Abstract
The objective of this study was to develop pH-responsive silica nanoparticles by imidazole-based ionic liquid for controlled release of methotrexate. In this article, we synthesized pH-responsive cationic silica nanoparticles by graft copolymerization of vinyl functionalized silica nanoparticles and methacrylic acid (MAA) monomer. Imidazole-based ionic liquid (Im-IL) was verified by 1HNMR and Fourier-transform infrared (FTIR) spectroscopy. The synthesized functionalized silica particles were characterized and confirmed by various technologies including the scanning electron microscopy (SEM), the infrared spectroscopy (IR) and the thermogravimetric analysis (TGA). SEM results reveal the uniformity in size/shape of silica particles. This nanosystem is modified for targeted delivery of an anticancer agent methotrexate. The nanocomposite-MTX complex was formed at physiological pH (7.4) due to the electrostatic interactions between anionic carboxylic group of MTX molecules and cationic rings in carrier, while, the release of which can be achieved through the cleavage of the nanocomposite-MTX complex by protonation of carboxyl groups in the MTX segment that are sensitive to variations in external pH at weak acidic conditions. FT-IR spectroscopy showed the presence of light interactions between the silicate silanols and the drug. MCF7 cells were incubated with the MTX-free nanocomposite and MTX-loaded nanocomposite at various concentrations for 24, 48 and 72 h, and the data showed that the nanocomposites themselves did not affect the growth of MCF7 cells. Antitumor activity of the MTX-loaded nanocomposites against the cells was kept over the whole experiment process. The results showed that the MTX could be released from the fibers without losing cytotoxicity.
Introduction
The development of new drug delivery systems, which can deliver precise quantities of therapeutic drugs to the targeted cells or tissues in a tailored release manner to enhance drug efficiency and reduce toxicity, has been one of the major thrust areas of pharmaceutical research these days (Singh & Lillard Jr, Citation2009; He & Shi, Citation2011; Ramteke et al., Citation2012). The adverse effects of anticancer drugs on healthy tissues on their way to the tumor target still represent a major drawback for their administration (Li et al., Citation2006). This general problem brought the need for the development of targeting drug delivery systems (DDSs), designed to administer cytotoxic drugs specifically to the cancer cells (He et al., Citation2011; Tung et al., Citation2011). In recent years, much effort was devoted to the development of DDSs based on mesoporous silica nanoparticles (MSNs) (Knežević et al., Citation2011; Singh et al., Citation2011), because they possess high specific surface, high concentration of surface silanol groups, ordered pores with tunable pore size, mesopore volume, superior chemical and thermal stabilities as well as the accessible surface fictionalization (Gan et al., Citation2011; Gao et al., Citation2011; Bitar et al., Citation2012; Najafi et al., Citation2012; Mahkam). MSNs offer both interior pore and exterior particle surfaces for loading different guest molecules but without appropriate surface modification, MSNs are unable to intelligently deliver drugs in a targeted and controlled manner (Gao et al., Citation2010). The presence of surface silanol groups enables chemical functionalization with different stimuli-responsive organic molecules. Several MSNs-based controlled release systems have been developed by the application of different kinds of pore blockers, including inorganic nanoparticles (Muhammad et al., Citation2011), organic molecules (Mahkam et al., Citation2012), polymer (Colilla et al., Citation2013) and supramolecular assemblies (Xue et al., Citation2011). Various physical/chemical stimuli, such as photo, pH, temperature and enzymes, have been used as actuators for uncapping the gatekeepers and releasing entrapped guest molecules (Xue et al., Citation2011). As the acidic tumor microenvironment is most common in solid tumors, the pH targeting approach is regarded as a more general strategy than many other targeting approaches (Meng et al., Citation2010; Deng et al., Citation2011; Wen et al., Citation2012; Xi et al., Citation2012). Methotrexate is an anticancer drug which is widely used for the treatment of breast cancer and epidermoid cancer of the head and lung cancer (Kang et al., Citation2002). However, it is reported to have toxic side effects to normal cells, drug resistance, and leucopoenia and nephrotoxicity (Tung et al., Citation2011). Here, we report a MTX delivery system responsive to a decrease in pH value. MTX was attaching to the silica surface, due to formation of strong hydrogen bonds and charge interaction with positive charge carrier. Hence, the idea was to utilize this adsorption ability of MTX for construction of a MSN-based drug carrier and then utilize its positive charge to gain the control over its adsorption/desorption equilibrium in aqueous environment. For that function, we devised MSNs functionalized with imidazolium-based positively charged ionic liquid and (PMAA) chains were grafted onto vinyl functionalized silica nanoparticles. The grafting procedure consisted of surface activation with 3-(trimethoxysilyl) propyl methacrylate (TSPA), followed by free-radical graft polymerization of methacrylic acid (MAA), using AIBN (Azobisisobutyronitrile) as an initiator. We report these novel MSNs as a delivery system based on the operation of nanovalves that are closed at physiological pH (7.4) but capable of opening and delivering drugs in acidifying cellular compartments. The MSNs employed in this work are ∼100 nm spheres. Nanoparticles were characterized by SEM, TGA and FT-IR spectroscopy. In vitro cellular cytotoxicity test was performed to evaluate the biocompatibility of ImIL-MSNs-PMAA, and the cytotoxic effect of drug-loaded carrier MTX@ ImIL-MSNs-PMAA to MCF7 cells was also investigated.
