Abstract
Enhancement of transdermal absorption through rat skin and stability of the human tyrosinase plasmid (P) using Tat (T) and an entrapment in elastic cationic niosomes (E) were described. E (Tween61:cholesterol:DDAB at 1:1:0.5 molar ratio) were prepared by the freeze-dried empty liposomes (FDELs) method using 25% ethanol. TP was prepared by a simple mixing method. TPE was prepared by loading T and P in E at the T:P:E ratio of 0.5:1:160 w/w/w. For gel formulations, P, TP, PE and TPE were incorporated into Carbopol 980 gel (30 µg of plasmid per 1 g of gel). For the transdermal absorption studies, the highest cumulative amounts and fluxes of the plasmid in viable epidermis and dermis (VED) were observed from the TPE of 0.31 ± 0.04 µg/cm2 and 1.86 ± 0.24 µg/cm2/h (TPE solution); and 4.29 ± 0.40 µg/cm2 and 25.73 ± 2.40 µg/cm2/h (TPE gel), respectively. Only plasmid from the PE and TPE could be found in the receiving solution with the cumulative amounts and fluxes at 6 h of 0.07 ± 0.01 µg/cm2 and 0.40 ± 0.08 µg/cm2/h (PE solution); 0.10 ± 0.01 µg/cm2 and 0.60 ± 0.06 µg/cm2/h (TPE solution); 0.88 ± 0.03 µg/cm2 and 5.30 ± 0.15 µg/cm2/h (PE gel); and 1.02 ± 0.05 µg/cm2 and 6.13 ± 0.28 µg/cm2/h (TPE gel), respectively. In stability studies, the plasmid still remained at 4 ± 2 °C and 25 ± 2 °C of about 48.00–65.20% and 27.40–51.10% (solution); and 12.34–38.31% and 8.63–36.10% (gel), respectively, whereas at 45 ± 2 °C, almost all the plasmid was degraded. These studies indicated the high potential application of Tat and an entrapment in elastic cationic niosomes for the development of transdermal gene delivery system.
Introduction
Currently, transdermal drug delivery is one of the most promising methods for drug administration. Increasing numbers of drugs are being added to the list of therapeutic agents that can be delivered to the systemic circulation via skin (Prausnitz et al., Citation2004). Currently available transdermal delivery systems are scopolamine (hyoscine) for motion sickness, clonidine and nitroglycerin for cardiovascular disease, fentanyl for chronic pain, nicotin to aid smoking cessation, oestradiol (alone or in combination with levonorgestrel or norethisterone) for hormone replacement and testosterone for hypogonadism (Patel et al., Citation2011). The efficient permeability of therapeutically active molecules through the biological membranes remains the most important hurdle for drug delivery. Elastic niosome is a non-ionic surfactant-based nanovesicle composes of nanovesicular fluidized compounds such as deoxycholate and ethanol (Choi & Maibach, Citation2005). Novel non-ionic surfactant-based elastic niosomes, containing ethanol as nanovesicular membrane fluidizer, was first described by Manosroi et al. (Citation2008). These nanovesicles can squeeze themselves and pass through a small pore in stratum corneum, which is smaller than their vesicular size. Hence, these types of nanovesicles were more efficient in delivering both low and high molecular weight drug in terms of quantity and depth (Choi & Maibach, Citation2005). Elastic niosomes also demonstrated prolonged release and better biological activity of the entrapped substances compared to conventional niosomes (Bouwstra et al., Citation2003; Manosroi et al., Citation2010a). Cell-penetrating peptides (CPPs), protein transduction domains (PTDs) or membrane transduction peptides (MTPs) consisting of 30 or less amino acids are classified as either cationic or amphipathic and have the ability to cross the cell membrane into cells. The ability of CPPs such as a transcriptional activating (Tat) protein of human immunodeficiency virus type 1 and penetratin to translocate into the cell is attributed to their amino acid sequence, which is mainly contributed by basic amino acids (Desai et al., Citation2010). Rothbard et al. (Citation2000) were the first to report the application of CPPs for the delivery of peptides into the skin. The conjugate R7-CsA (polyarginine-7-cyclosporin A) can release therapeutically-effective drug and its activity has been shown in a dose-dependent manner both in vitro and in vivo. Polylysine-9 (K9)- (Park et al., Citation2002) and Tat-coupled (Kim et al., Citation2003; Eum et al., Citation2005) antioxidative enzymes can translocate into epidermis and dermis. The Tat-linked small peptide GKH (glycine-lysine-histidine) showed 36 times more absorption than the GKH (Lim et al., Citation2003). This study investigated the transdermal absorption enhancement of tyrosinase plasmid through rat skin by Tat peptide and an entrapment in elastic cationic niosomes, and the potential application for further development to vitiligo gene therapy was anticipated.
