Abstract
The attenuated or non-pathogenic live vectors have been evolved specifically to deliver DNA into cells as efficient delivery tools in gene therapy. Recently, a non-pathogenic protozoan, Leishmania tarentolae (L.tar) has attracted a great attention. In current study, we used Leishmania expression system (LEXSY) for stable expression of HPV16 E7 linked to different mini-chaperones [N-/C-terminal of gp96] and compared their immunogenicity and protective effects in C57BL/6 mice against TC-1 challenge. TC-1 murine model is primary C57BL/6 mice lung epithelial cells co-transformed with HPV16 E6, HPV16 E7 and ras oncogenes. Our results showed that subcutaneous administration of mice with both the recombinant L.tar-E7-NT (gp96) and L.tar-E7-CT (gp96) led to enhance the levels of IFN-γ and also IgG2a before and after challenge with TC-1. Furthermore, L.tar-E7-CT (gp96) live vaccine indicated significant protective effects as compared to control groups as well as group vaccinated with L.tar-E7. Indeed, the recombinant live vector is capable of eliciting effective humoral and cellular immune responses in mice, but however, further studies are required to increase their efficacy.
Introduction
Infection with human papillomavirus (HPV), particularly HPV16, can result in cervical cancer (Bolhassani et al., Citation2009; Seo et al., Citation2009). Several therapeutic vaccines that target the HPV E6 and E7 proteins have been developed over the past years (Kanodia et al., Citation2008). Various forms of vaccines such as vector-based vaccines, tumor-based vaccines, DNA-based vaccines and protein/peptide-based vaccines have been described in experimental systems targeting HPV16 E6 and/or E7 proteins (Bolhassani et al., Citation2009). Recently, live recombinant vectors are central in the development of new vaccine strategies. Biological carriers are viruses or bacteria, which were naturally evolved to infect cells and transfer their genetic materials into the host cells (El-Aneed, Citation2004). Viruses used in gene therapy are modified in the laboratory to eliminate their pathogenicity and retain their high efficiency in gene transfer (El-Aneed, Citation2004). Intracellular bacteria (e.g. Listeria and Salmonella) as well as viral vectors (e.g. Vaccinia, adenovirus) have been extensively used for vaccine development against HPV infection (Kanodia et al., Citation2008). However, the use of bacterial or viral recombinant vectors as candidate vaccines in humans is hampered due to problems of either pre-existing immunity or inefficient antigen delivery and safety issues (Breton et al., Citation2005). Thus, there is an urgent need for the development of new safe live vaccine vectors that are capable of enhancing antigen presentation and eliciting potent immune responses without the risk of development of disease in humans. Recently, Leishmania tarentolae (L.tar), a unicellular eukaryotic protozoan, has been established as candidate for heterologous genes expression (Basile & Peticca, Citation2009). The studies have shown the potential of L. tarentolae that is non-pathogenic to humans, as a live candidate vaccine. L. tarentolae can activate the dendritic cell maturation process and induces T cell proliferation and the production of IFN-γ, thus skewing CD4+ T cells toward a Th1 cell phenotype (Breton et al., Citation2005). Currently, we showed that a recombinant L. tarentolae strain expressing high levels of full-length HPV16 E7 was immunogenic and could induce the production of IFN-γ cytokine. Furthermore, the protective effects of both subcutaneously DNA and live vaccine strategies were similar in tumor mice model (Salehi et al., Citation2012). These data indicated that further studies are required to increase live vaccine efficacy using different potent components such as adjuvants.
Heat-shock proteins are a family of chaperone proteins that facilitate delivery of non-covalently bound peptide to MHC class I molecules and induce peptide-specific CTL responses. Some studies aim at exploring the adjuvant properties of heat shock proteins (HSPs) in vaccine design (Srivastava, Citation2002). Indeed, HSPs serve as effective immunogens and have also been used in viral, protein and DNA-based vaccines. The immunogenicity of HSPs results from two different properties: (a) a peptide-dependent capacity to chaperone and elicit adaptive CTL responses against antigenic peptides and (b) a peptide-independent immunomodulatory capacity (Robert, Citation2003). The studies have shown that immunization with Glycoprotein 96 (Gp96) elicits protective immunity to the cancer or virus-infected cells from which it is derived (Chandawarkar et al., Citation2004). Gp96 resides in the lumen of the ER and is the most abundant ER protein. Gp96 contains an N-terminal signal sequence (70 amino acid) characteristic of the ER-targeted proteins and a carboxy terminal KDEL sequence (Lys-Asp-Glu-Leu), which is a retention/retrieval signal from the Golgi to the ER (Robert, Citation2003). Several studies indicated that immune activities of HSPs (e.g. Gp96) reside within their N- or C-terminal fragments known as mini-chaperones (Biswas et al., Citation2006; Bolhassani & Rafati, Citation2008; Bolhassani et al., Citation2008). These potent regions of HSPs are involved in development of various vaccines including DNA-based vaccine and protein/peptide-based vaccine (Bolhassani & Rafati, Citation2008). Our previous study demonstrated that co-delivery of E7 + Gp96 as DNA/DNA and E7 + CT (gp96) as DNA/protein could be an effective approach to induce E7-specific immune responses as a potential vaccine candidate for cervical cancer (Bolhassani et al., Citation2008).
