cm−1, for symmetric -COO− stretching vibration, asymmetric -COO− stretching vibration and for the -OH groups, respectively. In the FTIR spectrum of TSP, the characteristic peaks appeared at 1043.06 cm−1, 1650.37 cm−1, 2925.55 cm−1, and 3403.48 cm−1 suggested -HC = O stretching vibration, -CH-OH stretching vibration, aliphatic C-H stretching, and OH groups, respectively. On the other hand, the FTIR peaks of gliclazide was observed at 1351.54 cm−1, 1598.00 cm−1, 1702.50 cm−1, 3115.28 cm−1, and 3275.65 cm−1 for SO2–NH group, aromatic C=C, -HC=O stretching vibration, =C-H stretching vibration, and –NH stretching vibration, respectively. In the FTIR spectrum of gliclazide loaded TSP-alginate microspheres, various characteristic peaks of sodium alginate, TSP, and gliclazide were appeared without any significant shifting of these peaks. In short, the gliclazide loaded microparticles prepared with TSP-sodium alginate polymeric lend had significant characters of gliclazide in the FTIR spectrum, suggesting, there were no reactions between the gliclazide and the polymers (TSP, and sodium alginate) used.
In vitro drug release
Gliclazide release from various TSP-alginate microspheres was studied in gastric pH (0.1 N HCl, pH 1.2), and intestinal pH (phosphate buffer, pH 7.4). Various gliclazide loaded TSP-alginate microspheres showed prolonged release of gliclazide over 12 hours. The gliclazide release from TSP-alginate microspheres was slow and dependent on both the composition of the matrix (alginate and TSP) and the degree of cross-linking by Ca2+ ions. The release of gliclazide from TSP-alginate microspheres was observed 10.02 ± 0.08% (F 4) to 17.26 ± 0.16% (F 5) in 0.1 N HCl, pH 1.2 after 12 hours (); whereas, this was 75.98 ± 0.56% (F 4) to 95.16 ± 1.21% (F 5) in phosphate buffer, pH 7.4 after 12 hours (). The release of gliclazide from TSP-alginate microspheres at gastric pH (pH 1.2) was comparatively slow and sustained than intestinal pH (pH 7.4). This was due to the shrinkage of alginate at acidic pH (as alginate is pH sensitive), which might slower the drug release from the gliclazide from TSP-alginate microspheres. The reason of the higher drug release in phosphate buffer, pH 7.4 was due to the higher swelling rate of these microspheres. In case of comparatively higher TSP containing microspheres, the more hydrophilic property of the TSP binded better with water to form viscous gel structure, which might blockade the pores on the surface of microspheres and sustain the release profile of the drug, gliclazide. Again, the release of gliclazide from TSP-alginate microspheres formulated with higher degree of cross-linking was comparatively sustained than the microspheres formulated with lower degree of cross-linking. The higher concentration of cross-linking agent (i.e. CaCl2) could produce high degree of cross-linking and thereby lowered the drug release from highly cross-linked TSP-alginate microspheres.
Swelling behavior
Swelling behavior of gliclazide loaded TSP-alginate microspheres was evaluated in gastric pH (0.1 N HCl, pH 1.2), and intestinal pH (phosphate buffer, pH 7.4). The swelling index profile of these microspheres in both the pH were shown in , and . The swelling index of gliclazide loaded TSP-alginate microspheres was lower in 0.1 N HCl, pH 1.2 in comparison with swelling index in phosphate buffer, pH 7.4. This occurred due to shrinkage of alginate at acidic pH. Maximum swelling of these microspheres was noticed at 2–3 hours in phosphate buffer after which, erosion and dissolution took place. It has been previously reported that the swelling of calcium alginate was dependant on the progressive displacement of calcium ions from calcium alginate (Del Gaudio et al., Citation2005). It was also reported that the swelling might be enhanced by the presence of phosphate ions (in phosphate buffer), which acted as calcium sequestrant (Ostberg & Graffner, Citation1994). Therefore, the swelling behavior of gliclazide loaded TSP-alginate microspheres in phosphate buffer, pH 7.4 could be explained by the phenomenon of ion exchange between calcium ion of the cross-liked TSP-alginate microspheres and the sodium ions present in phosphate buffer, with the influence of calcium sequestrant phosphate ions, which resulted in disaggregation in the TSP-alginate matrix structure leading to matrix erosion and dissolution of the swollen microspheres. These results clearly suggested that these gliclazide loaded TSP-alginate microspheres might swell slightly in the stomach as they subsequently moved to the upper intestine, where the gliclazide was to be absorbed, and these microspheres began to swell more and released the loaded gliclazide.