Materials and methods
Materials
Human tyrosinase plasmid (pAH7/Tyr, P) was provided by Boehringer Ingelheim Company, Germany. The map of the pAH7/Tyr, containing 4986 base pairs with the CMV promoter is shown in . The Tat peptide (GRKKRRQRRRPPQRKC) was purchased from Chengdu KaiJie Bio-pharmaceutical Co., Ltd. (Chengdu, China). Tween61 (polyoxyethelene sorbitan monostearate), cholesterol and DDAB (dimethyl dioctadecyl ammonium bromide) were from Sigma Chemicals, St. Louis, MO. Phenol/chloroform/isoamylalcohol and ethanol were analytical grade reagents (Fluka, Buchs, Switzerland).
Preparation of elastic cationic niosomes
The 20 mM elastic cationic niosomes (E) were prepared by the freeze-dried empty liposomes (FDELs) method (Kikuchi et al., Citation1999). Briefly, Tween 61:cholesterol:DDAB at 1:1:0.5 molar ratio were mixed, placed in a clean, dry round-bottom flask and dissolved in 10 ml of chloroform. The solvent was removed by a rotary evaporator (R-124, Buchi, Flawil, Switzerland) at 50 ± 2 °C. The resulting film was dried by evacuation in a desiccator at room temperature (25 ± 2 °C) under reduced pressure for over 12 h and rehydrated with 10 ml of distilled water at 50 ± 2 °C for 30 min. The dispersion was sonicated using a microtip probe sonicator (Vibra Cell™, Sonics & Materials, Inc., Newton, CT) at pulse on 3.0, pulse off 1.0 with 33% amplitude for 15 min and centrifuged at 2190g, 4 °C for 1 min. The dispersion was further lyophilized overnight by a freeze-dryer (Alpha 1-2 LD model, Christ, Osterode am Harz, Germany) and kept at 4 °C until use. The lyophilized powder was reconstituted in 10 ml of 25% ethanol and the resulting dispersion was further sonicated at 4 °C in an ice bath for 15 min. The niosomal dispersion was centrifuged at 2190g, 4 °C for 1 min, filtered through a 0.45 µm membrane filter and kept at 4 °C until use.
Preparation of tyrosinase plasmid-loaded elastic cationic niosomes, Tat/tyrosinase plasmid and Tat/tyrosinase plasmid/elastic cationic niosomes
The tyrosinase plasmid-loaded elastic cationic niosomes (PE) at the P:E ratio of 1:160 w/w were prepared by incubating P with 20 mM E at room temperature (25 ± 2 °C) for 1 h using 100 µg of P per 16 mg of E. For Tat/tyrosinase plasmid (TP) and Tat/tyrosinase plasmid/elastic cationic niosomes (TPE), Tat (T) was incubated with tyrosinase plasmid (P) at a T/P ratio of 0.5:1 w/w at room temperature for 30 min. The TP obtained was further incubated with E at the same condition as of PE resulting in the TPE.
Preparation of P, TP, PE and TPE gel
Gel base was prepared by dispersing 0.6 g Carbopol 980 in 36.6 g distilled water with continuous stirring at 25 ± 2 °C for 1 h. The 0.3 g conc. paraben (2% propyl paraben and 18% methyl paraben in propylene glycol) was mixed with 1.0 g triethanolamine, added to the Carbopol 980 dispersion and mixed until a clear gel was obtained. The P, TP, PE or TPE solution was added into the gel base with the final concentration of 30 µg tyrosinase plasmid per 1 g of gel. For transmission electron microscopy, gel containing P, TP, PE or TPE was dissolved in 500 µl of distilled water. A drop of the dissolved gel was applied on a 300-mesh formvar copper grid and allowed to adhere for 10 min. The remaining dispersion was removed and a drop of 2% ammonium molybdate was added for 4 min. The remaining solution was then removed and the grid was air-dried overnight. The sample was examined with a transmission electron microscope (TEM, Philips Tecnai™ 10, FEI Company, Eindhoven, the Netherlands) with 80 kV acceleration voltage, objective diaphragm 4 (20 µm) and 100 µm condenser aperture.
