Abstract
Topical administration of celecoxib proved to be an effective mean of preventing skin cancer development and improving anticancer drugs effectiveness in skin tumors treatment. The aim of this study was the development of an effective topical formulation of celecoxib, able to promote drug skin delivery, providing its in depth penetration through the skin layers. Three kinds of vesicular formulations have been investigated as drug carriers: liposomes containing a surfactant, or transfersomes and ethosomes, containing suitable edge activators. Firstly, the effect of membrane composition variations on the system performance has been evaluated for each vesicle type. Selected formulations were characterized for particle size, polydispersity index and encapsulation efficiency. The best formulations were subjected to ex vivo permeation studies through excised human skin. All vesicular formulations markedly (p < 0.001) improved the drug amount penetrated into the skin with respect to an aqueous suspension, from 2.0 to 6.5, up to 9.0 folds for liposomes, transfersomes and ethosomes, respectively. In particular, ethosomes containing Tween 20 as edge activator not only showed the best vesicle dimensions and homogeneity, and the highest encapsulation efficacy (54.4%), but also enabled the highest increase in drug penetration through the skin, probably due to the simultaneous presence in their composition of ethanol and Tween 20, both acting as permeation enhancers. Therefore, among the various vesicular formulations examined in the study, Tween 20-ethosomes can be considered the most promising one as carrier for topical celecoxib applications aimed to prevent skin cancer development and increase the anticancer drugs effectiveness against skin tumors.
Introduction
Skin malignancies are the most common type of cancer in humans (Stern, Citation2010; American Cancer Society, Citation2011). In particular, the frequency of appearance of skin cancers in the United States is higher than that of all the other types of cancers combined (Rogers et al., Citation2010). Considering the high morbidity and mortality associated with these pathologies, the importance of prevention and early diagnosis is strongly increasing, together with the search for innovative and more effective treatments (Martinez & Otley Citation2001). Destructive modalities, such as surgical excision, cryosurgery, electrodessication and curettage, still represent the initial treatments rendered for many cases of non-melanoma skin cancers and pre-malignant lesions. However, although effective, these procedures are easily applicable on an isolated or limited number of lesions, often require local anesthesia and can result in scarring and pigmentary alterations. Furthermore, also the direct patient cost, due to hospitalization and prolonged postoperative recovery time should be taken into account (McGillis & Fein, Citation2004). Consequentially, this has led to the development of a variety of pharmacologic alternatives, including topical therapies. In particular, topical pharmacotherapy is considered an interesting approach in selected skin alterations, being a noninvasive and self-administered treatment, and, in particular, it represents a highly desirable alternative option in patients with lesions located on cosmetically sensitive areas (Micali et al., Citation2010).
Celecoxib (CXB) is a non-steroidal anti-inflammatory drug (NSAID) selective cyclooxygenase-2 (COX-2) inhibitor. CXB is available on the market in capsule dosage form for oral administration and it is generally used for the treatment of rheumatoid arthritis, osteoarthritis and acute pain. Nevertheless, topical administration of CXB has proven to be an effective mean of preventing skin cancer development in numerous animal models of skin alterations, such as 4-nitroquinoline-1-oxide-induced lingual epithelium modifications (Sohrabi et al., Citation2009) and ultraviolet light B-induced skin cancer in mice (Wilgus et al., Citation2003; Hatton et al., Citation2005). Although not effective in inducing the regression of established skin tumors when used alone, CXB has even shown the potential of increasing the effectiveness of anticancer drugs, in a chemotherapeutic regimen. For example, the association of topical CXB with 5-FU led to increased regression of cancer in mice, compared to treatments with only 5-FU (Wilgus et al., Citation2004). Although the exact mechanisms to explain the potential of CXB administrations in the prevention and treatment of skin cancer are still unclear, its effectiveness is widely accepted. Moreover, topical formulations of COX-2 inhibitors can represent an interesting approach for the treatment of the different COX-2 mediated skin diseases, such as inflammation, wounds, pain and skin tumors (Lee et al., Citation2003). This makes the development of effective CXB topical formulation highly desirable.
It is known that the main limiting step to drug penetration across the skin is represented by the stratum corneum (Trommer & Neubert, Citation2006). Different topical delivery systems of CXB have been recently investigated, which exploit some of the means proposed to overcome the skin barrier, such as the use of penetration enhancers (Yener et al., Citation2003) or microemulsions (Subramanian et al., Citation2004) or multivesicular liposomes (Jain et al., Citation2007) or nanostructured lipid carriers (Joshi & Patravale, Citation2008), thus demonstrating the increasing interest in this field. However, at the best of our knowledge, no topical formulations of CXB are presently available on the market.
