Abstract
Lactic acidosis is a serious, metabolic complication that may occur due to metformin hydrochloride (MH) accumulation during the treatment of diabetes mellitus. The aim of this study is to enhance the bioavailability of MH by oral route. Span 40 and cholesterol were used for the preparation of MH-loaded niosomes by the reverse phase evaporation technique. Dicetyl phosphate (DCP) and 1,2-dioleoyl-3-trimethylammonium-propane chloride salt (DOTAP) were used to obtain negatively and positively charged vesicles, respectively. The mean particle size ranged from 223.5 to 384.6 nm and the MH-loaded niosomes’ surface was negatively charged in the absence of charge inducing agents (−16.6 ± 1.4 mV) and also with DCP (−26.9 ± 1.0 mV), while it was positively charged (+8.7 ± 1.2 mV) with DOTAP. High entrapment efficiency was observed in all the formulations. MH-loaded niosomes were found to effectively sustain the release of drug, particularly with positively charged niosomes. The bioavailability of MH-loaded niosomes was assessed by measuring the serum values of glucose and metformin in the different studied Wistar rats groups. The pharmacokinetic data of MH-loaded niosomal preparation showed a significant prolongation and increased intensity of hypoglycemic effect more than that observed for free MH solution. Area above the blood glucose levels–time curve (AAC), maximum hypoglycemic response and time of maximum response (Tmax) were significantly higher (p < 0.001) when MH was administered in niosomal form compared to free drug solution. It could be concluded that MH-loaded niosome is promising extended-release preparation with better hypoglycemic efficiency.
Introduction
Metformin hydrochloride (MH) has become the standard of care for type II diabetes, particularly in overweight and it has been shown to reduce the cardiovascular disease within this population. MH is a small molecule (150 kDa) with 50% oral bioavailability. It does not undergo hepatic metabolism and the main route of elimination is renal tubular secretion. MH is not bound to proteins and its apparent volume of distribution is usually reported to be higher than 3 l/kg (63–646 l in total) attesting to the predominance of the intracellular location (UK Prospective Diabetes Study group, Citation1998).
Metformin-associated lactic acidosis is a rare but a classic side effect of metformin (Lebacq & Tirzmalis, Citation1972). It is potentially fatal complication that can occur due to MH accumulation. If it occurs, case fatality rate is ∼50% (Perrone et al., Citation2011). It is also known that metformin impairs lactate clearance of the liver through the inhibition of complex I of the mitochondrial respiratory chain (El-Mir et al., Citation2000; Owen et al., Citation2000). So, lactic acidosis associated with the use of MH is predominantly due to the lack of lactate’s clearance rather than to an increased production. The low bioavailability and short half-life of MH make the necessity for the development of extended-release tablets to minimize frequency and drug accumulation, minimizing side effects and augmenting patient compliance (Vidon et al., Citation1988; Hermann & Melander, Citation1992; Scheen, Citation1996; Corti et al., Citation2008).
Nanotechnology appears as one of the most promising areas in the field of pharmaceutics (Devalapally et al., Citation2007). Many varieties of nanoparticles are available such as different polymeric and metal nanoparticles, liposomes, niosomes, solid lipid particles, micelles, quantum dots, dendrimers, microcapsules, cells, cell ghosts, lipoproteins and different nano-assemblies (West & Halas, Citation2000).
Niosomes are vesicles composed of non-ionic surfactants, which are biodegradable, relatively nontoxic, more stable than liposomes and inexpensive. It is an alternative to liposomes. Niosomal vesicles can be small unilamellar, multilamellar or large unilamellar (Karim et al., Citation2010). Niosomes are capable of encapsulating large quantities of material in a relatively small volume of vesicles (Khandare et al., Citation1994).
Recently, niosomes have been studied as drug delivery system to provide a better oral bioavailability considering stability and high penetration property through biological membrane (Akhilesh et al., Citation2011).
Niosome formation requires the presence of a particular class of charge inducing agents to prevent vesicle aggregation, hence improves stability (Essa, Citation2010). In addition, charged vesicles may achieve more therapeutic efficiency than neutral vesicles, due to its ability to target certain cells. For example, cationic niosomes are used successfully as drug delivery systems for improving potency of oligonucleotides in vivo (Huang et al., Citation2008).