In the present study, we have used a Leishmania expression vector (LEXSY), which can mediate high-level production of heterologous proteins both intracellularly and extracellularly (Bolhassani et al., Citation2011). L. tarentolae was transfected with the expression cassettes containing E7-NT (gp96) or E7-CT (gp96) genes fused to the green fluorescent protein (GFP) as a reporter gene in order to rapidly and sensitively quantify Leishmania promastigotes. We showed that expression cassettes including E7-NT (gp96)-GFP/E7-CT (gp96)-GFP genes integrate in Leishmania 18 S rRNA through homologous recombination. In continuation, the potential of two recombinant L. tarentolae expressing E7-NT (gp96) and E7-CT (gp96) was evaluated to reduce HPV16 E7-overexpressing tumors in C57BL/6 mice model.
Materials and methods
Plasmid DNA constructs and preparation
For the generation of E7-NT (gp96)-GFP-expressing plasmid [pLEXSY-E7-NT (gp96)-GFP], the E7-NT (gp96)-GFP gene was sub-cloned from pcDNA-E7-NT (gp96)-GFP (previously provided by our groups in Molecular Immunology and Vaccine Research Lab.) into the BglII and KpnI cloning sites of pLEXSY-neo2 expression vector (Jena bioscience). Furthermore, to make the E7-CT (gp96)-GFP-expressing plasmid [pLEXSY-E7-CT (gp96)-GFP], the E7-CT (gp96) gene was sub-cloned from pUC-E7-CT (gp96) into the BglII and KpnI cloning sites of pLEXSY-GFP expression vector. DNA constructs containing E7-NT-GFP and E7-CT-GFP were purified in large-scale using Midi-kit (Qiagen). The presence of the inserted genes was confirmed by PCR and restriction enzyme digestion as detected on gel electrophoresis. Finally, DNA concentration was determined by the absorbance measured at 260 nm. The accuracy of these constructs was confirmed by DNA sequencing.
DNA manipulations
Approximately 10 µg of the expression plasmids were digested with SwaI restriction sites and the product was separated on a 0.8% agarose gel. The bands corresponding to the expected size was gel purified (QIAquick gel extraction kit) for electroporation.
Parasite growth and transfections
The L. tarentolae strain (ATCC 30267) was grown at 26 °C in complete M199 medium (Bolhassani et al., Citation2011). For transfection, 4 × 107 log-phase parasites re-suspended in ice-cold electroporation buffer (pH = 7.5) containing 8 µg of linearized pLEXSY-E7-NT-GFP or pLEXSY-E7-CT-GFP, stored on ice for 10 min and electroporated using Bio-Rad Gene Pulser Ecell under conditions of 500 µF, 450 V and pulse time ∼5–6 ms. The electroporated promastigotes were plated on solid media containing 50 µg/ml of G418 (Bolhassani et al., Citation2011). Clones were selected on Noble agar plates and further propagated in liquid M199 10% medium in the absence of G418. Expression of E7-NT-GFP and E7-CT-GFP proteins in promastigote stage of the recombinant Leishmania species was evaluated by Epi-fluorescent microscopy (Nikon, E200, ACT-1 software, Japan) as well as flow cytometry (BD: Becton Dickinson).
Confirmation of E7-NT (gp96)-GFP or E7-CT (gp96)-GFP gene integration by diagnostic PCR
Integration of the linearized expression cassettes into the ssu locus was confirmed by diagnostic PCR using genomic DNA of wild type and transgenic strains of L. tarentolae as template. For this purpose, a primer pair including one primer hybridizing within the expression cassette and one primer hybridizing to a ssu sequence not present on the plasmid were used (Bolhassani et al., Citation2011). Furthermore, additional diagnostic PCR was performed by specific primers for E7, GFP, E7-NT, NT-GFP, E7-CT and CT-GFP using genomic DNA of transgenic L. tarentolae.
RNA extraction and reverse-transcription PCR
RNA samples were extracted from promastigote form of wild type and transgenic parasites using RNeasy mini-kit (Qiagen) according to the manufacturer’s instructions. The cDNA synthesis was performed using Omniscript Reverse Transcriptase kit (Qiagen) from 1 µg of RNA (Bolhassani et al., Citation2011). PCR analysis was performed to amplify E7-NT, NT-GFP, E7-CT and GFP under standard conditions.