Among the different approaches for achieving an effective topical drug delivery, liposomes have been widely used as safe and effective vehicles, due to their proved potential in improving skin penetration and clinical efficacy of several drugs (Gregoriadis, Citation2000; Verma et al., Citation2003; Mura et al., Citation2007). Their delivery mechanism is mainly associated with accumulation of the liposomes in the stratum corneum and upper skin layers, which act as a local drug reservoir. However, it seems that traditional liposomes do not deeply penetrate skin, but rather remain confined to upper layers of the stratum corneum (Touitou et al., Citation2000). Therefore, new strategies have been developed in the attempt of enhancing the skin penetration ability of liposomes. These include the addition in the lipid phase, together with phosphatidylcholine and cholesterol, of a cationic or anionic surfactant, thus increasing the bilayer fluidity (Trotta et al., Citation2004; Mura et al., Citation2008; Bragagni et al., Citation2010). Moreover, the complete replacement of cholesterol with a suitable surfactant acting as “edge activator” led to formation of very elastic and flexible “ultradeformable” vesicular systems, named “transfersomes,” firstly introduced by Cevc (Citation1996). These ultra-deformable vesicles can penetrate the skin membranes intact, by squeezing themselves along the intracellular sealing lipid of the stratum corneum, and localize the drug at high concentrations in the deepest layers of the skin (Cevc & Blume, Citation2001; El Maghraby et al., Citation2008; Maestrelli et al., Citation2010). Another interesting alternative proposed to overcome the problems of poor skin permeability of classic liposomes is the use of “ethosomes,” lipid vesicles composed of phospholipids, water and ethanol in relatively high concentrations (Touitou et al., Citation2000). Ethosomes, revealed to be more efficient than classic liposomes in improving skin delivery of various drugs in terms of quantity and depth (Dayan & Touitou, Citation2000; Godin & Touitou, Citation2003; Paolino et al., Citation2005; Rattanapak et al., Citation2012). These effects have been attributed not only to the solvent action of ethanol on the stratum corneum of the skin but also to the higher deformability and malleability of these vesicles that can better penetrate intact through the skin (Elsayed et al., Citation2006; Elsayed et al., Citation2007; Dubey et al., Citation2007).
On the basis of these premises, we considered it worth of interest to develop a new nano-vesicular system as possible carrier to improve CXB skin permeability. With this aim we developed, evaluated and compared the effectiveness of three different kinds of liposomal formulations, i.e. traditional liposomes including a ionic surfactant in their composition, ethosomes and transfersomes. For each kind of vesicular system, a preliminary screening was performed to evaluate the effect of variations of lipid phase composition (type of surfactant for traditional liposome, or type of edge activator for transfersomes and ethosomes) and select the best one in terms of drug entrapment efficiency and vesicle size. The final selected formulations were then evaluated for drug skin penetration and permeation properties using excised human skin.
Materials and methods
Materials
Celecoxib (CXB) was obtained by extraction with methanol from Celebrex® capsules (200 mg, Pfizer Italia Srl, Latina, Italy) according to a previously established procedure (Mennini et al., Citation2012). Its identity and purity was checked by Differential Scanning Calorimetry (Mettler TA 4000 Stare apparatus equipped with DSC 25 cell, Mettler Toledo, Greifensee, Switzerland), X-ray powder diffractometry (Bruker D8, Bruker, Wisconsin, USA) and 1H-NMR spectroscopy (Bruker Avance 400 MHz, Karlsruhe, Germany) (Mennini et al., Citation2012). The DSC curve of CXB (10°C min−1, 30–250°C temperature range, static air) proved its crystalline anhydrous state, exhibiting a single endothermic fusion event peaked at 162.2°C (ΔH 91.7 J/g). The thermal behavior of the sample was in full agreement with literature data for both crystalline CXB and products obtained by its recrystallization from different solvents, including methanol, thus indicating the absence of polymorph or pseudopolymorph formation (Chawla et al., Citation2003). The purity of CXB, determined by DSC analysis, was 98.7%. Cholesterol (CHOL), dicethylphosphate (DCP), dipotassium glycyrrizinate (DPG), ethanol 96%, 1-α-phosphatidylcholine (PC), Sephadex G50 and sodium cholate (SC) were provided by Sigma Aldrich Srl, (Milan, Italy). Tween 20, Tween 80, stearylamine (SA) and Span 80 were purchased from Fluka, Sigma-Aldrich group (Basel, Switzerland). Dulbecco’s modified Eagle’s medium (DMEM), fetal bovine serum (FBS) and penicillin-streptomycin 100X solution were obtained from Histo-Line Laboratories (Milan, Italy). Phosphate buffer solution pH 7.4, 0.1 M (PBS) was prepared according to Eur. Pharm. 6th Ed. All other reagents and solvents were of analytical grade.