Mucoadhesion
The in vitro wash-off test for assessing mucoadhesivity of these TSP-alginate microspheres containing gliclazide was performed at both gastric pH (0.1N HCl, pH 1.2) and intestinal pH (phosphate buffer, pH 7.4) for 10 hours. The wash off was faster at intestinal pH than at gastric pH. In 0.1N HCl, the percentage of microspheres adhering to the goat intestinal mucosal tissue varied from 56.50 ± 4.25 to 70.85 ± 5.25% (), whereas, this varied from 38.55 ± 0.58 to 48.60 ± 3.25% in phosphate buffer (). The decreased mucoadhesion of microspheres in phosphate buffer might be resulted due to the erosion of calcium ion (Nayak et al., Citation2010c). The results of the wash-off test indicated that the gliclazide loaded TSP-alginate microspheres had fairly good mucoadhesive properties.
In vivo studies
In vivo studies in alloxan-induced diabetic albino rats were performed with gliclazide loaded TSP-alginate mucoadhesive microspheres of formulation F4, which was selected as best formulation based on swelling behavior, mucoadhesivity, and in vitro drug release studies. Pure gliclazide was administered orally in suspension form at a dose equivalent to 2 mg/kg body weight. When pure gliclazide solution was administered, a rapid reduction in blood glucose level was observed up to 2 hours. After that, the blood glucose level recovered rapidly towards the normal level (, and ). There were significant differences (p < 0.05) between the blood glucose level after administration of pure gliclazide and gliclazide loaded TSP-alginate mucoadhesive microspheres (F4). But, it was found that the reduction of blood glucose levels in the groups treated with gliclazide loaded TSP-alginate mucoadhesive microspheres of formulation F4 (Group B) was slower than that of the group treated with pure gliclazide (Group A). In the case of groups treated with TSP-alginate mucoadhesive microspheres containing gliclazide (Group B), the maximum reduction in blood glucose levels was found at 4 hours after oral administration and the reduction in glucose level were sustained over 12 hours. A 25% reduction in glucose level was considered as significant hypoglycemic effect (Chowdary & Rao, Citation2003; Prajapati et al., Citation2008; Venkidesh et al., Citation2010). Therefore, the sustained hypoglycemic effect by the TSP-alginate mucoadhesive microspheres containing gliclazide (F4) was observed over a longer period of time. The above studies suggested that the TSP-alginate mucoadhesive microspheres containing gliclazide containing gliclazide swelled slowly in the stomach and accordingly adhered to the stomach mucosa allowing more gliclazide to be absorbed minimizing the diffusion barriers to increase the absorption period by prolonging the gastric residence time of the drug. Then the microspheres were subsequently moved to the upper part of the intestine, where they swelled more and released drug through the polymeric gel layer, which was formed at matrices periphery. The results of this study clearly demonstrated the ability of the gliclazide TSP-alginate mucoadhesive microspheres to enhance, prolong and control the gastrointestinal absorption of gliclazide.
Figure 9. Comparative in vivo blood glucose level in alloxan-induced diabetic rats after oral administration of pure gliclazide and gliclazide loaded TSP-alginate mucoadhesive microspheres (F4) (mean ± SD, = 3). *Significant difference between blood glucose level after oral administration of pure gliclazide and gliclazide loaded TSP-alginate mucoadhesive microspheres (F4). The data were analyzed for significant differences (p < 0.05) by paired samples -test. The statistical analysis was conducted using MedCalc software version 9.6.4.0.