Western blot analysis
Promastigote forms were harvested by centrifugation at 3000 rpm for 10 min and washed in PBS 1X (Bolhassani et al., Citation2011). Samples from both wild type and transgenic L. tarentolae were separated by SDS-PAGE in a 12.5% (w/v) polyacrylamide gel. Western blot analyses were performed to confirm E7-CT-GFP or E7-NT-GFP protein expression using anti-HPV16 E7 monoclonal antibody (1:10 000 v/v, USBiological) or anti-GFP polyclonal antibody (1:5000 v/v; Acris antibodies GmbH) under standard procedures, respectively. The immunoreactive protein bands were visualized using peroxidase substrate named 3,3′-diaminobenzidine (DAB, Sigma, St. Louis, MO) (Bolhassani et al., Citation2011).
Preparation of the recombinant proteins and Freez-thaw parasites
For the production of recombinant E7, NT (gp96) and CT (gp96) proteins, the bacterial cultures containing pQE-E7, pQE-NT (gp96) and pQE-CT (gp96) were grown to an optical density of 0.6–0.8 at 600 nm and protein expression was induced with 1 mM IPTG for 2 h at 37 °C. Protein samples were analyzed by SDS-PAGE in 12.5% (W/V) polyacrylamide gel. The recombinant E7, CT and NT proteins were purified by affinity chromatography using 6xHis-tag according to the manufacturer’s instructions (Qiagen) (Bolhassani et al., Citation2008). In addition, the promastigotes of transgenic parasites were harvested from stationary phase cultures by centrifugation, washed two times with PBS and disrupted by 15 rounds of freezing and thawing. Protein concentrations were measured using BCA assay kit (Pierce, Rockford, IL) and kept at −70 °C prior to use.
Cells
TC-1 (ATCC number: CRL-2785) cancerous cell line (Ji et al., Citation1998) was cultured in complete RPMI 1640 (Mohit et al., Citation2012). On the day of tumor challenge, TC-1 cells were harvested by trypsinization, counted and finally re-suspended in PBS.
Mice immunization
Inbred C57BL/6 female mice, 6–8 week old were obtained from the breeding stocks maintained at the Pasteur Institute of Iran. All animal procedures were performed according to approved protocols and in accordance with the recommendations for the proper use and care of laboratory animals. Five groups of 12 mice were selected. For priming, test groups were immunized subcutaneously (s.c.) at the footpad with 2 × 107 stationary-phase recombinant L. tarentolae promastigotes expressing E7-CT-GFP (G1), E7-NT-GFP (G2) and E7-GFP (G3) proteins. The control groups (G4 & G5) were injected with L. tarentolae expressing GFP and PBS, respectively. We considered another control group as untreated or Naïve mice (G6) (10 mice) for evaluation of antibody responses and protective effects. For boosting (3 weeks after priming), all groups 1–5 received the regimens similar to priming. Three weeks after booster, all groups subcutaneously received 1 × 105 TC-1 cells on the right flank. shows the regimens of each group.
Table 1. Live vaccination in C57BL/6 mice by using E7 in different formulations.
Monitoring humoral immune response
Mice sera were collected from all groups before and 2 weeks after challenge. Pooled sera for each group were stored at −20 °C. IgG1 and IgG2a (1:1000, Southern biotechnology Association, USA) were measured using ELISA against recombinant (r) E7 (5 µg/ml), NT (gp96) (2.5 µg/ml), CT (gp96) (10 µg/ml) proteins and Freez-thaw (FT) Leishmania proteins (10 µg/ml) as coating antigens (Bolhassani et al., Citation2008).
Cytokine assay
Two mice from each group were scarificed before, 3 and 6 weeks after challenge and spleens were homogenized. After lysis using ACK buffer, splenocytes were washed and re-suspended in complete RPMI medium (Bolhassani et al., Citation2008). Cells were then seeded at a density of 2 × 106 cells/ml in the presence of 10 µg/ml rE7, rE7 + rNT, rE7 + rCT, Freez-thaw transgenic parasites as a recall antigen (provided in Molecular Immunology and Vaccine Research Lab.) and medium alone (RPMI 10%). Concanavalin A (ConA: 5 µg/ml) was also used as the positive control. Plates were incubated for 3 days at 37 °C in 5% CO2 humidified atmosphere. Culture supernatants from stimulated splenocytes under the same conditions were collected in triplicate and assayed for IFN-γ and IL-4 by a sandwich ELISA (R & D, Minneapolis, MN), according to the manufacturer’s instructions. The lower detection limits of IFN-γ and IL-4 were 2 and 7 pg/ml, respectively.
In vivo tumor protection
Three weeks after the second vaccination, mice were challenged subcutaneously with 1 × 105 TC-1 cells/mice. All mice were followed for 50 days to monitor tumor progression. Tumor growth was determined by direct palpation twice a week. At each time point, tumor size was determined by measuring the smallest diameter (a) and the biggest diameter (b) by caliper. Tumor volume was calculated using the formula: V = (a2b)/2 (Li et al., Citation2006).
