Abstract
Injectable implant would be a potential way for 2-methoxyestradiol (2-ME) for the therapy of breast cancer because of its water-insolubility, short half-life, and low oral bioavailability. So 2-ME microspheres based on poly (DL-lactide-co-glycolide) were prepared by emulsion solvent extraction method and characterized for morphology, particle size, drug physical state, entrapment efficiency and drug release in vitro and in vivo. Their cytotoxicity on MCF-7 cells was evaluated by sulforhodamine B (SRB) method. The 2-ME was successfully entrapped in the interior of microspheres with particle size of 55.44 ± 12.21 μm and could exist in an amorphism. In vitro and in vivo release of 2-ME from the microspheres occurred in a Ritger–Peppas and zero-order manner with a slow release over 46 days, respectively, and their better correlation was found. The 2-ME even with very low concentration in the microspheres could efficiently inhibit the growth of MCF-7 cells compared to the equivalent amount of drug in free solution, which indicated that the release rate from the microspheres and local water-solubility of 2-ME could maintain effective drug concentration in target site. The above results indicated that the microspheres prepared could not only control prolonged release of 2-ME in vitro and in vivo but also maintain effective drug concentration in target site. So 2-ME microspheres are acceptable for controlled release devices for effective treatment of breast cancer.
Introduction
The 2-methoxyestradiol (2-ME), formerly regarded as an inactive metabolite of 17β-estradiol, is now known to be an antitumor agent in some animal models of solid tumor, by its antiangiogenic effect and antiproliferative effect (CitationSutherland et al., 2007; CitationWang et al., 2011). The 2-ME is currently being evaluated in Phase II clinical trials for the treatment of solid tumors, such as breast cancer (Dahut et al., Citation2006; CitationJehana et al., 2006). However, the oral therapeutic outcome by 2-ME maintained unsatisfactory results because of its water-insolubility, short half-life, extensively distribution, and low oral bioavailability. Despite improved bioavailability through reformulation in a nanocrystal colloidal dispersion (NCD) (http://www.entremed.com), the short half-life of 2-ME, determined by susceptibility to metabolic degradation and conjugation, remains a major influence on Cmax and AUC. The problems associated with currently available oral therapies with 2-ME are that they offer rapid removal from plasma and unmaintained effective plasma level.
Localized delivery by subcutaneous injective microspheres would be a potential way for 2-ME, which could not only control drug release and maintain drug concentration in the tumor site at cytotoxic levels but also overcome the drawbacks of systemic administration, such as short half-life, metabolic deactivation, and extensively distribution associated with oral administration (CitationBodmer et al., 1992; Freiberg and Zhu, Citation2004). Poly (DL-lactide-co-glycolide) (PLGA) is the most commonly used biodegradable copolymer. With the long history of safe human use in the form of surgical sutures, PLGA has been studied extensively as the injectable polymeric microspheres to prolong the release of therapeutic agents, such as antibiotics, anti-inflammatory drugs, anticancer drugs, and proteins (CitationBodmer et al., 1992). Some of the products are commercially available, for example, Lupron Depot®, with a controlled release of leuprolide acetate for 1 month (Heijckmann et al., Citation2001; CitationOh and Pisegna, 2001; CitationMitwally et al., 2005) after subcutaneous administration.
The objective of this study was to develop 2-ME loaded PLGA microspheres, a newly controlled release system and to determine the physicochemical characteristics of the developed microspheres, such as morphology, particle size, drug physical state, entrapment efficiency (EE), and drug release in vitro and in vivo. In addition, cytotoxic effects of these microspheres in breast cancer MCF-7 cells were investigated.
Materials and methods
Materials
The 2-ME (99.5% in purity) was home-made. The PLGA 75/25 (Mw 38500, inherent viscosity 0.35dL/g) was purchased from Daigang (JiNan, China). Polyvinyl alcohol (PVA) (Mw 1788 ± 50, 88% hydrolyzed) was supplied by Chengdu Kelong Chemical Co., Ltd (China). Dialysis bags were provided from Sigma (molecular weight cutoff, MWCO, 8000–14000, USA). Methylene chloride (DCM) was purchased from Tianjinsiyou Chemical Co. All reagents for high-performance liquid chromatography (HPLC) analysis, including methanol and acetonitrile were of HPLC grade. Other chemicals used were of analytical grade.