The aim of this study is to produce more effective form of MH preparation with more prolonged effect by formulation of MH-loaded niosomes targeting for maintaining effective plasma drug concentrations.
Materials and methods
Materials
MH was kindly supplied by Saudi Pharmaceutical Industries & Medical Appliances Corporation (SPIMACO), Al-Qassim City, KSA; cholesterol (Chol), dicetylphosphate (DCP), Span 40, Streptozotocin (STZ; C8H15N3O7, molecular weight: 265.22 g/mol) and nicotinamide (C6H6N2O, molecular weight: 122.12 g/mol) were purchased from Sigma Chemical Co., St Louis, MO, Lipid DOTAP (1,2-dioleoyl-3-trimethylammonium-propane, chloride salt) was kindly supplied by Lipoid AG, Steinhausen, Switzerland.
Animals
Adult male Wistar rats (Rattus norvegicus), weighing about 120–130 g, were obtained from College of Veterinary Medicine and Animal Resources, King Faisal University, Al-Hufof, KSA. Animals were housed in suitable cages and acclimatized to laboratory conditions for a period of 1 week before the commencement of the experiments. Rats were fed standard rodent food pellets (ARASCO, Riyadh, KSA) and distilled water. All animals were humanely treated and the study design was approved by the King Faisal University Research Ethics Committee (22 May 2011, No. 245 DSR).
Preparation of metformin hydrochloride-loaded niosomes
Reverse phase evaporation (REV) technique with slight modifications was used to prepare MH-loaded niosomes. MH niosomes was prepared from a total of 0.2 mM of film forming constituents consisting of Span 40, Chol with or without DOTAP or DCP in molar ratios shown in . Blank niosomes without MH was prepared using Span 40 and Chol in a molar ratio 150:40.
Table 1. MH niosomal formulations expressed as molar ratios of lipid components.
Lipid solution (Chol and Span 40 with or without charge inducing agents DOTAP or DCP dissolved in 5 ml of diethyl ether and 5 ml chloroform) was emulsified with the aqueous phase containing drug (2 ml) using a Microson XL-2000 (Misonix Inc., Farmingdale, NY) probe sonicator at 4 °C for 5 min. The clear gel formed is further sonicated after the addition of a small amount (1 ml) of phosphate-buffered saline (PBS; pH 6.8). The organic solvents were evaporated by swirling at room temperature to obtain a thick gel. The gel was collapsed by the addition of 2 ml PBS with simultaneous eddying and then evaporated in a rotary evaporator at 40 °C under low pressure for 10 min, then heated on a water bath at 60 °C for 10 min to yield niosomal vesicles (Raja Naresh et al., Citation1994; Namdeo & Jain, Citation1999).
Characterization of niosomes
Entrapment efficiency
The niosome formulations were first dialyzed exhaustively using dialysis tubing (with a molecular weight cutoff 12 000–14 000 Da) against PBS. To 0.1 ml of the dialyzed dispersion, 1 ml of n-propranolol was added and kept aside for 5 min for complete disruption of niosomes. The resulting solution was then diluted with 50% n-propranolol in PBS and the absorbance was measured at 232.2 nm. Percent drug entrapped was calculated by the following formula (Vyas et al., Citation1998; Sammour et al., Citation2004):
Particle size and zeta potential
Niosomal size distribution and zeta potential were determined using photon correlation spectroscopy (Zetasizer Nano ZS, ZEN3600; Malvern Instruments, Malvern, UK). The size distribution analysis was performed at a scattering angle of 90° and at a temperature of 25 °C, whereas zeta potential was measured using a disposable zeta cuvette. For each sample, the mean diameter/zeta potential ± standard deviation of six determinations was calculated applying multimodal analysis.
In vitro drug release studies
In vitro drug release studies from the prepared niosomal formulations were carried out at pH 6.8 using a dialysis bag (with a molecular weight cutoff 12 000–14 000 Da). An amount of niosomes equivalent to 100 mg of MH was weighed and filled in the bag which was placed into a beaker containing 100 ml of PBS. The beaker was placed over a magnetic stirrer (50 rpm). The temperature was maintained at 37 ± 1 °C. Aliquots (4 ml) were withdrawn periodically and equal volumes of fresh medium equilibrated at 37 ± 1 °C were replaced. The test was continued for 12 h. The withdrawn samples were then analyzed for drug content spectrophotometrically at 232.2 nm. To check the eventual limiting effects of dialysis membrane on drug release, separate experiment was run with free MH solution in the same PBS.