Statistical analysis
Statistical analysis was performed using Prism 5.0 software (GraphPad, San Diego, CA). One-way ANOVA and Student’s t-test were performed to analyze immune responses as well as tumor volume measurements among different various immunization groups and compare individual data points, respectively. For all analyses, p < 0.05 was considered statistically significant. Data are presented as mean ± standard deviation (SD).
Results
Generation of recombinant L. tarentolae expressing E7-NT-GFP and E7-CT-GFP proteins
To determine whether L. tarentolae could express E7-NT-GFP or E7-CT-GFP proteins efficiently, the 2034 bp and 1788 bp fragments encoding the E7-NT-GFP and E7-CT-GFP were cloned into pLEXSY-neo2. Then, the recombinant plasmids were prepared in large scale with high purity, linearized and electroporated into parasites. The transformants were selected by plating on solid medium containing G418. Integration of genes in the genomic DNA of the recombinant cells was confirmed by PCR analysis. The expected 1 kb band was only observed from transformed cells indicating the correct integration of expression constructs into the ssu locus. The expected PCR products of GFP, E7-CT, CT-GFP, E7-NT and NT-GFP were appeared as ∼720 bp, ∼1068 bp, ∼1488 bp, ∼1314 bp and ∼1734 bp fragments for E7-NT-GFP and E7-CT-GFP positive clones. To verify mRNA synthesis, RT-PCR was performed on total mRNA extracted from wild and transformed cells. The desired bands for GFP, E7-CT, CT-GFP, E7-NT and NT-GFP were obtained in recombinant Leishmania promastigotes.
Monitoring of E7-NT-GFP or E7-CT-GFP expression in L. tarentolae as a live vector
The expression of E7-NT-GFP and E7-CT-GFP using GFP was monitored by Epi-fluorescent microscopy and flow cytometry. The expression of genes was readily evident from the intense green fluorescence of the parasites. Fluorescence-activated cell sorting (FACS) analysis indicated a clear quantitative separation between transfected and wild type parasites (). E7-NT-GFP and E7-CT-GFP expression in transgenic L. tarentolae were almost similar to GFP expression in L.tar-GFP at promastigote form (94% and 92.9% versus 97.5%, respectively). The stability of protein expression was monitored over a period of 3 months post-electroporation. There was no decrease in fluorescence intensity during this period in the absence of G418. Protein expression was also detectable in cell extracts of transgenic parasites compared to wild type by western blotting. As shown in , the dominant bands of ∼81 and ∼72 kDa detected in transgenic parasites expressing E7-NT-GFP and E7-CT-GFP, respectively. No such corresponding bands were revealed in the wild-type promastigotes.
Figure 1. (A) Detection of E7-NT-GFP (left) and E7-CT-GFP (right) expression by Epi-fluorescent microscopy. (B) FACS analysis of un-transfected parasites as a negative control (gray color) in contrast with L.tar-GFP as a positive control (red color, left), L.tar-E7-CT-GFP (blue color, medium) and L.tar-E7-NT-GFP (red color, right) at logarithmic phase. (C) Detection of E7-NT-GFP (above) and E7-CT-GFP (below) proteins in the recombinant live vectors by western blotting. Western blot analysis using an anti-GFP and anti-E7 antibodies showed high levels of expression of the full-length E7-NT-GFP (∼81 kDa) and E7-CT-GFP (∼72 kDa) proteins, respectively. GFP was detected at 27 kDa for L.tar-GFP as a positive control.
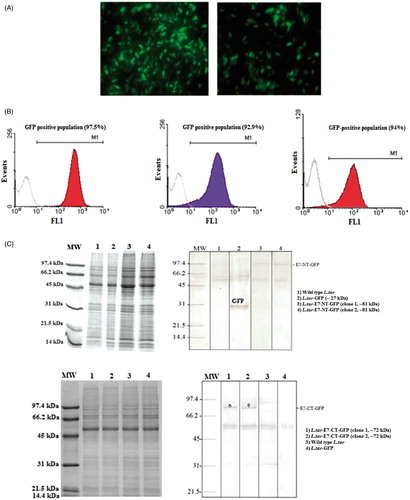
Immunization with L.tar-E7-NT-GFP and L.tar-E7-CT-GFP induces an IFN-gamma response in C57BL/6 mice
Protection following successful immunization requires the generation and maintenance of antigen-specific CD4+ Th cells. Herein, the levels of IFN-γ and IL-4 were compared between different groups. Our results indicated a significant increase in the level of IFN-γ following stimulation with recall antigens, in all test groups as compared to un-stimulated splenocytes. In addition, splenocytes from test groups (G1--G3) produced significantly higher levels of IFN-γ, before and after challenge as compared to control mice (G4, G5) (p < 0.05). However, the levels of IFN-γ in G1 and G2 were significantly higher than those in G3, before and 3 weeks after challenge with TC-1 (p < 0.05), confirming the induction of Th1 response (). It is worth to mention that IFN-γ immune responses were reduced gradually in G1 and G2 after challenge, but were significantly comparable with other groups. The peak response in G1 and G2 was elicited at 3 weeks after second immunization. Splenocytes from test groups (G1 and G2) didn’t elicit IL-4 production in comparison with control groups (p > 0.05).