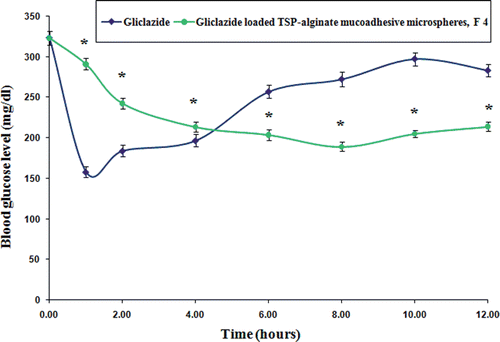
Conclusion
The gliclazide loaded new TSP-alginate mucoadhesive microspheres by ionotropic gelation technique was developed and evaluated. The drug entrapment efficiencies of these microspheres were within the range between 58.12 ± 2.42 to 82.78 ± 3.43% w/w. The prepared microspheres were of spherical shape with rough surfaces, and their average particle size varied within the range of 752.12 ± 6.42 to 948.49 ± 20.92 µm. FTIR analysis suggested that there were no reactions between the gliclazide and the polymers (TSP, and sodium alginate) used. All these TSP-alginate microspheres exhibited good mucoadhesivity. The in vivo study revealed that selected best formulation of TSP-alginate mucoadhesive microspheres containing gliclazide produced the significant hypoglycemic effect on oral administration. The preparation method for TSP-alginate mucoadhesive microspheres for oral gliclazide delivery was found to be very simple and reproducible. Finally, it can be concluded that these gliclazide loaded new TSP-alginate mucoadhesive microspheres are very much suitable for prolonged systemic administration of gliclazide through sustained drug release and mucoadhesive properties after oral administration in the management of NIDDM with maintenance of blood glucose level and reduction of patient to patient variability.
Acknowledgments
One of the authors is thankful to Dr R Dubey, Vice Chancellor, IFTM University, Lodhipur, Moradabad, UP, India, for providing necessary facilities.
Declaration of interest
The authors report no conflicts of interest. The authors alone are responsible for the content and utility of the paper.
References
- Al-Kassas RS, Al-Gohary OM, Al-Faadhel MM. Controlling of systemic absorption of gliclazide through incorporation into alginate beads. Int J Pharm 2007;341:230–237.
- Andrews GP, Laverty TP, Jones DS. Mucoadhesive polymeric platforms for controlled drug delivery. Eur J Pharm Biopharm 2009;71:505–518.
- Basu SK, Adhiyaman R. Preparation and characterization of nitrendipine-loaded Eudragit RL 100 microspheres by an emulsion-solvent evaporation method. Trop J Pharm Res 2008;7:1033–1041.
- Cevher E, Taha MA, Orlu M, Araman A. Evaluation of mechanical and mucoadhesive properties of clomiphene citrate gel formulations containing carbomers and their thiolated derivatives. Drug Deliv 2008;15:57–67.
- Chowdary KR, Rao YS. Design and in vitro and in vivo evaluation of mucoadhesive microcapsules of glipizide for oral controlled release: a technical note. AAPS PharmSciTech 2003;4:E39.
- Chowdary KR, Rao YS. Mucoadhesive microspheres for controlled drug delivery. Biol Pharm Bull 2004;27:1717–1724.
- Chowdary KPR, Srinivas Rao S. Mucoadhesive microspheres and microcapsules: current status. Indian J Pharm Sci 2005;67:141–150.
- Comoglu T, Gomul N. Development and in vitro evaluation of pantoprazole–loaded microspheres. Drug Deliv 2008;15:295–302.
- Davis TA, Llanes F, Volesky B, Diaz-Pulido G, McCook L, Mucci A. 1H-NMR study of Na alginates extracted from Sargassum spp. in relation to metal biosorption. Appl Biochem Biotechnol 2003;110:75–90.
- El-Kamel AH, Al-Gohary OM, Hosny EA. Alginate-diltiazem hydrochloride beads: optimization of formulation factors, in vitro and in vivo availability. J Microencapsul 2003;20:211–225.
- George M, Abraham TE. Polyionic hydrocolloids for the intestinal delivery of protein drugs: alginate and chitosan–a review. J Control Release 2006;114:1–14.
- Del Gaudio P, Colombo P, Colombo G, Russo P, Sonvico F. Mechanisms of formation and disintegration of alginate beads obtained by prilling. Int J Pharm 2005;302:1–9.
- Jain SK, Chourasia MK, Jain AK, Jain RK, Shrivastava AK. Development and characterization of mucoadhesive microspheres bearing salbutamol for nasal delivery. Drug Deliv 2004;11:113–122.
- Jana S, Lakshman D, Sen KK, Basu SK. Development and evaluation of epichlorohydrin cross-linked mucoadhesive patches of tamarind seed polysaccharide for buccal application. Int J Pharm Sci Drug Res 2010;2:193–198.
- Jani GK, Shah DP, Prajapati VD, Jain VC. Gums and mucilages: versatile excipients for pharmaceutical formulations. Asian J Pharm Sci 2009;4:309–323.
- Kroll E, Winnik FM, Ziolo RF. In situ preparation of nanocrystalline γ-Fe2O3 in iron (II) cross-linked alginate gels. Chem Mater 1996;8:1594–1596.