Morphology
Niosomes were analyzed by negative stain electron microscopy. A drop of the vesicle preparation was applied to a precoated copper grid and the excess was removed with a filter paper. A drop of 2% uranyl acetate solution was then added to the grid and, after 2 min, the excess was removed with a filter paper (Liu & Guo, Citation2007). The stained samples were viewed and photographed with an electron microscope (100 CX, Jeol, Tokyo, Japan).
In vivo anti-hyperglycemic activity
Induction of type 2 diabetes
Type 2 diabetes was induced in overnight fasted rats by STZ (a single intraperitoneal injection of 65 mg/kg body weight) and nicotinamide (a single intraperitoneal injection of 110 mg/kg body weight, 15 min before STZ injection) as previously described (Murugan & Pari, Citation2007). STZ and nicotinamide were dissolved in citrate buffer (pH 4.5) and physiological saline, respectively. Rats with values of blood glucose higher than 200 mg/dl (72 h after diabetes induction) were considered diabetic. This model is characterized by only 40% reduction in β-cell mass, which results in moderate and stable hyperglycemia, glucose intolerance and altered but significant glucose-stimulated insulin secretion.
Experimental design and treatment schedule
Animals were randomly divided into eight groups of six animals each. They were divided as two control groups, one healthy group and one diabetic group, and six drug-test diabetic groups treated with metformin. Blood samples were taken at 0, 1, 2, 4, 6, 8 and 24 h following drug administration. In the healthy group, rats were injected with intraperitoneal saline and given 1.0 ml distilled water orally.
Blood sampling
Animals were subjected to light diethyl ether anesthesia before sacrificing. The blood was collected into clean and dry test-tubes without EDTA to separate serum, which was divided into samples and preserved at −40 °C until used for biochemical analysis.
Measurements
Serum glucose was estimated using glucose oxidase and peroxidase (Trinder, Citation1969). Serum levels of metformin were analyzed by liquid chromatography–mass spectrometry (LCMS).
LCMS assay method
Five hundred microliters of blood was collected and then centrifuged at 9000 rpm for 10 min. To 200 µl of plasma, 200 µl of methanol was added to precipitate the protein. Then, the mixture was eddied for 1 min and centrifuged at 9000 rpm for 10 min. Again, 100 µl supernatant was mixed with 100 µl of mobile phase and 20 µl was injected into the LCMS system for metformin concentration determination. The conditions for LCMS assay were as follows: The LCMS system (Agilent, Germany) consisted of 1200 pump, 1200 MS detector and an auto injector, with Chem data module. Chromatographic separation of active ingredients was achieved using a Zorbax eclipse C18 (150 mm × 4.6 mm i.d, 5 μm) reverse phase analytical column at room temperature (approximately 25 °C). The mobile phase was 95% 20 mM ammonium acetate, pH 6.8 and 5% methanol and before use the solution was filtered through a 0.45 µm Nylon filter. HPLC was performed isocratically at a flow rate of 0.5 ml/min. The eluate was continuously monitored by an MS detector using positive ion mode at m/z 130.2.
Pharmacokinetic and statistical analysis
The pharmacokinetic parameters were calculated, variables of interest included area above the concentration–time curve (AAC), maximum response and time of maximum response (Tmax). The in vivo data were presented as mean ± SEM (standard error of the mean). Statistical analysis was performed with one-way analysis of variance (ANOVA, Tukey’s Multiple Comparison Test) for the significant interrelation between the various groups, using GraphPad Prism version 4.00 (Windows, GraphPad software, San Diego, CA). p Values of <0.05, <0.01 and <0.001 will be considered statistically significant, highly significant and very highly significant, respectively.
Results and discussion
The prepared niosomes were evaluated and the effects of incorporation of DCP or DOTAP on entrapment efficiency, release rate, particle size and zeta potential were studied.
Preparation of metformin hydrochloride-loaded niosomes
The prepared niosomes were selected to include about 25% Chol to give appropriate stability for niosomes bilayer membrane; this accords with previous studies that decided that 10–20% Chol was optimum to get stable vesicle by abolishing the phase transition temperature resulting in stable niosomes avoiding drug leakage (Hao et al., Citation2002; Srinivas et al., Citation2010).