Assay of CXB
The drug was assayed by HPLC analysis (Merck Hitachi Elite Lachrom chromatograph) equipped with a UV–visible L-2400 detector (Shimadzu, Kyoto, Japan). CXB was separated using a C18 Supelcosil column (250 × 4.6 mm, 5 µm), a 70:30 v/v methanol:water mixture as mobile phase, a flow rate of 0.9 mL/min, at a temperature of 25°C. CXB was monitored at 250 nm. The retention time of CXB under these experimental conditions was 6.9 min. The lower limit of quantification (LOQ) and the limit of detection (LOD) were 0.21 mg/L and 0.06 mg/L, respectively.
Preparation of vesicular systems
Deformable liposomes and transfersomes were obtained by thin layer evaporation technique (Bragagni et al., Citation2010). For deformable liposomes, the lipid phase consisted of PC (230 mg), CHL (33 mg), and a cationic or anionic surfactant (SA or DCP) (12 mg). These components were dissolved in chloroform, together with 25 mg of CXB. The solvent was then removed under reduced pressure in a rotary evaporator at 58°C, to obtain a thin film on the flask wall. Evaporation was continued for 2 h after the dry residue appeared, to completely remove all the solvent. The film was then hydrated by adding 5 mL of water. The hydrated film was subjected to water bath heating at 58°C for 10 min and vortexing for 2 min; the treatment was repeated, performing four cycles in total. The dispersion was hermetically sealed, protected from light and stored at 4°C.
Transfersomes were prepared according to the same procedure, but using a lipid phase having the following composition: PC (218 mg) and an edge activator (DPG or SC or Span 80, or Tween 20 or Tween 80) (33 mg).
Ethosomes were prepared according to the method of Touitou et al. (Citation2000), slightly modified. PC (218 mg) and an edge activator (DPG or SC or Span 80, or Tween 20 or Tween 80) (33 mg) together with 25 mg of CXB were dissolved in 1.5 mL of 96% ethanol in a 10 mL flask. The flask was transferred into a thermostatic bath at 65°C; while the ethanolic solution was vigorously stirred, 3.5 mL of water were added drop wise. The mixture was hermetically closed, to avoid solvent evaporation, and maintained under stirring at 65°C for 10 min. The obtained dispersion was left cooling down, sealed, protected from light and stored at 4°C.
Characterization of the vesicles
Particle size, polydispersity index (PDI) and Zeta potential of the vesicles were determined by dynamic light scattering using a Zetasizer Nano ZS90 (Malvern Instruments). Suspensions were properly diluted with distilled water to avoid multiscattering phenomena. Six independent samples were taken from each dispersion. The effective formation and the morphological characteristics of the vesicles were investigated by optical microscopy (Labophot-2A optical microscope, Nikon) and transmission electron microscopy (TEM) (CM12 TEM, Philips, Netherlands). For TEM analysis, a drop of the vesicle dispersion was placed onto a Cu/C grid and stained by adding a drop of 2% (w/w) uranyl acetate solution; the excess solution was removed by using a filter paper, followed by a through air-drying.
For encapsulation efficacy (EE%) determination, the free drug was separated by size exclusion chromatography from the encapsulated CXB. Columns loaded with 5 mL of Sephadex G50 gel were prepared and each of them was employed for the separation of 0.25 mL of liposomal suspension, using water as mobile phase. For their relatively large dimensions, vesicles present in the suspension did not bind to the gel and rapidly eluted from the columns as an opaque liquid. The liquid collected from the columns was quantitatively diluted in methanol (the ratio vesicular suspension: solvent was approximately 1:3) to favor the disruption of the vesicles. The dispersion was left stirring overnight, treated with bath sonication for 30 min and centrifuged at 6000 rpm for 15 min to precipitate the lipids. The supernatant was collected, centrifuged, opportunely diluted with mobile phase and analyzed by HPLC. The total drug present in the vesicles was obtained disrupting with the same procedure a sample of the initial vesicular suspension, not introduced in chromatographic columns. The encapsulation efficiency (EE%) was calculated according to the following equation:
Each result is the mean of five separate experiments.
Stability studies
Selected formulations of the three types of vesicles examined (liposomes containing stearylamine, transfersomes and ethosomes) were stored at 4°C and at room temperature (25°C) for 30 days, and monitored every 10 days for physical stability (visual observation and mean size determination) and for drug entrapment efficacy.