Preparation of microspheres
Microspheres were prepared by solvent extraction technique (Jain, Citation2000). Briefly, 17.1 mg 2-ME was dissolved in 1 mL methylene chloride (DCM) containing 125 mg PLGA by ultrasound (KQ-500DE, Kunshan, China) at 25°C and 250 W for 20 min. The resulting organic phase was slowly added to a 30 mL PVA (1.0%) aqueous solution and then the mixture was oscillated at 2250 rpm for 10 min. The obtained emulsion was then added to 80 mL PVA (1.0%) aqueous solution and stirred at 300 rpm for 4 h at room temperature to evaporate DCM. The microspheres prepared were collected by centrifugation at 3000 rpm for 5 min and washed three times with distilled water. The microspheres were then lyophilized for 24 h and stored −20°C before further analysis. Blank microspheres were prepared by the above same method without adding 2-ME.
Characters of microspheres
The particle size distribution of the microspheres prepared was measured using Nikonicroscopy with a digital camera attached and the SPSS software (Jackson et al., Citation2007). The microspheres lyophilized were suspended in 0.05% Tween80 aqueous solution and vortexed before measurement. The morphology of the microspheres was observed by fluorescent microscopy (×200) (ECLIPSE 80i, Nikon).
Differential scanning calorimetry (DSC) analysis was performed to characterize the physical state of the drug in microspheres lyophilized. The samples were weighed into an aluminum pan, which was then sealed with a pinhole-pierced cover. Heating curves from 10°C to 200°C were recorded with a scan rate of 5°C/min in a DSC (Daojin DSC-60A, Japan) equipped with a cooling unit. An empty pan was used as a reference.
The EE and drug loading (DL) were determined by HPLC method on Agilent 1200 series system with fluorescence detector (Agilent, USA). The chromatographic conditions were as follows: C18 column (150 mm × 4.6mm, 5 μm) (Agilent, USA), a guard (C18, 10 mm × 4.6mm, 5 μm) installed ahead of the analytical column, column temperature 30°C, injection volume 20 μL, mobile phase consisting of methanol and water (65:35, v/v) was at a flow rate of 1.0 mL/min, the excited wavelength and emission wavelength were 285 nm and 325 nm, respectively. The calibration curve for the quantification for 2-ME was linear over the range of standard concentration between 25 and 1250 ng/mL with a correlation coefficient of R2 = 0.9996. About 10 mg of the freeze-dried microspheres were dissolved in 0.5 mL of DCM. The resulting solution was then diluted to 3 mL with methanol and vortex-mixed for 10 min. The dispersion was centrifuged for 10 min at 10,000 rpm. The supernatant was injected into HPLC system to determine the concentration of 2-ME. The DL and EE were calculated as follows: DL% = (drug amount found in microspheres/total amount of microspheres) × 100, EE% = (drug amount found in microspheres/drug amount added) × 100. All the measurements were conducted in triplicate and the mean values and standard deviations were reported.
In vitro drug release studies
In vitro release of 2-ME from microspheres was performed using the dialysis bag diffusion technique. The dialysis bags (MWCO, 8000–14000, Sigma) were soaked in deionized water for 12 h before use. About 10 mg of 2-ME microspheres lyophilized were rehydrated in 1 mL of PBS (PH 7.4) and were placed in dialysis bags with two ends fixed by thread (the distance between two nodes was 3 cm). The bag was put into the flask containing 20 mL of 0.1 M PBS (pH 7.4) containing 1% Tween 80 and 0.02% sodium azide and then shaken horizontally (ZD-85, Zhejiangjintan, China) at 37°C and 50 strokes/min. Aliquots of the dissolution medium (1 mL) were withdrawn at appropriate intervals, and the same volume of fresh dissolution medium was added to the flask to maintain the constant volume. Then the sample was centrifuged at 10,000 rpm for 10 min. The amount of 2-ME in the collected supernatant was measured by the HPLC method (see “Characters of microspheres”).
In vivo drug release studies
Kunming strain female mice (30 ± 2 g) were treated by subcutaneous injection of the suspension containing 10 mg of drug-loaded microspheres and 0.4 mL of dispersed solution (containing 0.5% CMC-Na and 0.05% Tween-80). All suspension used was vortex-mixed before administration. After administration, all the centrifuge tubes were collected to determine the residue drug and calculate the actual amount of injected drug. At designed time point, mice were sacrificed. The skin was cut and the residual microspheres was taken out carefully and placed in tubes. The drug amount in the residual microspheres was determined by HPLC (see “Characters of microspheres”) after the microspheres were dissolved by dichloromethane.