Entrapment efficiency
Entrapment efficiency of MH in different niosomal preparations is presented in . Entrapment efficiency was high in all the formulations which may be attributed to the use of REV technique to prepare niosomes. This technique resulted in high uptake of MH due to the large encapsulated volume in unilamellar vesicles of REV (Namdeo & Jain, Citation1999; Guinedi et al., Citation2005), which leads to more entrapped MH as a hydrophilic drug within the aqueous core.
Table 2. Properties of MH-loaded niosomes.
Also, the use of Span 40 as non-ionic surfactant may be from the factors that led to high entrapment efficiency, this could be attributed to the high HLB values 6.7 which result in reduction in surface free energy (Yoshioka et al., Citation1994; Manconi et al., Citation2002). Moreover, Span 40 is solid at room temperature and exhibit high phase transition temperature (Tc) that allows to form vesicles of large size, and hence high entrapment efficiency.
Negatively charged niosomes showed the highest entrapment efficiency (92.62%) of MH more than neutral (87.78%) and positively charged niosomes (83.36%), respectively. This may be attributed to the formation of a lipophilic moiety localized in the hydrophobic lamella because of the strength of electrostatic attraction between DCP and MH as cationic drug. This is in addition to the amount of MH localized in the aqueous region encapsulated as hydrophilic drug. In contrast, the drug is localized only in the aqueous hydrophilic region of neutral and positively charged niosomes. It has also been observed that the inclusion of DOTAP into MH niosomes relatively decreases entrapment efficiency compared to the formulation without charge-inducing agents, presumably due to the electrostatic repulsion forces between MH as a cationic drug and a cationic head group of DOTAP.
Particle size and zeta potential
Particle size and zeta potential of niosomal formulations were measured (). The particle sizes of all developed niosomes were between 223.5 and 384.6 nm. Negatively charged niosomes showed larger particle size than neutral and positively charged niosomes, respectively.
The inclusion of DCP into MH niosomes produced relatively large particle sizes compared to the formulations without DCP. In contrast, the inclusion of DOTAP into MH niosomes showed much smaller sizes. This could be explained by the similar charge of DCP, Span 40 and Chol head groups that cause electrostatic repulsion between them decreasing membrane curvature, hence increased particle size. Contrary to that, electrostatic attraction occurs between DOTAP, Span 40 and Chol head groups, hence more membrane curvature and smaller particle size. This is in agreement with other studies that reported the effect of charge-inducing agents on vesicle size due to the electrostatic repulsion or attraction forces between the head groups of charge-inducing agents and lipid components in vesicle bilayer (Van Hal et al., Citation1996; Carafa et al., Citation1998; Fang et al., Citation2001; Pardakhty et al., Citation2007; Junyapraset et al., Citation2008).
Blank niosomes showed a negative charge with a zeta potential value of −55.6 ± 7.5 mV. This might be attributed to hydroxyl ions adsorbed on niosomes surface. Whereas, MH-loaded neutral niosomes (MN1) composed of the same molar ratio of Span 40:Chol showed −16.6 ± 1.4 mV. This could be explained by the cationic nature of MH that seems to lead to the reduction of zeta potential by more than 70% (from −55.6 ± 7.5 mV to −16.6 ± 1.4 mV).
The addition of DCP to the formulation increased the negative surface charge of MN2 to −26.9 ± 1.0 mV, while the addition of DOTAP showed a positive average zeta potential of +8.7 ± 1.2 mV for MN3.
In vitro release studies
shows that MH-loaded niosomes slow down the rate of drug release compared with the MH-free drug solution. The efflux of MH from niosomes was biphasic, with an initial faster release which lasts for about 1–3 h, followed by a period of slow but sustained release. This biphasic release pattern of the water soluble drugs seems to be characteristic of the bilayer vesicles. Similar reports exist in the case of liposomes (Betageri & Parsons, Citation1992) and in niosomes (Popli & Nair, Citation1996).
Figure 1. Effect of lipid composition on the release profile of metformin hydrochloride. The data represent the mean ± SD of six determinations.