Figure 2. The levels of IFN-γ were measured by a sandwich ELISA in culture supernatants of splenocytes following in vitro re-stimulation with the recombinant proteins and freez-thaw (F/T) transgenic L. tarentolae promastigotes. Vaccination with L.tar-E7-CT-GFP (G1) and L.tar-E7-NT-GFP (G2) induces IFN-γ production at before (A), 3 weeks (B) and 6 weeks (C) after challenge. p Values <0.05 have indicated statistically significant differences between G1/G2 and G3 vaccinated mice. The mean of ConA concentration is more than 600 pg/ml in all groups.
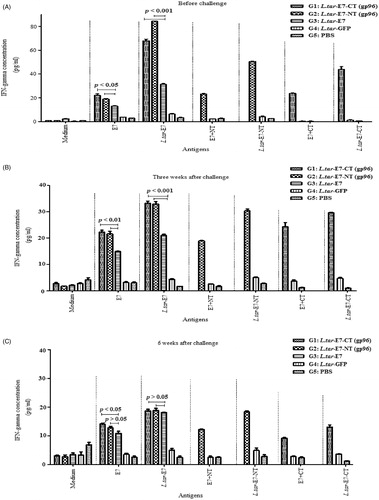
Immunization with transgenic L. tarentolae elicits high level of IgG2a after TC-1 challenge
We evaluated the levels of IgG1 and IgG2a antibodies against different antigens using ELISA before and 2 weeks after challenge. As shown in , IgG2a levels were significantly higher in test groups than those in control groups (p < 0.05). Interestingly, the levels of IgG1 did not increase in test groups compared to L.tar-GFP (G4), before and after TC1 challenge (data not shown).
Figure 3. Before (A) and 2 weeks (B) after challenge, mice were bled and the pooled sera from each group were used to determine antibody levels against different antigens by ELISA. Data represent means ± SD and each assay was performed in duplicate. p Values <0.05 have indicated statistically significant differences between G1/G2 and G3 vaccinated mice.
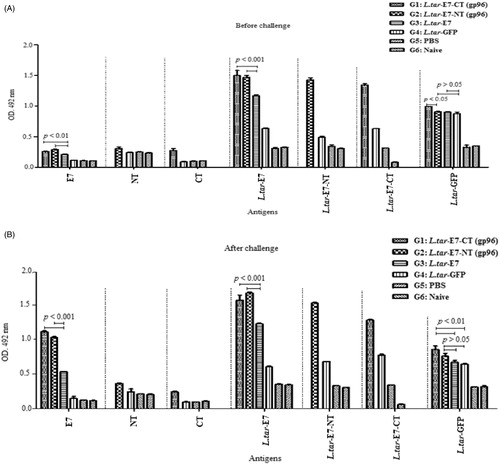
Vaccination with L.tar-E7-CT (gp96)-GFP reduced significantly tumor growth after TC-1 challenge
Considering that L. tarentolae is capable of eliciting a Th1-type cytokine response in mice, we tested whether vaccination with this vector expressing HPV16 E7-NT and E7-CT could protect mice against 1 × 105 cell/ml TC-1 tumors. C57BL/6 mice immunized with L.tar-E7-CT-GFP (G1) indicated considerably more protection than control groups even after 50 days (p < 0.05). Control groups (G4 and G5) and also Naïve mice developed progressive tumor growth. Our results indicated that group vaccinated with L.tar-E7-CT-GFP has significant protection compared to group vaccinated with L.tar-E7-GFP from 41 days after TC-1 challenge (p < 0.05) but not against group vaccinated with L.tar-E7-NT-GFP (p > 0.05) (). In addition, group vaccinated with L.tar-E7-NT-GFP did not show any significant protection in comparison with groups vaccinated with L.tar-E7-GFP (G3) and L.tar-GFP (G4) after TC-1 challenge (p > 0.05).
Figure 4. In vivo tumor protection experiments: C57BL/6 mice were subcutaneously challenged with 1 × 105/mouse of TC-1 tumor cells; Line graph depicting the tumor volumes in TC-1 tumor bearing mice treated with different regimens and untreated TC-1 tumor-bearing mice used as a control. Data is shown as means ± SD.