Vesicular size and zeta potential
Vesicular size and zeta potential of TP, PE and TPE solution and gel formulations were determined by the dynamic light scattering technique using Zetasizer Nano ZS (Malvern Instrument, Malvern, UK), with DTSv5.0 software (Malvern Instrument, Malvern, UK). All samples were diluted for 30 folds with freshly distilled water prior to both measurements. The measurement was performed at 25 °C for five individual runs. The medium used in these measurements was water, which has the viscosity, refractive index and dielectric constant of 0.8872 cP, 1.330 and 78.5, respectively.
Stability study
Five milliliters of P, TP, PE or TPE solution and 5 g of P, TP, PE or TPE gel was transferred to a clear glass-vial and kept at 4 ± 2, 25 ± 2 and 45 ± 2 °C in a dark chamber for three months. Samples were withdrawn at the predetermined time intervals (initial, one, two and three months). The viscosity of the gel formulations was determined by using the rotational viscometer (VR 3000 model, Myr, Tarragona, Spain). The experiment was performed at 25 ± 2 °C. The rheology behavior of each gel formulation was evaluated by a graph plotted between shear rate and shear stress. The plasmid was extracted from P and TPE by mixing 100 µl of PE or TPE with 100 µl of phenol:chloroform:isoamylalcohol (P:C:IAA, 25:24:1), vortex for 1 min and centrifuged at 13 680g at 4 °C for 10 min. The TP or extracted TPE containing 2.5 µg of DNA were separately mixed with 20 µl of trypsin solution (5 µg of trypsin in 50 mM HEPES pH 7.4) and incubated at room temperature (25 ± 2 °C) for 24 h to hydrolyze the T from TP or TPE. Trypsin can hydrolyze the Tat peptide which cleaves the peptide chains mainly at the carboxyl side of the amino acids lysine or arginine, except when either is followed by proline (Adami et al., Citation1998). This hydrolyzation resulted in the separation of the Tat peptide from the TP. The remaining plasmid was analyzed in 1% agarose gel electrophoresis at 100 V for 40 min and determined the plasmid band by gel documentation (Universal Hood, BioRad Laboratory, Milan, Italy) with Quantity One program analysis. Quantitative determination of the remaining plasmid was performed using Quant-iTTM dsDNA BR assay kit (Invitrogen, Karlsruhe, Germany).
Transdermal absorption by vertical Franz diffusion cell
Transdermal absorption through rat skin of P, TP, PE and TPE in solution and gel formulations were performed in triplicate using vertical Franz diffusion cells. Sixteen male Sprague–Dawley rats (150–200 g and 10–12 weeks old) obtained from National Laboratory Animal Centre, Mahidol University, Thailand, were used. The abdominal skin of anesthetized rat was shaved and excised. The subcutaneous fat was removed using a scalpel blade and mounted on the receiving chamber with the stratum corneum (SC) side facing upward. The area between the donor and the receiving chamber of the diffusion cell was 2.46 cm2. The receiving chamber was filled with 13 ml of phosphate-buffered saline (PBS, pH 7.0), controlled at 37 ± 2 °C and stirred with a small magnetic bar at 100 rpm throughout the experiment. One milliliter of the solution or 1 g of gel formulation (containing 30 µg of tyrosinase plasmid) was added into the donor chamber and covered with the parafilm. The experiments were stopped at 30 min, 1, 3 and 6 h. The skin were removed and swirled in 100 ml of distilled water of at least 1 min for three times. The receiving solution was collected, lyophilized and analyzed for P by gel electrophoresis and gel documentation. The P in SC was assayed by the stripping method using a Scotch Magic™ tape (3M, St. Paul, MN; 1 cm × 1 cm) (Dutkiewicz et al., Citation2000) and put into a glass bottle containing 3 ml of PBS. The stripped skin was cut into small pieces and put into a glass bottle together with 3 ml of PBS. The P contents in the stripped tapes and the viable epidermis and dermis (VED) were extracted by adding 3 ml of P:C:IAA (25:24:1), vortexed and centrifuged at 8780g, 4 °C for 10 min. The aqueous phase was collected and assayed for the P contents. For TP or TPE, the P in various parts of skin was assayed by mixing with 20 µl of trypsin solution (5 µg of trypsin in 50 mM HEPES pH 7.4) and incubated at room temperature (25 ± 2 °C) for 24 h to hydrolyze the T from the TP or TPE, respectively. The P concentration was determined in 1% agarose gel electrophoresis using 10 µl of the sample and run at 100 V for 40 min. The plasmid band density was determined by gel documentation (ImageMaster® VDS, Pharmacia Biotech, Freiburg, Germany) and the P concentration was calculated from the calibration curve of the plot between the band densities and tyrosinase plasmid concentrations.