Cytotoxicity
In a 96-well plate, MCF-7 cells were seeded at a density of 7000–8000 cells/well in 0.2 mL of RPMI1640 with 10% fetal bovine serum (FBS) and antibiotics, and then cultured at 37°C for 24 h. After culture, the growth medium was removed and growth medium containing 2-ME dimethyl sulfoxide (DMSO) solution, blank microspheres, and 2-ME loaded microspheres with different concentration was added, respectively (Kimiko et al., 2004). The cells were further incubated for 24 h, 48 h, and 72 h, respectively. After incubation, cells were fixed by adding 20 μL cold 50% trichloroacetic acid (TCA, w/v, 4°C) and incubated at 4°C for 1 h. After the plates were washed with tap water and dried in air, an aliquot of 50 μL 4% (w/v) sulforhodamine B (SRB) in 1% acetic acid (v/v) was added and stained for 30 min. Excess SRB was removed by washing with 1% acetic acid (v/v) for at least three times, and then a volume of 150 μL Tris base (10 mM) was added to each well and shaken for 15–30 min. The optical density (OD) was read (Spectra MR, DYNEX, USA) at 490 nm. Finally, the values of IC50 were determined from concentration-dependent cell viability curves.
Results
Characteristics of PLGA microspheres
The morphology of 2-ME loaded microspheres observed visually by fluorescent microscopy was shown in . From , spherical microspheres with some pores were observed. While blank microspheres have relatively smooth surface, 2-ME microspheres have a concave appearance, which could be due to surface deposition of the drug in the solidification process of the organic droplets (Liggins and Burt, Citation2004). Generally, the injected microspheres should easily go through the syringe. So the particle size should be less than 250 μm, and it will be preferable if it is less than 125 μm. The microspheres with the particle size of 55.44 ± 12.21 μm were prepared, which provided guarantee with better syringeability for subcutaneous injection. The EE and the DL determined from three batches was estimated to be 81.70 ± 1.60% and 11.66 ± 0.449%, respectively, with satisfactory reproducibility. The unencapsulated drugs were washed off in the preparation.
Figure 1. (A) The morphology of blank microspheres and (B) 2-ME loaded microspheres prepared by PLGA with Mw 38500 by fluorescent microscope (×200) (ECLIPSE 80i, Nikon).
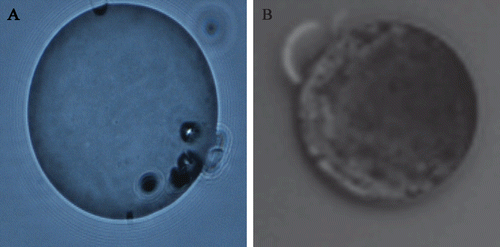
The DSC was used to investigate the existing form of 2-ME in microspheres. In the present work, the DSC thermograms of 0.8 mg pure 2-ME, physical mixture of 6.06 mg pure PLGA, and 0.8 mg 2-ME, 6.86 mg blank microspheres, and 6.86 mg 2-ME-loaded microparticles containing 0.8 mg 2-ME were detected and shown in . The melting endothermic peak of PLGA was observed at 52.53°C in physical mixture, which could be observed for blank and drug-loaded microspheres. The melting endothermic peak of 2-ME was observed at 189.46°C, which could be observed for the physical mixture. However, there was no peak observed at the temperature range of 100°C–250°C for 2-ME loaded microspheres. The DSC study did not detect any crystalline drug material in 2-ME-loaded microparticles. It could thus be concluded that the 2-ME formulated in the microspheres was in an amorphous or disordered-crystalline phase of a molecular dispersion or a solid solution state in the polymer matrix after fabrication. This suggested that 2-ME was successfully entrapped in microspheres.
Drug release in vitro and in vivo and their relationship evaluation
showed the in vitro release profiles of 2-ME from the microspheres at the previously defined time. The microspheres could control prolonged release of 2-ME over 2 months without significant burst release. Experimental in vitro drug release data were fitted with Ritger–Peppas model (lnQ = 0.7692 lnt-3.2422, r = 0.9962) to establish that the release rate and pattern of drug from PLGA matrix was mainly dependent not only on diffusion of drug through the matrix but also on the degradation of PLGA (CitationSchwendeman et al., 1996; Berkland et al., 2008).
Figure 3. The 2-ME release obtained from PLGA microspheres in vitro at 37°C in PBS buffer (pH7.4) the dialysis bag diffusion technique (mean ± SD; n = 3) (A), in vivo in Kunming strain female mice (mean ± SD; n = 3) (B) and their correlation (C).