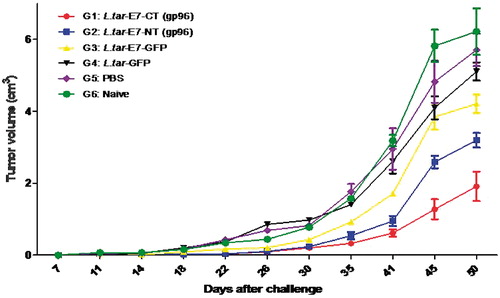
Discussion
In current study, we evaluated the potency of live vaccines expressing HPV16 tumor antigen (E7) linked to N-/C-terminal fragments of gp96 as an adjuvant. Regarding to studies, the majority of clinical trials using therapeutic agents have shown limited efficacy in eradicating established tumors in humans (Kanodia et al., Citation2008). The design of recent clinical trials may have improved to examine efficacy of therapy in patients with early stage cancers when their immune system is more competent. Thus, the necessity to focus efforts on the development of new therapeutic strategies remains. Live recombinant vaccines that express HPV E7 or E6 proteins have proven generally to stimulate strong CD4+ and CD8+ T cell responses in mice model (Lin et al., Citation2010). Various bacterial vectors have been explored in HPV therapeutic vaccines including Listeria monocytogenes (Sewell et al., Citation2008), Lactobacillus lactis (Bermudez-Humaran et al., Citation2005), Lactobacillus plantarum (Cortes-Perez et al., Citation2005) and Salmonella (Krul et al., Citation1996). Moreover, some effective properties make viral vectors a feasible option in therapeutic HPV vaccines (Lin et al., Citation2010). The Viruses include adenovirus (Gomez-Gutierrez et al., Citation2007), adeno-associated virus (Jin et al., Citation2005), vaccinia virus (Lamikanra et al., Citation2001) and alphaviruses (Cassetti et al., Citation2004). The tumor cells may express different levels of antigenic peptides loaded by class I MHC molecule. For example, a portion of TC-1 tumor cells may load either E6- or E7-derived antigenic peptides on their MHC class I molecule in vivo (Held et al., Citation2008). Thus, it is likely that some TC-1 tumor cells may not be lysed by CD8+ T cells generated from vaccination with a single antigen-encoding DNA vaccine, indicating the importance of broad immunity (Seo et al., Citation2009). Furthermore, targeting the activation of DCs is an important strategy associated with the development of cancer vaccines since these cells play a central role in the induction of anti-tumor immunity (Seo et al., Citation2009). In the present study, we investigated the potential of a non-pathogenic parasite, L. tarentolae (Basile & Peticca, Citation2009) against tumor as a live vaccine candidate vector to efficiently target DCs and lymphoid organs, thus enhancing antigen presentation and consequently influencing the magnitude and quality of T cell immune responses. In addition, the fusion of antigen with heat shock proteins is one such strategy for enhancing immune responses and regression of HPV-16 E7-expressing tumors in mice (Devaraj et al., Citation2003).
Herein, L. tarentolae expressing E7-NT-GFP or E7-CT-GFP proteins were stably generated to immunize mice. We showed that the L. tarentolae expressing E7-CT-GFP (G1) and also E7-NT-GFP (G2) induce the production of IFN-γ, thus skewing CD4+ T cells toward a Th1 cell phenotype and acting as an immunostimulatory adjuvant. These recombinant strains successfully generated significant levels of IgG2a compared to other groups before and after challenge with TC-1 tumor cells. A significant protection level of live L.tar-E7-CT-GFP was observed against the tumor outgrowth in comparison with live L.tar-E7 and control groups from 41 days after TC-1 challenge but not against group vaccinated with L.tar-E7-NT-GFP. Regarding to the same IFN-gamma response in both L.tar-E7-CT-GFP and L.tar-E7-NT-GFP groups against L.tar-E7 antigen; thus, the protection observed in mice immunized with L.tar-E7-CT-GFP through s.c. route could probably be attributed to other cytokines–secreting T cells toward a Th1 cell phenotype. It is clear that s.c. administration is among the most applicable means of vaccine delivery in human (Ravindran et al., Citation2012).
A study showed that the recombinant L. tarentolae elicits an HIV-specific T-cell-mediated immunity (Barouch et al., Citation2003), which makes it an attractive candidate not only against HIV-1 (Breton et al., Citation2007), but also against other intracellular pathogens such as Leishmania infantum (Mizbani et al., Citation2009). Recently, a recombinant non-pathogenic clone of Trypanosoma cruzi expressing a cancer testis antigen (NY-ESO-1) has been used as a vaccine vector to induce long-term T cell-mediated immunity and delay the rate of tumor development in mice (Junqueira et al., Citation2011).