Results and discussion
Vesicular size and zeta potential of P, TP, PE and TPE solution and gel formulations
Physical appearances of all gel formulations have been observed. The gel base was clear and the gel appearance was not changed when the P and TP solutions were incorporated, while the transparency of the gel was decreased when the PE and TPE complexes were added. The TEM images of the PE and TPE in gel formulation are demonstrated in , respectively. The nanovesicles were observed in irregular shape with the vesicular size of about 100–300 nm. The vesicular sizes and zeta potential values of P, E, TP, PE and TPE solution and gel formulations are shown in . TP, PE and TPE solutions exhibited larger vesicular sizes (206.4 ± 10.9, 165.0 ± 2.8 and 123.5 ±8.0 nm, respectively) than the E solution (100.2 ± 0.9 nm). The zeta potential values of P and TP solutions were −38.6 ± 1.4 and −9.7 ± 1.9 mV, respectively, whereas the E, PE and TPE solutions demonstrated the positively charged of 42.6 ± 5.0, 37.9 ± 1.4 and 32.5 ± 7.2 mV, respectively. Since the negatively charged plasmid could be located both inside the aqueous phase between the vesicular bilayers and adsorbed outside the vesicular membrane of the elastic cationic niosomes, the charge repulsion between the plasmid molecules might increase the vesicular size (Bouwstra & Honeywell-Nguyen, 2002; Bouwstra et al., Citation2003). The plasmid might also neutralize the positive charges of the vesicular surface resulting in lowering of the zeta potential of the PE and TPE. The gel formulations demonstrated larger vesicular size and lower zeta potential values than the solution formulations. This might be due to the network structure of the Carbopol gel that may interfere with the light scattering intensity resulting in the vesicular size in micrometer range (Manosroi et al., Citation2012). Since Carbopol is a polymer of the acrylic acid cross-linked with the polyalkenyl ethers or divinyl glycol providing carboxyl groups by the acrylic acid backbone (Avinash et al., Citation2006), the negative charge of the carboxyl groups in the Carbopol polymer may neutralize the positive charges of the cationic niosomes resulting in less negative zeta potential values of PE and TPE gel (−44.1 ± 1.1 and −44.3 ± 2.3 mV, respectively) than the P and TP gel (−62.2 ± 2.4 and −51.9 ± 3.3 mV, respectively). The charge neutralization may be beneficial for the loaded cationic niosomes in Carbopol gel to have better physical stability (Jo et al., Citation2004).
Figure 2. TEM images of the PE (A) and TPE (B) in the gel formulations at the magnification of ×20 000.
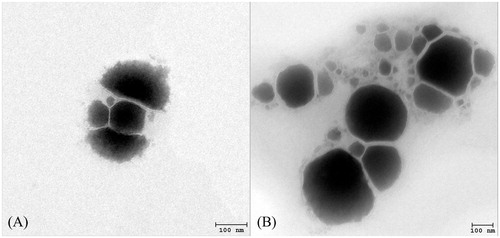
Table 1. Viscosity values (cP) of tyrosinase plasmid (pAH7/Tyr, P), Tat/tyrosinase plasmid complexes (TP), tyrosinase plasmid-loaded elastic cationic niosomes (PE) and Tat/tyrosinase plasmid/elastic cationic niosomes complexes (TPE) gel formulations.