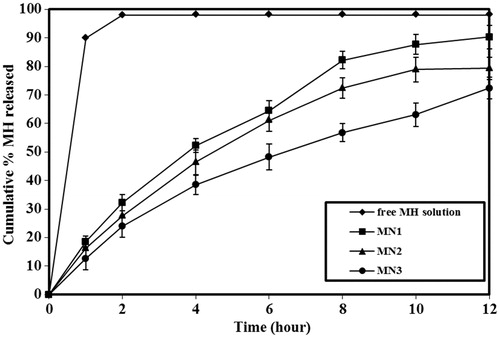
Presence of Span 40 slows the release rate. This may be attributed to the high Tc of Span 40 which led to rigid bilayer (Ladbrooke et al., Citation1968; Papahadjopoulos et al., Citation1973; Yoshioka et al., Citation1994; Namdeo & Jain, Citation1999).
The presence of charge inducing agents caused a further reduction in the amount of drug released. This may be due to the fact that charged lipids serve to tighten the molecular packaging of the vesicle bilayer (Omaima & Ahmed, Citation1997), resulting in decreased drug release from charged niosomes. This result is in accordance with that reported by several authors (Finkelstein & Weissman, Citation1979; Namdeo & Jain, Citation1999).
Morphology
Examination of all batches by electron microscope showed conventional spherical vesicles. shows the negative staining micrograph for niosomes (MN2 and MN3, respectively).
In vivo study
Metformin is insulin sensitizer known to improve glycemic control in type 2 diabetics (Natali & Ferrannin, Citation2006). In vivo evaluation was carried out in diabetic adult male Wistar rats by measuring serum levels of glucose and metformin in the different studied groups after the oral administration of free MH solution or positively charged MH-loaded niosomes (MN3) using the same dose. MN3 formulation was preferred for further in vivo studies due to its in vitro release profile (MH release was less in the initial period) and the fairly good entrapment efficiency (83.36%).
and and and reveal that when free MH solution was administered, a rapid hypoglycemic effect was observed and maximum response of ∼25.21% (52.47 ± 6.3 mg/dl, reduction in blood glucose levels) was at 1 h (Tmax) after oral administration. In the case of MH niosomes, the hypoglycemic effect was slow and reached maximum reduction at 4 h (Tmax) after oral administration and the maximum response was ∼45.89% (95.3 ± 5.9 mg/dl, reduction in blood glucose levels). Drug effect was extended to 6–8 h by loading with niosomes compared with the free drug solution which lasted only for 2–4 h. The area above the blood glucose levels–time curve over 8 h (AAC0–8) after oral administration of niosomes (MN3) was found to be (∼332.5 mgh/dl) approximately twofold higher than the free MH solution (∼155.0 mgh/dl).
Figure 3. Blood glucose concentration after oral administration of free MH solution or MH-loaded niosomes (MN3). The data represent the mean ± SEM of six determinations.
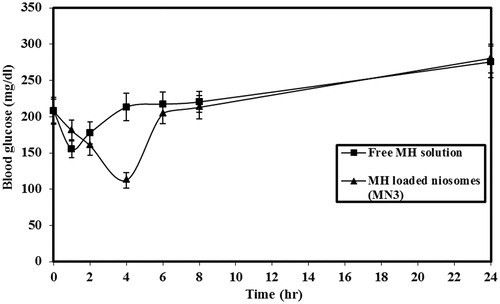
Figure 4. Serum level of metformin hydrochloride after oral administration of free MH solution or MH-loaded niosomes (MN3). The data represent the mean ± SEM of six determinations.
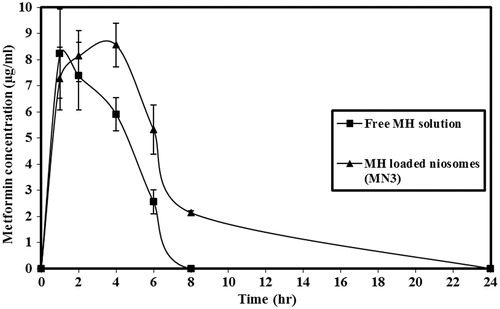
Table 3. Serum levels of glucose and metformin in the different studied groups after oral administration of free MH solution or MH-loaded niosomes (MN3).
Table 4. Pharmacokinetic parameters of blood glucose levels following oral administration of free MH solution or MH-loaded niosomes (MN3).
Statistical analysis of data revealed that oral administration of MH-loaded niosomes (MN3) led to a significant increase in values of AAC0–8, maximum hypoglycemic response and Tmax, compared to the free MH solution (p < 0.001).