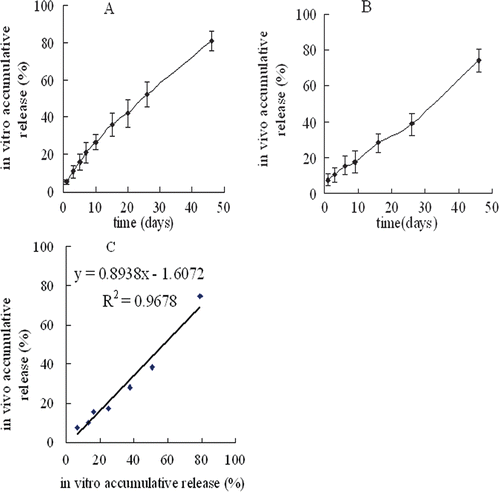
The in vivo release profiles () of 2-ME from the microspheres occurred in a zero-order manner with a slow release over 46 days (Q = 1.4438t + 5.4274, r = 0.9953), indicating that the microspheres could control in vivo slow and steady release of 2-ME.
As shown in , a linear relationship between in vitro and in vivo release data was found by plotting the in vitro release data on the x-axis and the in vivo release data on the y-axis, while time points (z-axis, not shown) were on the same scale was established.
Cytotoxicity
Based on the above release results in vitro and in vivo, about 10% 2-ME could be released from the microspheres in 72 h. So 2-ME concentration in the microspheres 10 times higher than in the solution was designed and then the amount of 2-ME released from the microspheres in 72 h was equivalent to its amount in the solution.
The effect of DMSO and blank microspheres on the growth of MCF-7 had been investigated. The OD value of blank growth medium, DMSO (1 μL/mL) and blank microspheres (103.67 μg, the maximal amount used) were 1.988 ± 0.021, 2.075 ± 0.018, and 2.109 ± 0.019, respectively, indicating that the effect of DMSO and blank microspheres on cells was very weak and neglectable.
From and , IC50 value significantly decreased with incubation time, which indicated that the growth inhibition of MCF-7 cells showed significant time-dependence after exposure to 2-ME both in solution and in microspheres. The growth inhibition of MCF-7 cells showed significant dose-dependence when 2-ME concentration in solution was lower than 5.0 μM and then achieved saturation. However, the growth inhibition of MCF-7 cells after exposure to 2-ME in microspheres showed no significant dose-dependence, and 2-ME even with very lower concentration in the microspheres could efficiently inhibit the growth inhibition of MCF-7 cells compared to the equivalent amount of drug in free solution from the and .
Table 1. Cytotoxicities of 2-ME solution and 2-ME microspheres against MCF-7cells after different incubation time (n = 3, mean ± SD).
Discussions
In this paper, an injectable implant, 2-ME microspheres have been successfully prepared by emulsion solvent extraction method. Recently, CitationKashappa et al. (2008) had reported injectable cylindrical PLGA implants on the millimeter scale (0.8 mm diameter) for 2-ME and only investigated the 2-ME release in vitro. In this paper, in vivo release of 2-ME from the microspheres occurred in a zero-order manner and the microspheres could control in vivo slow and steady release of 2-ME over 46 days without burst release or lag phase (Jeong et al., Citation2003; Sun et al., Citation2008), which provided important guarantee for steady therapeutic effect and low side effect. In addition, better correlation between in vitro and in vivo drug release from the microspheres was achieved, which indicated that the experimental method of in vitro drug release was reasonable and drug release in vivo could be inferred by drug release in vitro. However, different environments in vivo and in vitro resulted in different drug release model from the microspheres (Banu et al., 2008).
Local subcutaneous injection of 2-ME microspheres could not only overcome the above mentioned shortcomings of systemic administration but also really maintain effective drug concentration in local/target site. The latter could be confirmed by the cytotoxicity experiments of 2-ME microspheres on the MCF-7 cells. The 2-ME even with very low concentration in microspheres could effectively inhibited growth of MCF-7 cells compared to the equivalent amount of drug in free solution, which indicated that 2-ME release rate from the microspheres and its local solubility could meet requirement for efficient therapy of breast cancer and 2-ME microspheres was a suitable and potential preparation for local therapy of breast cancer.
Conclusions
The 2-ME microspheres were successfully prepared by emulsion solvent extraction method. The 2-ME entrapped in the microparticles existed in a new phase in the polymer matrix. The microspheres could control slow and steady release of 2-ME over 46 days in vitro and in vivo and they revealed better correlation. The 2-ME release rate from the microspheres and its local water-solubility could meet the requirements for effective therapy of breast cancer. So 2-ME microspheres were a potential and effective preparation for local therapy of breast cancer.
Acknowledgment
The authors thank Dr. XiufangShi (Pharmaceutical Chemistry Department, Zhengzhou University) providing the material of 2-ME.