Ex vivo skin permeation studies
A sample of human skin was obtained from a mammary reduction procedure from a single donor. Immediately after the surgical intervention, the subcutaneous fat tissue was removed by a lancet. The remaining tissue, formed by stratum corneum and epidermis, was divided into portions of approximately 1.5 × 1.5 cm. These fragments were immersed in DMEM containing a 10% FBS, 1% penicillin and 1% streptomycin, conserved in a CO2 cell culture incubator and used within 24 h. Before the experiments each sample was washed with PBS, gently dried by filter paper, weighted and mounted in a Franz diffusion cell (Rofarma, Italy) with an orifice diameter of 10 mm. The dermal side of the skin was in contact with the receptor phase, which was PBS (7 mL) maintained at 37°C and under gentle agitation. The donor chamber was loaded with 1 mL of the formulation to study. Three different formulations were investigated: deformable liposomes, transfersomes and ethosomes. In all cases, the amount of loaded drug was 0.5% w/v. After 4 h, the sample of human skin was removed, accurately washed with PBS, dried by filter paper and frozen at −80°C for further analyses. The drug content in the receiving compartment medium was assayed by HPLC. Each result is the mean of five separate experiments.
To determine the amount of CXB retained by the skin, the drug was extracted with methanol from each sample of tissue collected at the end of permeation experiments (Minghetti et al., Citation2006). The human skin fragments were thawed out and cut into thin slices (approximately 3 mm wide) using a scalpel. The slices were transferred to a glass vial containing 1 mL of methanol. The vial was closed and the content was gently stirred for 12 h. The dispersion, which had a homogeneous opaque aspect, was centrifuged (Hermle Z200A, USA) at 6000 rpm for 15 min and the supernatant was analyzed by HPLC. The extraction and analysis procedures were repeated twice on the precipitate, using 1 mL of fresh methanol each time. The three aliquots of methanol were separately analyzed by HPLC and the quantities of CXB extracted were summed up.
Statistical analysis
Results of vesicle characterization, stability studies and permeation experiments were statistically analyzed by ANOVA (one-way analysis of variance) followed by the Student-Neuwman Keuls multiple comparison post-test (GraphPad Prism, version 4). The differences were considered statistically significant when p < 0.05.
Results and discussion
The final goal of the present work was the development of a CXB vesicular formulation able to assure an effective delivery of the drug into the deepest strata of the skin. Deformable liposomes, transfersomes and ethosomes were the three types of vesicular systems investigated for this purpose.
In the development of a vesicular formulation, there are numerous parameters to be taken into account, each of which can sensitively influence the characteristics of the final preparation. Thus, preliminary studies were carried out to opportunely select the most suitable conditions for vesicle preparation, using optical microscopy as a screening technique to rapidly verify the actual formation of the vesicles or evidence possible formulation problems.
As a first step, the technique pointed out problems in obtaining homogeneous vesicular dispersions when the drug was added to the lipophilic phase of the vesicles at concentrations higher than 0.5% w/v, evidencing in such cases the presence of not entrapped CXB, precipitated out as crystals. For this reason, all further studies were carried out using a CXB concentration in the vesicular dispersions of 0.5% w/v.
The following phase of the study was devoted to the selection of the best composition for each kind of vesicular system investigated. The lipid phase compositions of the different formulations examined in this study are presented in .
Table 1. Composition of the lipid phase of the different kinds of vesicular systems examined.
The surfactants tested in the case of classic liposome formulations were the cationic stearylamine (SA) and the anionic dicethylphosphate (DCP), both reported as able to improve the flexibility of vesicle membrane and its ability to penetrate into the skin (Trotta et al., Citation2004; Mohammed et al., Citation2004; Fang et al., Citation2006; Mura et al., Citation2008). However, the presence of DCP give rise to formation of unstable vesicular dispersions, and then it was excluded from further studies, and only SA was used as surfactant.
As for the choice of the “edge activators” to use for preparation of transfersomes or ethosomes, the candidates examined were dipotassium glycyrrizinate (DPG), Span 80, sodium cholate (SC), Tween 20 and Tween 80. However, addition of DPG or Span 80 to the lipid phase did not allow formation of homogeneous and stable vesicles, and thus both these components were ruled out from vesicle compositions.
The different vesicle formulations selected after this screening were then characterized for mean size and polydispersity index (PDI) and for drug entrapment efficiency. The results of these studies are summarized in .
Table 2. Mean vesicle size (n = 6), polydispersity index (PDI) (n = 6) and entrapment efficiency (EE%) (n = 5) of the different CXB-loaded (0.5% w/v) vesicular dispersions.