Materials and method
MAA (Sigma-Aldrich, St. Louis, MO) was distilled under a reduced pressure in nitrogen atmosphere; Tetraethylorthosilicate (TEOS), ammonia solution (37%), absolute methanol. The n-hexane was purchased from Sigma-Aldrich and used as received. MTT (3-(4,5-dimethylthiazol-2-yl)-2,5-diphenyltetrazolium bromide) assay and other biological reagents were purchased from Invitrogen Corp. 3-(Trimethoxysilyl) propyl methacrylate (TSPA) (99%) was purchased from Sigma-Aldrich. Methotrexate (vial 50 mg/5 mL) was purchased from (Koçak Farma, Istanbul, Turkey). AIBN (Azobisisobutyronitrile) was purchased from Merck Co. and all other chemicals were of analytical grade and purchased from Fluka Company.
Synthesis of silica nanoparticles
Silica nanoparticles were prepared according to the sol–gel method (Chruściel & Ślusarski, Citation2003; Jafarzadeh et al., Citation2009). In a 250-mL round bottom flask, 60 mL (10 mmol) ammonia solution (37%) and 1.98 g (110 mmol) water were added to 100 mL of absolute methanol. The solution was stirred vigorously for 5 min. Then, 10.41 g (500 mmol) (Tetraethoxysilane) TEOS was added dropwise. The final solution was stirred for three days at ambient temperature. Then, silica nanoparticles suspension was precipitated with n-hexane and extracted through centrifugation (twice at 6000 rpm). The obtained silica nanoparticles were freeze dried to give the white powder.
Synthesis of imidazolium-based ionic liquid (ImIL)
The synthesis is carried out under argon atmosphere. 1-Methylimidazole (2.060 g, 25.085 mmol) and (3-chloropropyl) trimethoxysilane (6.042 g, 25.091 mmol) were refluxed for three days at 80 °C. The orange suspension is filtered off and the solvent evacuated. By addition of 150 mL dry dichloromethane, a precipitate appears which is filtered off under argon atmosphere. The product is then separated by distillation at 150 °C under vacuum (1 mbar) and 1-methyl-3-(3-trimethoxysilylpropyl) imidazolium chloride was obtained with a honey-like consistency at room temperature (in 98% yield).
Synthesis of cationic ImIL-modified silica nanoparticles (ImIL-MSNs)
Silica (1.016 g) was suspended in CH2Cl2 (5 mL) and 1-methyl-3-(3-trimethoxysilylpropyl) imidazolium chloride (300 mg, 0.929 mmol) dissolved in CH2Cl2, and then added. After stirring the mixture for three days at ambient temperature, the silica was allowed to settle down. The supernatant solution was decanted and the modified silica was extracted with CH2Cl2 prior to being dried for several hours in vacuum.
Synthesis of ImIL-modified MSN
Hundred and seventy milliliter of ethanol and 7/2 mL TEOS were added to a 250-mL round bottom flask. Then, 28 mg 1-methyl-3-(3-trimethoxysilylpropyl) imidazolium chloride was added and 25 µl γ-methacryloxypropyltrimethoxysilane (MPS, 97 wt%) was added dropwise. The final solution is stirred for 24 h at ambient temperature. After completion of reaction, product was washed with ethanol to remove excess of unreacted materials.
Synthesis of cationic and pH-sensitive PMAA-grafted silica nanoparticles (ImIL-MSNs-PMAA)
One milliliter of methacrylic acid and 0.3 g modified nano silica from first step and 5 mL acetonitrile were stirred. To this mixture, 0.039 g AIBN (Azobisisobutyronitrile) initiator was added. The prepared mixture was placed in a clean polymerization tube, and the reaction mixture was kept for 10 min under argon flow. Then the tubes were sealed under argon atmosphere and the mixture polymerized for 48 h at 70–72 °C. After completion of reaction, the product was washed and completely pulverized in a mortar to achieve a fine powder.
MTX loading and release
Both silica-filled polymer nanocomposites were ultrasonically dispersed in predetermined amount of the MTX solution for five minutes with sonication (400 W) by using the probe-type ultrasonic generator. The weight ratios of the composite nanoparticles to the drug were 10:1 and 5:1. Then, the mixture was stirred at room temperature for 24 h. For the determination of encapsulation efficiency, the dispersion was centrifuged at 14000 rpm for 10 min to collect the MTX-loaded composite microspheres. The supernatant contains unentrapped MTX, which was removed, and the remaining pellet in the centrifuge tube resuspended in sodium hydroxide (0.1 N) (as MTX is highly soluble in 0.1 N NaOH) and vortexed thoroughly for 3 min. After vortexing 1 mL of the suspension, it was taken in a micropipette and transferred to a test tube. To this, we added 5 mL of methanol and it was further vortexed for 2 min. The absorbance of resulting solution was measured using a Shimadzu UV Spectrophotometer at 303 nm after suitable 10 dilutions with methanol.