Declaration of interest
All authors explicitly state that they all have no declarations of interest to report.
References
- Banu SZ, Diane JB. (2008). Evaluation of in vivo–in vitro release of dexamethasone from PLGA microspheres. J Control Rel 127: 137–145.
- Berkland C, King M, Cox A, Kim K, Pack DW. Precise control of PLG microsphere size provides enhanced control of drug release rate. J Control Release 2002;82:137–147.
- Bodmer D, Kissel T, Traechslin E. (1992). Factors influencing the release of peptides and proteins from biodegradable parenteral depot systems. J Control Rel 2:129–138.
- Dahut WL, Lakhani NJ, Gulley JL, Arlen PM, Kohn EC, Kotz H et al. Phase I clinical trial of oral 2-methoxyestradiol, an antiangiogenic and apoptotic agent, in patients with solid tumors. Cancer Biol Ther 2006;5:22–27.
- Freiberg S, Zhu XX. Polymer microspheres for controlled drug release. Int J Pharm 2004;282:1–18.
- Heijckmann CA, Menheere PP, Sels JP, Beuls EA, Wolffenbuttel BH. Clinical experience with Sandostatin LAR in patients with acromegaly. Neth J Med 2001;59:286–291.
- Jackson JK, Hung T, Letchford K, Burt HM. The characterization of paclitaxel-loaded microspheres manufactured from blends of poly(lactic-co-glycolic acid) (PLGA) and low molecular weight diblock copolymers. Int J Pharm 2007;342:6–17.
- Jain RA. The manufacturing techniques of various drug loaded biodegradable poly(lactide-co-glycolide) (PLGA) devices. Biomaterials 2000;21:2475–2490.
- Jehana JDJ, Murry AM, Treston AM. (2006). Phase I safety, pharmacokinetic and pharmacodynamic studies of 2-methoxyestradiol alone or in combination with docetaxel in patients with locally recurrent or metastatic breast cancer. Invest New Drugs 25:41–48.
- Jeong YI, Song JG, Kang SS, Ryu HH, Lee YH, Choi C et al. Preparation of poly(DL-lactide-co-glycolide) microspheres encapsulating all-trans retinoic acid. Int J Pharm 2003;259:79–91.
- Kashappa GH, Desai SR, Mallery SP, Schwende M. (2008). Effect of formulation parameters on 2-methoxyestradiol release from injectable cylindrical poly (DL-lactide-co-glycolide) implants. Eur J Pharm Biopharm 7:187–198.
- Kimiko M,Takehisa N, Mitsuhiko S, Fuminori I, Shizutoshi A, Chie K, Hiroyuki I. (2004). Efficient intracellular delivery of rifampicin to alveolar macrophages using rifampicin-loaded PLGA microspheres: effects of molecular weight and composition of PLGA on release of rifampicin. Colloids and SurfacesB: Biointerfaces, 36, 35–42.
- Liggins RT, Burt HM. Paclitaxel loaded poly(L-lactic acid) (PLLA) microspheres. II. The effect of processing parameters on microsphere morphology and drug release kinetics. Int J Pharm 2004;281:103–106.
- Mitwally MF, Diamond MP, Ayres J, Khan I, Shamma FN, Faki MH. (2005). Low-dose Lupron-Depot with GnRH antagonist rescue: a novel pituitary down-regulation protocol during controlled ovarian hyperstimulation (COH) for assisted reproductive technology (ART). Fertil Steril 84:321–22.
- Oh DS, Pisegna JR. (2001). A pilot study of Sandostatin LAR in combination with human recombinant alpha interferon in treating patients with metastatic neuroendocrine tumors. Gastroenterology, 120, 613.
- Schwendeman SP, Cardamone M, Klibanov A. (1996). Microparticulate Systems for the Delivery of Proteins and Vaccines. New York: Marcel-Dekker, pp. 1–49.
- Sun Y, Wang J, Zhang X, Zhang Z, Zheng Y, Chen D et al. Synchronic release of two hormonal contraceptives for about one month from the PLGA microspheres: in vitro and in vivo studies. J Control Release 2008;129:192–199.
- Sutherland TE, Anderson RL, Hughes RA. (2007). 2-Methoxyestradiol − a unique blend of activities generating a new class of anti-tumour/anti-inflammatory agents. Drug Discovery Today 12:13–14.
- Wang Y, Guo R, Cao XY, Shen MG, Shi XY. (2011). Encapsulation of 2-methoxyestradiol within multifunctional poly(amidoamine) dendrimers for targeted cancer therapy. Biomaterials 32:3322–3329.