In order to evaluate the effect of drug loading on the vesicle properties, for every kind of formulation, also the corresponding empty vesicles were obtained, having the same lipid phase composition. No statistically significant variations in size or in PDI (p > 0.05) were found for drug-loaded vesicles with respect to the empty ones (data not shown).
Liposomes containing SA showed the largest mean dimensions and, at the same time, the lowest entrapment efficiency with respect to all the other formulations (Batch A, ). It is possible to hypothesize that this surfactant can compete with CXB when arranging in the membrane bilayer, thus excluding the drug from assembling in the membrane structure.
In the case of ultradeformable vesicles (batches B, C and D) the introduction of the different kinds of edge activators in the formulations did not influence markedly the particle size, which varied in the 483–516 nm range. On the contrary, the type of edge activator clearly affected the drug entrapment efficiency of the vesicles. The best results in terms of greater vesicle homogeneity (lower PDI) and higher EE% were obtained when using Tween 80.
An evident effect of the kind of edge activator on the vesicle dimensions was instead observed in the case of ethosomes (batches E, F and G), whose mean size varied from 484 nm in the presence of SC to 258 nm in the presence of Tween 20. In particular, a very homogeneous dispersion was obtained when Tween 20 was employed (formulation F). Moreover, the use of this component also allowed the obtainment of the highest EE% (54.4%) of all the examined formulations. In the case of ethosomes prepared with SC, it was not possible to determine the EE% value, since the suspension showed physical instability after less than 24 h from the vesicle preparation.
On the basis of these findings, batches A, D and F were selected as the best formulations of the three different types of vesicular systems considered. TEM analysis performed on these selected batches () demonstrated in all cases the actual formation of nano-sized vesicles. However, the vesicles of the liposomal formulation containing SA (batch A) were poorly homogeneous in size and shape, in agreement with the high PDI value obtained by light-scattering analysis (). Some reduction in vesicle mean size, and polydispersity was noticed for formulation of transfersomes containing Tween 80 (batch D) (), while the most homogeneous nano-sized vesicular dispersion was observed for ethosomes containing Tween 20 (batch F) (), thus confirming, also in these cases, the light-scattering data ().
Figure 1. Transmission electron micrographs of liposomes (batch A), transfersomes (batch D) and ethosomes (batch F; see and for batch formulation composition).
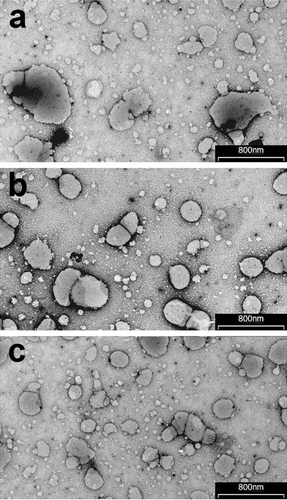
The selected vesicular systems were then subjected to a 30 days storage at 4°C and at room temperature (25°C), in order to evaluate their physical and chemical stability in terms of vesicle size and homogeneity and drug entrapment efficiency, respectively (). No sedimentation phenomena were observed in any formulation at the end of the storage period, and not significant variations in size were observed, even though the average size and PDI of all formulations stored at 25°C slightly increased (p > 0.05). The entrapment efficiency of formulations stored at 4°C was practically unchanged for all formulations. On the contrary, a reduction of about 20% was observed for liposomes containing stearylamine (Batch A) after 30 days storage at room temperature. This effect was less evident for transfersomes, where a loss of about 8% was found, while it was not statistically significant (p > 0.05), with a loss lower than 4%, in the case of ethosomes, indicating the higher chemical stability of this last kind of vesicular dispersion.
Table 3. Mean vesicle size, polydispersity index (PDI) and entrapment efficiency (EE%) of the selected CXB-loaded (0.5% w/v) vesicular systems after 30 days storage at 4°C and room temperature (25°C).
The selected vesicular dispersions (batches A, D and F) were finally examined by ex vivo penetration and permeation studies trough excised human skin, in comparison with a simple aqueous suspension of the drug at the same concentration. In order to avoid possible phenomena of alterations of the skin and to better mimic the actual physiological conditions, the receiving compartment of the Franz cells was a pH 7.4 buffered aqueous solution simulating the plasmatic compartment, without addition of surfactants or organic solvents which are sometimes used to improve drug solubility in the acceptor medium.
With all the tested formulations, the concentration of drug permeated through the skin after 4 h was practically undetectable, being under the LOQ of the HPLC assay method (0.21 µg/mL). This finding indicated that our first prefixed goal of not obtaining a systemic absorption of the drug after topical application of the vesicular CXB formulations has been actually achieved.