Stability study
The viscosity and rheology of the gel containing either P, TP, PE or TPE are shown in and , respectively. All gel formulations exhibited the Non-Newtonian, pseudoplastic behavior. presents the increases in vesicular size observed in almost all gel formulations at all temperatures after three months except in PE and TPE at 4 ± 2 °C. For the solution formulations, only E, PE and TPE exhibited the stable dispersion, whereas the P and TP solutions were unstable when kept at 45 ± 2 °C for three months. The size enlargement of the pAH7/Tyr loaded nanovesicles after three months of storage might be due to the self-assembly between the vesicular surface. Based on freeze-fracture electron micrographs and X-ray diffraction studies, it was suggested that DNA is sandwiched between many nanovesicles (Sternberg et al., Citation1994; Radler et al., Citation1997). This structure was in arrangement with the increase of vesicular size (Almofti et al., Citation2003). Moreover, the negatively charged DNA would neutralize cationic nanovesicles, resulting in aggregation and continuous fusion with time. All gel formulations demonstrated the zeta potential values within the stable dispersion range (out of ±30 mV) at all temperatures after three months of storage. The percentages remaining of the plasmid in various solution and gel formulations kept at 4 ± 2, 25 ± 2 and 45 ± 2 °C for three months are shown in . Comparing with the initial concentration (100%), significant (p < 0.05) decreases in the percentages remaining of the plasmid were observed from the P and TP solutions kept at all temperatures, whereas the PE and TPE solutions kept at 4 ± 2 °C and 25 ± 2 °C for one month showed slight decreases in the percentages remaining of the plasmid. The highest percentages remaining of the plasmid in solution and gel formulations were observed at 4 ± 2 °C followed by 25 ± 2 °C and 45 ± 2 °C. At the first and second month, the remaining of the plasmid from PE and TPE solutions and gel kept at all temperatures were ranging from 67.60–97.10% to 61.70–97.50% (solution); and 51.52–77.65% to 51.25–74.81% (gel), respectively, which were higher than the remaining plasmid from P and TP solution and gel formulations. In the third month, the plasmid was still observed from all solution and gel formulations kept at 4 ± 2 and 25 ± 2 °C of about 48.00–65.20% and 27.40–51.10% (solution); and 12.34–38.31% and 8.63–36.10% (gel), respectively, whereas the plasmid in the gel formulations kept at 45 ± 2 °C was almost degraded. These results indicated that the loading of the tyrosinase plasmid in the elastic cationic niosomes in the form of free plasmid or TP could enhance the thermal stability of the loaded plasmid since the vesicular membrane might act as a shield to protect the loaded plasmid from thermal and stress of the environment (Varshosaz et al., Citation2003).
Figure 3. Rheology behavior of (A) gel base, (B) E gel, (C) P gel, (D) TP gel, (E) PE gel and (F) TPE gel.
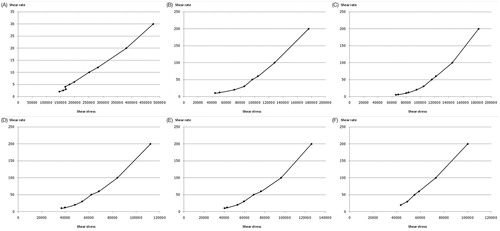
Figure 4. Percentages remaining of the human tyrosinase plasmid (pAH7/Tyr) in free plasmid (P), Tat/tyrosinase plasmid (TP), tyrosinase plasmid-loaded elastic cationic niosomes (PE) and Tat/tyrosinase plasmid/elastic cationic niosomes (TPE) solution and gel formulations kept at 4 ± 2 °C, 25 ± 2 °C and 45 ± 2 °C for 3 months. The * indicates significant (p < 0.05) difference of the percentages remaining of the plasmid compared to the initial (100%).
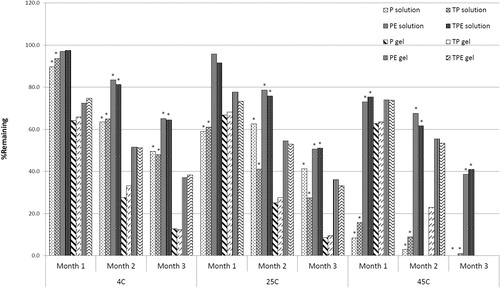
Table 2. Vesicular sizes and zeta potential values of tyrosinase plasmid (pAH7/Tyr, P), Tat peptide (T), blank elastic cationic niosomes (E), Tat/tyrosinase complexes (TP), tyrosinase plasmid loaded elastic cationic niosomes (PE) and Tat/tyrosinase plasmid/elastic cationic niosomes complexes (TPE) at initial and at 4 ± 2 °C, 25 ± 2 °C and 45 ± 2 °C after three months of storage.