Hypoglycemic effect was observed to be higher for MH-loaded niosomes than MH-free solution. This refers to the high penetration property of the niosome encapsulated agents through the biological membranes (Akhilesh et al., Citation2011). The extended hypoglycemic effect produced by positively charged niosomes has been monitored. This effect could be explained due to the presence of lipid bilayer which acts as hydrophobic barrier which leads to slow release of the drug. Moreover, the mucoadhesive properties between positively charged niosomes and negatively charged mucosal surface lead to absorption of MH over longer periods (Bernkop-Schnurch et al., Citation2004).
Conclusion
It can be concluded that the administration of MH-loaded niosomes as a delivery system for oral purposes would be advantageous because a prolonged and improved hypoglycemic effect can be obtained compared to free drug solution using the same dose. This leads to a reduction of the number of doses that should be given to the patients daily as well as expected minimizing the side effect of the drug. Hence, comfortable dosage form can be produced.
Lyophilization of MH niosomal nanosuspension and incorporating it in the tablet form is recommended for further investigations as promising sustained release oral preparation using the same tablet dose on the market.
Declaration of interest
The authors report no conflicts of interest. The authors alone are responsible for the content and writing of this article.
References
- Akhilesh D, Anoop VN, Rao BP. (2011). Formulation and evaluation of gliclazide loaded maltodextrin based proniosomes. Int J Res Pharm Biomed Sci 2:1582–9
- Bernkop-Schnurch A, Guggi D, dan Pinter Y. (2004). Thiolated chitosans: development and in vitro evaluation of a mucoadhesive, permeation enhancing oral drug delivery system. J Control Release 94:177–86
- Betageri GV, Parsons DL. (1992). Drug encapsulation and release from multilamellar and unilamellar liposomes. Int J Pharm 81:235–41
- Carafa M, Santucci E, Alhaique F, et al. (1998). Preparation and properties of new unilamellar non-ionic/ionic surfactant vesicles. Int J Pharm 160:51–9
- Corti G, Cirri M, Maestrelli F, et al. (2008). Sustained-release matrix tablets of metformin hydrochloride in combination with triacetyl-b-cyclodextrin. Eur J Pharm Biopharm 68:303–9
- Devalapally H, Chakilam A, Amiji MM. (2007). Role of nanotechnology in pharmaceutical product development. J Pharm Sci 96:2547–55
- El-Mir MY, Nogueira V, Fontaine E, et al. (2000). Dimethylbiguanide inhibits cell respiration via an indirect effect targeted on the respiratory chain complex I. J Biol Chem 275:223–8
- Essa EA. (2010). Effect of formulation and processing variables on the particle size of sorbitan monopalmitate niosomes. Asian J Pharm 4:227–33
- Fang J, Hong CT, Chiu W, Wang YY. (2001). Effect of liposomes and niosomes on skin permeation of enoxacin. Int J Pharm 219:61–72
- Finkelstein MC, Weissman G. (1979). Enzyme replacement via liposomes. Int J Pharm 588:202–16
- Guinedi AS, Mortada ND, Mansour S, Hathout RM. (2005). Preparation and evaluation of reverse-phase evaporation and multilamellar niosomes as ophthalmic carriers of acetazolamide. Int J Pharm 306:71–82
- Hao Y, Zhao F, Li N, et al. (2002). Studies on a high encapsulation of colchicine by niosome system. Int J Pharm 244:73–80
- Hermann LS, Melander A. (1992). Biguanides: basic aspects and clinical use. In: Alberti KGMM, De Fronzo RA, Keen H, eds. International textbook of diabetes mellitus. New York: Wiley, 772–95
- Huang Y, Chen J, Chen X, et al. (2008). PEGylated synthetic surfactant vesicles (Niosomes): novel carriers for oligonucleotides. J Mater Sci: Mater Med 19:607–14
- Junyapraset VB, Teeranachaideekul V, Supapem T. (2008). Effect of charged and non-ionic membrane additives on physicochemical properties and stability of niosomes. AAPS PharmSci Tech 9:851–9