- Kulkarni D, Dwivedi AK, Sarin JPS, Singh S. Tamarind seed polyose: a potential polysaccharide for sustained release of verapamil hydrochloride as a model drug. Indian J Pharm Sci 1997;59:1–7.
- Llanes F, Ryan DH, Marchessault RH. Magnetic nanostructured composites using alginates of different M/G ratios as polymeric matrix. Int J Biol Macromol 2000;27:35–40.
- Mailhot J. Efficacy and safety of gliclazide in the treatment of non-insulin-dependent diabetes mellitus: a Canadian multicenter study. Clin Ther 1993;15:1060–1068.
- Nayak AK, Maji R, Das B. Gastroretentive drug delivery systems: a review. Asian J Pharm Clin Res 2010a;3:2–10.
- Nayak AK, Malakar J, Sen KK. Gastroretentive drug delivery technologies: current approaches and future potential. J Pharm Educ Res 2010b;1:1–12.
- Nayak AK, Hasnain MS, Beg S, Alam MI. Mucoadhesive beads of gliclazide: design, development, and evaluation. Sci Asia 2010c;36:319–325.
- Nerkar PP, Gattani S. In vivo, in vitro evaluation of linseed mucilage based buccal mucoadhesive microspheres of venlafaxine. Drug Deliv 2011;18:111–121.
- Ostberg T, Graffner C. Calcium alginate matrices or oral multiple unit administration: II Influence of calcium concentration, amount of drug added and alginate characteristics on drug release. Int J Pharm 1994;111:271–282.
- Pal D, Nayak AK. Development, optimization, and anti-diabetic activity of gliclazide-loaded alginate-methyl cellulose mucoadhesive microcapsules. AAPS PharmSciTech 2011;12:1431–1441.
- Palmer KJ, Brogden RN. Gliclazide. An update of its pharmacological properties and therapeutic efficacy in non-insulin-dependent diabetes mellitus. Drugs 1993;46:92–125.
- Patel B, Patel P, Bhosale A, Hardikar S, Mutha S, Chaulang G. Evaluation of tamarind seed polysaccharide (TSP) as a mucoadhesive and sustained release component of nifedipine buccoadhesive tablet & comparison with HPMC and Na CMC. Int J PharmTech Res 2009;1:404–410.
- Prajapati SK, Tripathi P, Ubaidulla U, Anand V. Design and development of gliclazide mucoadhesive microcapsules: in vitro and in vivo evaluation. AAPS PharmSciTech 2008;9:224–230.
- Rao PS, Ghosh TP, Krishna S. Extraction and purification of tamarind seed polysaccharide. J Sci Ind Res 1946;4:705.
- Sachan NK, Bhattacharya A. Modeling and characterization of drug release from glutinous rice starch based hydrogel beads for controlled drug delivery. Int J Health Res 2009;2:93–99.
- Sahoo R, Sahoo S, Nayak PL. Release behavior of anticancer drug paclitaxel from tamarind seed polysaccharide galactoxyloglucan. Eur J Sci Res 2010;47:197–206.
- Satturwar MP, Fulzele VS, Dorle AK. Biodegradation and in vivo biocompatibility of rosin: a natural film-forming polymers. AAPS PharmSci Tech 2003;4:434–439.
- Sharma VK, Bhattacharya A. Release of metformin hydrochloride from ispaghula-sodium alginate beads adhered on cock intestinal mucosa. Indian J Pharm Educ Res 2008;42:365–372.
- Shilpa A, Agarwal SS, Rao AR. Controlled delivery of drug from alginate matrix. J Macromol Sci –Polym Rev 2003;43:187–221.
- Smidsrod O, Draget KI. Chemistry and physical properties of alginates. Carbohydr Eur 1996;14:6–13.
- Tripathi KD. Essential of Medical Pharmacology. 4th edition. New Delhi: Jaypee Brothers, Medical Publishers.
- Vasir JK, Tambwekar K, Garg S. Bioadhesive microspheres as a controlled drug delivery system. Int J Pharm 2003;255:13–32.
- Venkidesh R, Pal DK, Mohana LS, Saravanakumar A, Mandal SC. Antidiabetic activity of Smilax chinensis L. extract in streptozotacin-induced diabetic rats. Int J Phytopharm 2010;1:16–21.
- Young JF, Wei GL, Lu R, Liu CX, Zheng BZ, Feng P. Bioavailability of gliclazide sustained release tablet in healthy volunteers. Asian J Pharmacodyn Pharmacokin 2009;2:150–160.