Transdermal absorption through rat skin
Transdermal absorption through rat skin of P from free P, TP, PE and TPE were investigated by vertical Franz diffusion cell for 6 h. In solution formulations, P was not observed from all formulations in the SC layer, whereas P from all samples was observed in VED at 0.5, 1, 3 and 6 h. Only P from PE and TPE was found in the receiving solution at 3 and 6 h (data not shown). The highest cumulative amount and flux of the P in VED were observed from the TPE at 6 h of 0.31 ± 0.04 µg/cm2 and 1.86 ± 0.24 µg/cm2/h, respectively (), which was 2.38 folds of P. TP and PE demonstrated the cumulative amount and flux in VED of 1.38 and 2.23 folds of P, respectively. The maximum cumulative amounts and fluxes of the P from PE and TPE in the receiving solution were observed at 6 h of 0.07 ± 0.01 µg/cm2 and 0.40 ± 0.08 µg/cm2/h (PE) and 0.10 ± 0.01 µg/cm2 and 0.60 ± 0.06 µg/cm2/h (TPE), respectively (), whereas the P from free P and TP could not be observed. These might be due to the positive charge of the PE and TPE, which could be attached to the negatively charged of the skin via electrostatic interaction. In contrast, the negatively charged P and TP could not pass through the skin due o the charge repulsion effect between the negative charge of the P or TP and the negative charge of the skin (Prausnitz et al., Citation1993). Moreover, the high transdermal absorption through rat skin of the PE and TPE might be due to the elasticity of the elastic cationic niosomes that could squeeze through the small pore in the stratum corneum, which is smaller than their vesicular size into the deeper layer of the skin (Choi & Maibach, Citation2005). Furthermore, the ethanol composition of the elastic niosomal formulation might play an important role in the enhancement of the transdermal absorption of the loaded plasmid. Mechanisms of ethanol on skin permeation enhancement have been reported, including an increase of drug diffusion through the lipid pathway of the skin (Hatanaka et al., Citation1993), the reduction of lipid polar head interactions or disordering liquid–crystalline phases within the membrane (Knutson et al., Citation1990) and an increase of drug solubility in the SC (Megrab et al., Citation1995). The TPE which exhibited lower positive charge than the PE showed higher cumulative amounts and fluxes of the P in both VED and receiving solution. This result indicating that the T peptide played an important role over the charge interaction between the TPE and the skin. This result was correlated to previous studies which showed that the interaction of the CPPs including Tat peptide with skin lipids may be the main transport across the SC, since this interaction may destabilize the SC resulting in an increase in the membrane permeability (Rothbard et al., Citation2000). Another suggested mechanism of transport was via the tight junctions which allowed penetration into the viable skin layers (Lopes et al., Citation2008). Kang et al. (Citation2010) studied the in vitro and in vivo skin penetration effect of Tat-coated elastic liposomes, they found that the Tat peptide could increase the flux of the liposomes by about 20%.
Figure 5. Cumulative amounts (A) and fluxes (B) of the pAH7/Tyr in viable epidermis and dermis of free tyrosinase plasmid (P), Tat/tyrosinase plasmid (TP), tyrosinase plasmid-loaded elastic cationic niosomes (PE) and Tat/tyrosinase plasmid/elastic cationic niosomes (TPE) solution formulations.
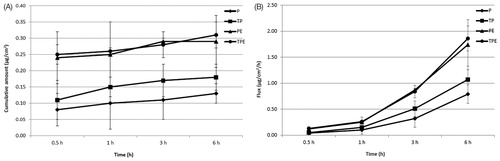
Figure 6. Cumulative amounts (A) and fluxes (B) of the pAH7/Tyr in the receiving solution of free tyrosinase plasmid (P), Tat/tyrosinase plasmid (TP), tyrosinase plasmid-loaded elastic cationic niosomes (PE) and Tat/tyrosinase plasmid/elastic cationic niosomes (TPE) solution formulations.