In order to determine the penetration ability through the skin of the drug carried by the different vesicular systems, at the end of permeation experiments the skin was washed with PBS, to eliminate the drug present on the surface, and then extracted with methanol according to the procedure described in the corresponding Methods section.
shows the amounts of CXB retained in the human skin samples for the different formulations tested. As expected, all the vesicular formulations showed better ability to promote drug penetration into the skin layers with respect to the simple aqueous suspension. However, significant differences (p < 0.05) were observed among the three types of vesicles examined.
Figure 2. Amount of Celecoxib (CXB) penetrated into the excised human skin after 4 h from application of the three kinds of selected vesicular formulations (liposomes, batch A; transfersomes, batch D; ethosomes, batch F) and of an aqueous drug suspension as reference (see and for batch formulation composition; each result is the mean of five separate experiments).
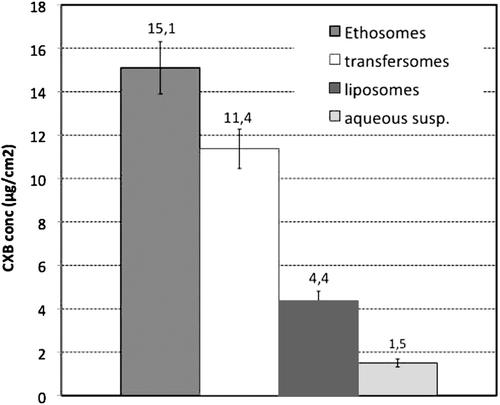
In fact, the amount of drug penetrated into the skin when using the formulation of ultradeformable vesicles was almost 2.6 folds greater than that one obtained with liposomes containing SA. Moreover, the CXB quantity delivered into the skin by the ethosomal formulation was even superior, being almost 3.5 folds higher than that obtained with liposomes, and 1.3 folds higher than that obtained by using transfersomes vesicles.
The greater efficacy in improving the drug skin penetration showed by transfersomes and ethosomes might be attributable to the particular structure of these vesicles, which include permeation enhancers in their composition. Two different mechanisms of action have been proposed to explain the enhancing effect exploited by the edge activator. According to the intracellular mechanism, both conventional and ultradeformable liposomes may fuse and mix with skin lipids, changing the enthalpy of the lipid-related transitions of the stratum corneum; the introduction of edge activators would somehow influence positively this mechanism (Kirjavainen et al., Citation1999). Considering an extracellular pathway, the presence of the activator would reduce the energy required for the deformation and would allow these specialized vesicles to “squeeze” trough the small pores of the membranes. Both these mechanisms may be simultaneously operative.
The better performance of ethosomal formulations may be attributed to the combined presence of edge activator and of ethanol, which is a known permeation enhancer. Permeation studies of drugs from ethosomal systems versus hydro-ethanolic solutions showed that the permeation enhancement from ethosomes was much greater than from ethanol alone, pointing out a synergistic mechanism could occur between ethanol, vesicles and skin lipids (Dayan & Touitou, Citation2000). Ethanol could provide the vesicles with flexible characteristics, allowing them to more easily penetrate into deeper layers of the skin. It was also proposed that ethosomes may influence the bilayer structure of the stratum corneum and this may lead to enhancement of drug penetration (Kirjavainen et al., Citation1999). Most likely, a combination of various processes contributes to the enhancing effect obtained by the introduction of ethanol in the composition.
Furthermore, ethosomes prepared with Tween 20 as edge activator (formulation F) exhibited particularly favorable technological characteristics. In particular, in addition to the high homogeneity of the dispersion, the reduced dimensions of these vesicles, which have a mean diameter below 260 µm, could positively contribute to their enhancing effect on drug penetration through the skin.
Conclusions
Three different kinds of nano-vesicular systems, i.e. traditional liposomes containing a surfactant, transfersomes and ethosomes, were developed and fully characterized in order to compare their performance as potential carriers for achieving an effective skin delivery of CXB. The best formulations selected for each kind of vesicles were tested ex vivo to evaluate their effective ability to deliver the drug into the human skin, in comparison with a simple aqueous suspension containing the same amount of drug (0.5% w/v). All the vesicular formulations markedly (p < 0.001) improved the amount of drug penetrated into the skin layers with respect to the simple aqueous suspension, from a minimum of 200% in the case of liposomes, to 650% for transfersomes, up to a maximum of 900%, in the case of ethosomes.
In particular, ethosomes containing Tween 20 as edge activator not only showed the best characteristics in terms of vesicle dimensions and homogeneity, and enabled the highest encapsulation efficacy (54.4%), but also gave rise to the highest increase in drug penetration ability through the skin, probably due to the simultaneous presence in their composition of ethanol and Tween 20, both acting as permeation enhancers.