- Karim KM, Mandal AS, Biswas N, et al. (2010). Niosome: a future of targeted drug delivery systems. J Advan Pharm Tech Res 1:374–80
- Khandare JN, Madhavi G, Tamhankar BM. (1994). Niosomes novel drug delivery system. East Pharm 37:61–4
- Ladbrooke BD, Williams RM, Chapman D. (1968). Studies on lecithin-cholesterol-water interaction by differential scanning calorimetry and X-ray diffraction. Biochim Biophys Acta 150:333–40
- Lebacq EG, Tirzmalis A. (1972). Metformin and lactic acidosis. Lancet 1:314–15
- Liu T, Guo R. (2007). Investigation of PEG 6000/Tween 80/Span 80/H2O niosome microstructure. Colloid Polym Sci 285:711–13
- Manconi M, Valenti D, Loy G, Fadda LM. (2002). Niosomes as carriers for tretinoin. I. Preparation and properties. Int J Pharm 234:237–48
- Murugan P, Pari L. (2007). Protective role of tetrahydrocurcumin on changes in the fatty acid composition in streptozotocin-nicotinamide induced type 2 diabetic rats. J Appl Biomed 5:31–8
- Namdeo A, Jain NK. (1999). Niosomal delivery of 5-flurouracil. J Micro Encapsul 16:731–40
- Natali A, Ferrannini E. (2006). Effects of metformin and thiazolidinediones on suppression of hepatic glucose production and stimulation of glucose uptake in type 2 diabetes: a systematic review. Diabetologia 49:434–41
- Omaima NE, Ahmed HH. (1997). Formulation and evaluation of acetazolamide liposomes as an ocular drug delivery system. Int J Pharm 158:121–7
- Owen MR, Doran E, Halestrap AP. (2000). Evidence that metformin exerts its anti-diabetic effects through inhibition of complex 1 of the mitochondrial respiratory chain. Biochem J 348:607–14
- Papahadjopoulos D, Jacobson K, Nir S, Isac T. (1973). Phase transitions in phospholipid vesicles: fluorescence polarization and permeability properties concerning the effect of temperature and cholesterol. Biochim Biophys Acta 311:330–48
- Pardakhty A, Varshosaz J, Rouholamini A. (2007). In vitro study of polyoxyethylene alkyl ether niosomes for delivery of insulin. Int J Pharm 328:130–41
- Perrone J, Phillips C, Gaieski D. (2011). Occult metformin toxicity in three patients with profound lactic acidosis. J Emerg Med 40:271–5
- Popli H, Nair MS. (1996). Niosomal delivery of tenoxicam. Indian J Pharm Sci 58:163–6
- Raja Naresh RA, Chandrashekhar G, Pillai GK, Udupa N. (1994). Anti-inflammatory activity of niosome encapsulated diclofenac sodium with Tween-85 in Arthitic rats. Indian J Pharmacol 26:46–8
- Sammour OA, Abu-Zaid SA, Abu-Selem ME, et al. (2004). Formulation and evaluation of timolol maleate-loaded niosomes as an ocular drug delivery system. Bull Fac Pham Cairo Univ 42:249–61
- Scheen AJ. (1996). Clinical pharmacokinetics of metformin. Clin Pharmacokinet 30:359–71
- Srinivas S, Anand Kumar Y, Hemanth A, Anitha M. (2010). Preparation and evaluation of niosomes containing aceclofenac. Dig J Nanomater Biostruct 5:249–54
- Trinder P. (1969). Determination of glucose in blood using glucose oxidase with an alternative oxygen acceptor. Ann Clin Biochem 6:24–7
- UK Prospective Diabetes Study (UKPDS) Group. (1998). Effect of intensive blood-glucose control with metformin on complications in overweight patients with type 2 diabetes (UKPDS 34). Lancet 352:854–65
- Van Hal DA, Bouwstra JA, Rensen AV, et al. (1996). Preparation and characterization of non-ionic surfactant vesicles. J Colloid Interface Sci 178:263–73
- Vidon N, Chaussade S, Noel M, et al. (1988). Metformin in the digestive tract, diabetes. Res Clin Pract 4:223–9
- Vyas SP, Mysore N, Jaitely V, Venkatesan N. (1998). Discoidal niosome based controlled ocular delivery of timolol maleate. Pharmazie 53:466–9
- West JL, Halas NJ. (2000). Applications of nanotechnology to biotechnology commentary. Curr Opin Biotechnol 1:215–17
- Yoshioka T, Sternberg B, Florence AT. (1994). Preparation and properties of vesicles (niosomes) of sorbitan monoesters (Span-20, 40, 60 and 80) and sorbitan triester (Span 85). Int J Pharm 105:1–6