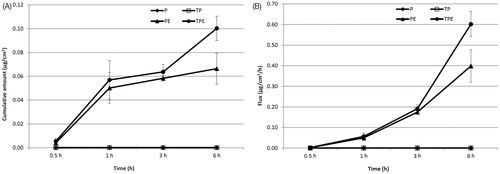
For the gel formulations, the cumulative amounts and fluxes of the plasmid in VED are shown in . The highest cumulative amount and flux of the plasmid in VED was observed from the TPE gel at 6 h of 4.29 ± 0.40 µg/cm2 and 25.73 ± 2.40 µg/cm2/h, respectively, which was 5.39 folds of P. For TP and PE gel formulations, the cumulative amount and flux of these formulations in VED were 0.88 and 2.12 folds of P, respectively. Only plasmid from PE and TPE gel was found in the receiving solution with the highest cumulative amount and flux from the TPE gel at 6 h of 1.02 ± 0.05 µg/cm2 and 6.13 ± 0.28 µg/cm2/h, respectively (). Higher cumulative amounts and fluxes of the plasmid in various parts of skin of the gel formulations over the solution formulations indicated that the gel structure can promote the penetration of the plasmid DNA owing to the occlusion effects from the gel formulation, which can enhance skin hydration and consequently increase the absorption and penetration of the plasmid DNA across the rat skin (Manosroi et al., Citation2010b). The results of this study indicated the potent enhancement of transdermal absorption through rat skin and the thermal stability of the human tyrosinase plasmid (pAH7/Tyr) using the Tat peptide incorporated elastic cationic niosomes both in solution and gel formulations.
Conclusion
The transdermal absorption through rat skin and thermal stability of the human tyrosinase plasmid (pAH7/Tyr) could be potently enhanced using Tat peptide and an entrapment in elastic cationic niosomes. The TPE solution and gel formulations demonstrated a higher cumulative amount and flux of the P in the VED and receiving solution and also percentage remaining of the plasmid than the P, TP and PE solution and gel formulations kept at 4 ± 2, 25 ± 2 and 45 ± 2 °C for three months. From the results of this study, the application of the TPE as a transdermal gene delivery system was anticipated.
Declaration of interest
The authors report no declarations of interest.
Acknowledgements
This work was supported by the Thailand Research Fund (TRF) under the RGJ-PhD program, Natural Products Research and Development Center (NPRDC), Science and Technology Research Institute (STRI), Chiang Mai University, Chiang Mai 50200, Thailand. The authors gratefully acknowledge the supporting of Boehringer Ingelheim and University of Tübingen, Germany, for providing tyrosinase (pAH7/Tyr) plasmid used in this study.
References
- Adami RC, Collard WT, Gupta SA, et al. (1998). Stability of peptide-condensed plasmid DNA formulations. J Pharm Sci 87:678–83
- Almofti MR, Harashima H, Shinohara Y, et al. (2003). Cationic liposome-mediated gene delivery: biophysical study and mechanism of internalization. Arch Biochem Biophys 410:246–53
- Avinash HH, Thorat YS, Kastur PV. (2006). Carbopol and its pharmaceutical significance: a review. Pharmaceut Rev 4:1–20
- Bouwstra JA, Honeywell-Nguyen PL. (2002). Skin structure and mode of action of vesicles. Adv Drug Deliv Rev 54:41–55
- Bouwstra JA, Honeywell-Nguyen PL, Gooris GS, Ponec M. (2003). Structure of the skin barrier and its modulation by vesicular formulations. Progr Lipid Res 42:1–36
- Choi MJ, Maibach HI. (2005). Elastic vesicles as topical/transdermal drug delivery systems. Int J Cosmet Sci 27:211–21
- Desai P, Patlolla RR, Singh M. (2010). Interaction of nanoparticles and cell-penetrating peptides with skin for transdermal drug delivery. Mol Membr Biol 27:247–59
- Dutkiewicz R, Albert DM, Levin LA. (2000). Effects of latanoprost on tyrosinase activity and mitotic index of cultured melanoma lines. Exp Eye Res 70:563–9
- Eum WS, Jang SH, Kim DW, et al. (2005). Enhanced transduction of Cu, Zn-superoxide dismutase with HIV-1 Tat protein transduction domains at both termini. Mol Cells 19:191–7