Some groups isolated molecular chaperones (Gp96 and Hsp90) as tumor-specific transplantation antigens, which provoked a highly specific immune response against a certain type of tumor (Robert, Citation2003; Bolhassani & Rafati, Citation2008). Gp96 exerts adjuvant activity with some viral and bacterial antigens when applied in the form of a DNA vaccine (Bolhassani & Rafati, Citation2008; Bolhassani et al., Citation2008). Some studies have shown the adjuvant activity of the N-/C-terminal domains of gp96 in DNA or protein vaccine strategies. For instance, fusion of the C-terminal domain to Her2/neu inhibited the tumor growth (Pakravan et al., Citation2010). Moreover, the N-terminal domain has also potent adjuvant activity toward hepatitis B surface antigen (Yan et al., Citation2007). The controversy surrounding adjuvant activity of these terminal domains of gp96 prompted us to compare their adjuvant activity toward HPV16 E7, as live vaccine in tumor mice model.
In summary, this study showed the potential value of live L.tar-E7-CT-GFP vaccine that is successful in eliciting the proper immune responses and further protective effects. However, this formulation could not confer full protection against tumor challenge after 50 days. Two reasons could be assumed: (1) failure of the immune system at the final stages or (2) antigen loss and tumor escape (He et al., Citation2005; Kmieciak et al., Citation2007). Thus, further studies are required to generate more potent live vaccine candidate and long-lived protective immunity against tumors using immuno-adjuvants.
Declaration of interest
The authors report no conflicts of interest.
References
- Barouch DH, McKay PF, Sumida SM, et al. (2003). Plasmid chemokines and colony-stimulating factors enhance the immunogenicity of DNA priming-viral vector boosting human immunodeficiency virus type 1 vaccines. J Virol 77:8729–35
- Basile G, Peticca M. (2009). Recombinant protein expression in Leishmania tarentolae. Mol Biotechnol 43:273–8
- Bermudez-Humaran LG, Cortes-Perez NG, Lefevre F, et al. (2005). A novel mucosal vaccine based on live Lactococci expressing E7 antigen and IL-12 induces systemic and mucosal immune responses and protects mice against human papillomavirus type 16 induced tumors. J Immunol 175:7297–302
- Biswas C, Sriram U, Ciric B, et al. (2006). The N-terminal fragment of GRP94 is sufficient for peptide presentation via professional antigen-presenting cells. Int Immunol 18:1147–57
- Bolhassani A, Mohit E, Rafati S. (2009). Different spectra of therapeutic vaccine development against HPV infections: a review. Human Vaccines 5:1--11
- Bolhassani A, Rafati S. (2008). Heat shock proteins as powerful weapons in vaccine development: a review. Expert Rev Vaccine 7:1185–99
- Bolhassani A, Taheri T, Taslimi Y, et al. (2011). Fluorescent Leishmania species: development of stable GFP expression and its application for in vitro and in vivo studies. Exp Parasitol 127:637–45
- Bolhassani A, Zahedifard F, Taghikhani M, Rafati S. (2008). Enhanced immunogenicity of HPV16 E7 accompanied by Gp96 as an adjuvant in two vaccination strategies. Vaccine 26:3362–70
- Breton M, Tremblay MJ, Ouellette M, Papadopoulou B. (2005). Live non-pathogenic parasitic vector as a candidate vaccine against visceral leishmaniasis. Infect Immun 73:6372–82
- Breton M, Zhao C, Ouellette M, et al. (2007). A recombinant non-pathogenic Leishmania vaccine expressing human immunodeficiency virus 1 (HIV-1) Gag elicits cell-mediated immunity in mice and decreases HIV-1 replication in human tonsillar tissue following exposure to HIV-1 infection. J Gen Virol 88:217–25
- Cassetti MC, McElhiney SP, Shahabi V, et al. (2004). Antitumor efficacy of Venezuelan equine encephalitis virus replicon particles encoding mutated HPV16 E6 and E7 genes. Vaccine 22:520–7
- Chandawarkar RY, Wagh MS, Kovalchin JT, Srivastava P. (2004). Immune modulation with high-dose heat shock protein gp96: therapy of murine autoimmune diabetes and encephalomyelitis. Int Immunol 16:615–24
- Cortes-Perez NG, Azevedo V, Alcocer-Gonzalez JM, et al. (2005). Cell-surface display of E7 antigen from human papillomavirus type 16 in Lactococcus lactis and in Lactobacillus plantarum using a new cell-wall anchor from lactobacilli. J Drug Target 13:89–98
- Devaraj K, Gillison ML, Wu TC. (2003). Development of HPV Vaccines for HPV-associated head and neck squamous cell carcinoma. Crit Rev Oral Biol Med 14:345–62