According to these results, entrapment of CXB into Tween 20-containing ethosomes proved to be the most effective formulative strategy for improving the therapeutic efficacy of the drug for topical administration on intact skin. Thus, according to literature data (Sohrabu et al., 2003; Wilgus et al., Citation2003; Lee et al., Citation2003; Wilgus et al., Citation2004), the developed formulation could be particularly useful for topical applications of CXB aimed to prevent skin cancer development and to increase the effectiveness of anticancer drugs in skin tumors treatment.
Declaration of interest
The authors report no declarations of interest.
References
- American Cancer Society. Global cancer facts & figures. 2nd ed. Atlanta: American Cancer Society, 2011.
- Bragagni M, Maestrelli F, Mennini N, Ghelardini C, Mura P. Liposomal formulations of prilocaine: effect of complexation with hydroxypropyl-ß-cyclodextrin on drug anesthetic efficacy. J Liposome Res 2010;20:315–322.
- Cevc G. Transfersomes, liposomes and other lipid suspensions on the skin: permeation enhancement, vesicle penetration, and transdermal drug delivery. Crit Rev Ther Drug Carrier Syst 1996;13:257–388.
- Cevc G, Blume G. New, highly efficient formulation of diclofenac for the topical, transdermal administration in ultradeformable drug carriers, Transfersomes. Biochim Biophys Acta 2001;1514:191–205.
- Chawla G, Gupta P, Thilagavathi R, Chakraborti AK, Bansal AK. Characterization of solid-state forms of celecoxib. Eur J Pharm Sci 2003;20:305–317.
- Dayan N, Touitou E. Carriers for skin delivery of trihexyphenidyl HCl: ethosomes vs. liposomes. Biomaterials 2000;21:1879–1885.
- Dubey V, Mishra D, Jain NK. Melatonin loaded ethanolic liposomes: physicochemical characterization and enhanced transdermal delivery. Eur J Pharm Biopharm 2007;67:398–405.
- El Maghraby GM, Barry BW, Williams AC. Liposomes and skin: from drug delivery to model membranes. Eur J Pharm Sci 2008;34:203–222.
- Elsayed MM, Abdallah OY, Naggar VF, Khalafallah NM. Deformable liposomes and ethosomes: mechanism of enhanced skin delivery. Int J Pharm 2006;322:60–66.
- Elsayed MM, Abdallah OY, Naggar VF, Khalafallah NM. Deformable liposomes and ethosomes as carriers for skin delivery of ketotifen. Pharmazie 2007;62:133–137.
- Fang JY, Hwang TL, Huang YL, Fang CL. Enhancement of the transdermal delivery of catechins by liposomes incorporating anionic surfactants and ethanol. Int J Pharm 2006;310:131–138.
- Godin B, Touitou E. Ethosomes: new prospects in transdermal delivery. Crit Rev Ther Drug Carrier Syst 2003;20:63–102.
- Gregoriadis G. Liposome technology. II ed. Boca Raton, FL: CRC Press, 2000:367–398.
- Hatton JL, Parent AE, Hoppes T, Kusewitt DF, Vanbuskirk AM, Oberyszyn TM. Topical celecoxib decreases the exacerbated UVB-induced skin inflammation and carcinogenesis seen in immunosuppressed mice. Proc Amer Assoc Cancer Res 2005;46.
- Jain SK, Gupta Y, Jain A, Bhola M. Multivesicular liposomes bearing celecoxib-beta-cyclodextrin complex for transdermal delivery. Drug Deliv 2007;14:327–335.
- Joshi M, Patravale V. Nanostructured lipid carrier (NLC) based gel of celecoxib. Int J Pharm 2008;346:124–132.
- Lee JL, Mukhtar H, Bickers DR, Kopelovich L, Athar M. Cyclooxygenases in the skin: pharmacological and toxicological implications. Toxicol Appl Pharmacol 2003;192:294–306.
- Kirjavainen M, Mönkkönen J, Saukkosaari M, Valjakka-Koskela R, Kiesvaara J, Urtti A. Phospholipids affect stratum corneum lipid bilayer fluidity and drug partitioning into the bilayers. J Control Release 1999;58:207–214.
- Maestrelli F, González-Rodríguez ML, Rabasco AM, Ghelardini C, Mura P. New “drug-in cyclodextrin-in deformable liposomes” formulations to improve the therapeutic efficacy of local anaesthetics. Int J Pharm 2010;395:222–231.