- Hatanaka T, Shimoyama M, Sugibayashi K, Morimoto Y. (1993). Effect of vehicles on the skin permeability of drugs: polyethylene glycol 400-water and ethanol-water binary solvents. J Control Release 23:247–60
- Kang MJ, Eum JY, Jeong MS, et al. (2010). Facilitated skin permeation of oregonin by elastic liposomal formulations and suppression of atopic dermatitis in NC/Nga mice. Biol Pharm Bull 33:100–6
- Kikuchi H, Suzuki N, Ebihara K, et al. (1999). Gene delivery using liposome technology. J Control Release 62:269–77
- Kim DW, Eum WS, Jang SH, et al. (2003). Ginsenosides enhance the transduction of tat-superoxide dismutase into mammalian cells and skin. Mol Cells 16:402–6
- Knutson K, Krill SL, Zhang J. (1990). Solvent-mediated alterations of the stratum corneum. J Control Release 11:93–103
- Jo V, Krassimira Y, Annick L. (2004). Influence of the homogenization procedure on the physicochemical properties of PLGA nanoparticles. Chem Pharm Bull (Tokyo) 50:1273–9
- Lim JM, Chang MY, Park SG, et al. (2003). Penetration enhancement in mouse skin and lipolysis in adipocytes by TAT-GKH, a new cosmetic ingredient. J Cosmet Sci 54:483–91
- Lopes LB, Furnish E, Komalavilas P, et al. (2008). Enhanced skin penetration of P20 phosphopeptide using protein transduction domains. Eur J Pharm Biopharm 68:441–5
- Manosroi A, Jantrawut P, Manosroi J. (2008). Anti-inflammatory activity of gel containing novel elastic niosomes entrapped with diclofenac diethylammonium. Int J Pharm 360:156–63
- Manosroi A, Khanrin P, Lohcharoenkal W, et al. (2010a). Transdermal absorption ehhancement through rat skin of gallidermin loaded in niosomes. Int J Pharm 392:304–10
- Manosroi J, Khositsuntiwong N, Götz F, et al. (2010b). Enhancement of transdermal absorption, gene expression and stability of tyrosinase plasmid (pMEL34) loaded elastic cationic niosomes: potential application in vitiligo treatment. J Pharm Sci 99:3533–41
- Manosroi A, Ruksiriwanich W, Abe M, et al. (2012). Transfolliculer enhancement of gel containing cationic niosomes loaded with unsaturated fatty acids in rice (Oryza sativa) bran semi-purifies fraction. Eur J Pharm Biopharm 81:303–13
- Megrab NA, Williams AC, Barry BW. (1995). Oestradiol permeation across human skin, silastic, and snake skin membrenes: the effects of ethanol/water co-solvent systems. Int J Pharm 116:101–12
- Park J, Ryu J, Jin LH, et al. (2002). 9-Polylysine protein transduction domain: enhanced penetration efficiency of superoxide dismutase into mammalian cells and skin. Mol Cells 13:202–8
- Patel D, Patel N, Parmar M, Kaur N. (2011). Transdermal drug delivery system: review. Int J Biopharm Toxicol Res 1:61–80
- Prausnitz MR, Bose VG, Langer R, Weaver JC. (1993). Electroporation of mammalian skin: a mechanism to enhance transdermal drug delivery. Proc Natl Acad Sci USA 90:10504–8
- Prausnitz MR, Mitragotri S, Langer R. (2004). Current status and future potential of transdermal drug delivery. Nat Rev Drug Discov 3:115–24
- Radler JO, Koltover I, Salditt T, Safinya CR. (1997). Structure of DNA-cationic liposome complexes: DNA intercalation in multilamellar membranes in distinct interhelical packing regimes. Science 275:810–14
- Rothbard JB, Garlington S, Lin Q, et al. (2000). Conjugation of arginine oligomers to cyclosporine A facilitates topical delivery and inhibition of inflammation. Nat Med 6:1253–7
- Sternberg B, Sorgi FL, Huang L. (1994). New structures in complex formation between DNA and cationic liposomes visualized by freeze-fracture electron microscopy. FEBS Lett 356:361–6
- Varshosaz J, Pardakhty A, Hajhashemi VI, Najafabadi AR. (2003). Development and physical characterization of sorbitan monoester niosomes for insulin oral delivery. Drug Deliv 10:251–62