- El-Aneed A. (2004). An overview of current delivery systems in cancer gene therapy. J Control Release 94:1–14
- Gomez-Gutierrez JG, Elpek KG, Montes de Oca-Luna R, et al. (2007). Vaccination with an adenoviral vector expressing calreticulin-human papillomavirus 16E7 fusion protein eradicates E7 expressing established tumors in mice. Cancer Immunol Immunother 56:997–1007
- He YF, Wang XH, Zhang GM, et al. (2005). Sustained low-level expression of interferon-γ promotes tumor development: potential insights in tumor prevention and tumor immunotherapy. Cancer Immunol Immunother 54:891–7
- Held G, Neumann F, Sturm C, et al. (2008). Differential presentation of tumor antigen-derived epitopes by MHC-class I and antigen-positive tumor cells. Int J Cancer 123:1841–7
- Ji H, Chang EY, Lin KY, et al. (1998). Antigen-specific immunotherapy for murine lung metastatic tumors expressing human papillomavirus type 16 E7 oncoprotein. Int J Cancer 78:41–5
- Jin HS, Park EK, Lee JM, et al. (2005). Immunization with adenoviral vectors carrying recombinant IL-12 and E7 enhanced the antitumor immunity to human papillomavirus 16-associated tumor. Gynecol Oncol 97:559–67
- Junqueira C, Santos LI, Galvão-Filho B, et al. (2011). Trypanosoma cruzi as an effective cancer antigen delivery vector. PNAS 108:19695–700
- Kanodia S, Silva DMD, Kast WM. (2008). Recent advances in strategies for immunotherapy of human papillomavirus-induced lesions. Int J Cancer 122:247–59
- Kmieciak M, Knutson KL, Dumur CI, Manjili MH. (2007). Her2/neu antigen loss and relapse of mammary carcinoma are activity induced by T cell mediated anti-tumor immune responses. Eur J Immunol 37:675–85
- Krul MR, Tijhaar EJ, Kleijne JA, et al. (1996). Induction of an antibody response in mice against human papillomavirus (HPV) type 16 after immunization with HPV recombinant Salmonella strains. Cancer Immunol Immunother 43:44–8
- Lamikanra A, Pan ZK, Isaacs SN, et al. (2001). Regression of established human papillomavirus type 16 (HPV 16) immortalized tumors in vivo by vaccinia viruses expressing different forms of HPV16E7 correlates with enhanced CD8+ T cell responses that home to the tumor site. J Virol 75:9654–64
- Li Y, Subjeck J, Yang G, et al. (2006). Generation of anti-tumor immunity using mammalian heat shock protein 70 DNA vaccines for cancer immunotherapy. Vaccine 24:5360–70
- Lin K, Doolan K, Hung CF, Wu TC. (2010). Perspectives for preventive and therapeutic HPV vaccines. J Formos Med Assoc 109:4–24
- Mizbani A, Taheri T, Zahedifard F, et al. (2009). Recombinant Leishmania tarentolae expressing the A2 virulence gene as a novel candidate vaccine against visceral leishmaniasis. Vaccine 28:53–62
- Mohit E, Bolhassani A, Zahedifard F, et al. (2012). Immunomodulatory effects of IP-10 chemokine along with PEI600-Tat delivery system in DNA vaccination against HPV infections. Mol Immunol 53:149–60
- Pakravan N, Soleimanjahi H, Hassan ZM. (2010). Gp96 C-terminal improves Her2/neu DNA vaccine. J Gene Med 12:345–53
- Ravindran R, Maji M, Ali N. (2012). Vaccination with liposomal leishmanial antigens adjuvanted with monophosphoryl lipid-trehalose dicorynomycolate (MPL-TDM) confers long-term protection against visceral leishmaniasis through a human administrable route. Mol Pharm 9:59–70
- Robert J. (2003). Evolution of heat shock protein and immunity. Dev Comparative Immunol 27:449–64
- Salehi M, Taheri T, Mohit E, et al. (2012). Recombinant Leishmania tarentolae encoding the HPV type 16 E7 gene in tumor mice model. Immunotherapy 4:1107–20
- Seo SH, Jin HT, Park SH, et al. (2009). Optimal induction of HPV DNA vaccine-induced CD8+ T cell responses and therapeutic anti-tumor effect by antigen engineering and electroporation. Vaccine 27:5906–12
- Sewell DA, Pan ZK, Paterson Y. (2008). Listeria-based HPV16 E7 vaccines limit autochthonous tumor growth in a transgenic mouse model for HPV16 transformed tumors. Vaccine 26:5315–20
- Srivastava P. (2002). Interaction of heat shock proteins with peptides and antigen presenting cells: chaperoning of the innate and adaptive immune responses. Annu Rev Immunol 20:395--425
- Yan J, Liu X, Wang Y, et al. (2007). Enhancing the potency of HBV DNA vaccines using fusion genes of HBV-specific antigens and the N-terminal fragment of Gp96. J Gene Med 9:107–21