- Martinez JC, Otley CC. The management of melanoma and nonmelanoma skin cancer: a review for the primary care physician. Mayo Clin Proc 2001;76:1253–1265.
- McGillis ST, Fein H. Topical treatment strategies for non-melanoma skin cancer and precursor lesions. Semin Cutan Med Surg 2004;23:174–183.
- Mennini N, Furlanetto S, Cirri M, Mura P. Quality by design approach for developing chitosan-Ca-alginate microspheres for colon delivery of celecoxib-hydroxypropyl-ß-cyclodextrin-PVP complex. Eur J Pharm Biopharm 2012;80:67–75.
- Micali G, Lacarrubba F, Dinotta F, Massimino D, Nasca MR. Treating skin cancer with topical cream. Expert Opin Pharmacother 2010;11:1515–1527.
- Minghetti P, Cilurzo F, Casiraghi A, Montanari L. Evaluation of ex vivo human skin permeation of genistein and daidzein. Drug Deliv 2006;13:411–415.
- Mohammed AR, Weston N, Coombes AG, Fitzgerald M, Perrie Y. Liposome formulation of poorly water soluble drugs: optimisation of drug loading and ESEM analysis of stability. Int J Pharm 2004;285:23–34.
- Mura P, Maestrelli F, González-Rodríguez ML, Michelacci I, Ghelardini C, Rabasco AM. Development, characterization and in vivo evaluation of benzocaine-loaded liposomes. Eur J Pharm Biopharm 2007;67:86–95.
- Mura P, Capasso G, Maestrelli F, Furlanetto S. Optimization of formulation variables of benzocaine liposomes using experimental design. J Liposome Res 2008;18:113–125.
- Paolino D, Lucania G, Mardente D, Alhaique F, Fresta M. Ethosomes for skin delivery of ammonium glycyrrhizinate: in vitro percutaneous permeation through human skin and in vivo anti-inflammatory activity on human volunteers. J Control Release 2005;106:99–110.
- Rattanapak T, Young K, Rades T, Hook S. Comparative study of liposomes, transfersomes, ethosomes and cubosomes for transcutaneous immunisation: characterisation and in vitro skin penetration. J Pharm Pharmacol 2012. DOI: 10.1111/j.2042-7158.2012.01535.x
- Rogers HW, Weinstock MA, Harris AR, Hinckley MR, Feldman SR, Fleischer AB, Coldiron BM. Incidence estimate of nonmelanoma skin cancer in the United States, 2006. Arch Dermatol 2010;146:283–287.
- Sohrabi M, Soleimani J, Roshangar L, Vatansever S, Arbabi F, Khaki AA, Abbasi MM, Dustar Y, Javadzadeh Y. The effect of dietary and topical celecoxib on 4-nitroquinoline-1-oxide-induced lingual epithelium alterations in rat. J Pak Med Assoc 2009;59:769–774.
- Stern RS. Prevalence of a history of skin cancer in 2007: results of an incidence-based model. Arch Dermatol 2010;146:279–282.
- Subramanian N, Ghosal SK, Moulik SP. Topical delivery of celecoxib using microemulsion. Acta Pol Pharm 2004;61:335–341.
- Touitou E, Dayan N, Bergelson L, Godin B, Eliaz M. Ethosomes - novel vesicular carriers for enhanced delivery: characterization and skin penetration properties. J Control Release 2000;65:403–418.
- Trommer H, Neubert RH. Overcoming the stratum corneum: the modulation of skin penetration. A review. Skin Pharmacol Physiol 2006;19:106–121.
- Trotta M, Peira E, Carlotti ME, Gallarate M. Deformable liposomes for dermal administration of methotrexate. Int J Pharm 2004;270:119–125.
- Verma DD, Verma S, Blume G, Fahr A. Liposomes increase skin penetration of entrapped and non-entrapped hydrophilic substances into human skin: a skin penetration and confocal laser scanning microscopy study. Eur J Pharm Biopharm 2003;55:271–277.
- Wilgus TA, Koki AT, Zweifel BS, Rubal PA, Oberyszyn TM. Chemotherapeutic efficacy of topical celecoxib in a murine model of ultraviolet light B-induced skin cancer. Mol Carcinog 2003;38:33–39.
- Wilgus TA, Breza TS Jr, Tober KL, Oberyszyn TM. Treatment with 5-fluorouracil and celecoxib displays synergistic regression of ultraviolet light B-induced skin tumors. J Invest Dermatol 2004;122:1488–1494.
- Yener G, Gönüllü U, Uner M, Degim T, Araman A. Effect of vehicles and penetration enhancers on the in vitro percutaneous absorption of celecoxib through human skin. Pharmazie 2003;58:330–333.