The encapsulation efficiency was calculated using the formula:
To study the release behavior, MTX-loaded cationic-modified silica nanoparticles, MTX-loaded cationic and pH-sensitive PMAA-grafted silica nanoparticles were re-dispersed in 2 mL buffer solutions and transferred into a dialysis bag (MWCO 12000 Da, Sigma-Aldrich). The bag was subsequently placed in a 10-mL PBS solution at 37 °C. The medium was stirred continuously during the release study. During the appropriate time intervals, the whole solution (10 mL) was collected and at the same time, 10 mL of the fresh PBS solution was added. Two pH values of 4 and 7.4 were used to simulate the different biological environments. The amount of MTX in the release medium was measured by UV at the wavelength of 303 nm. All measurements were performed in triplicate. The releasing content was calculated by the following formula:
Cell culture and In vitro cytotoxicity assay
MCF7 cell lines were obtained from NCBI (National Cell Bank of Iran, Pasteur Institute) as the target cells and were used to evaluate the cytotoxicity of ImIL-MSNs-PMAA using MTT (3-(4,5-dimethylthiazol-2-yl)-2,5-diphenyltetra-zolium bromide) assay. Also, the antitumor activity of the free MTX and MTX@ImIL-MSNs-PMAA was evaluated by MTT method as previously described (Salehi et al., Citation2013). The cells were cultured in Roswell Park Memorial Institute 1640 growth medium (RPMI 1640 medium; Gibco BRL Life Technologies) containing 10% fetal bovine serum, 100 U/mL penicillin and 100 µg/mL streptomycin and incubated at 37 °C in an atmosphere of 5% CO2 and 95% air with more than 95% humidity. Medium was changed every other day for cell feeding. When cell population reached to 70% confluence, they were dissociated with 0.25% trypsin in PBS (pH 7.4) and centrifuged at 900 rpm for 8 min at room temperature. Cells were seeded into 96-well microtiter plates with a cell density of 2 × 104 cell/well in 180 μL RPMI 1640 and incubated in a humidified atmosphere containing 5% CO2 at 37 °C for 24 h to allow for attaching to the bottom of the well. After incubation for 24 h, the medium was aspirated and various concentrations (0.8, 0.6, 0.4 and 0.2 mg/mL) of samples (free MTX, MTX@ ImIL-MSNs-PMAA and ImIL-MSNs-PMAA) in fresh cell growth medium were added. Control cells were added with equivalent volume of fresh media. After incubation for a predetermined time, a microplate was withdrawn for morphology observation and for MTT assay. The MTT assay was performed twice. The old medium was removed and 100 µL of fresh medium and 10 µL of a 5 mg/mL MTT solution were added to each well. Plates were then incubated under cell culture conditions for 4 h. The old medium was spirated and 100 µL DMSO was added to dissolve the formazan crystals. The absorbance of each sample was measured at 570 nm with a background correction at 630 nm.
Result and discussion
Characterization
The silica nanoparticles-supported imidazolium ionic liquids (Im-IL) were prepared via grafting of organic spacers on the surface of synthetic silica nanoparticles (). In the second step, the cationic and pH-sensitive PMAA-grafted silica nanoparticles were obtained. The chemical structures of the IL-modified silica nanoparticles and PMAA-grafted silica nanoparticles were fully characterized by FT-IR and HNMR. Thermal behavior and morphology of products were analyzed by TGA and SEM-EDX.
FTIR
The chemical structures of the synthesized silica nanoparticles (), imidazolium ring ionic liquid (ImIL) (), cationic ImIL-modified silica nanoparticles (ImIL-MSNs) () and cationic and pH-sensitive PMAA-grafted silica nanoparticles (ImIL-MSNs-PMAA) () were studied by FTIR spectroscopy. It can be observed from that the absorption bands at 1641 must be due to aromatic C = C of imidazolium ring. The peak at 1572 is also attributed to stretching vibration of C = N of imidazolium aromatic ring. The absorption band at 3087 cm–1 is due to the vibration absorptions of aromatic ring (C–H) groups. The C–H stretching vibration of the aliphatic section is manifested through strong peak at 2950 cm–1. The peak at 1191 cm–1 is attributed to the stretching vibration of (C–O) for the three methoxy silane groups. In , in addition to all peaks of imidazolium ring ionic liquid, the typical Si–O–Si peak appeared around 1088 cm−1. A broad peak at 2800–3700 and 803 cm–1 is attributed to the stretching and out-of plane bending of free-silanol O–H groups, respectively. The FTIR spectra of PMAA-grafted silica nanoparticles (ImIL-MSNs-PMAA) () reveal a characteristic absorption band appearing at 1720 cm–1, which could be due to the absorption of carboxyl groups of PMAA (Yang et al., 2005). All other peaks are similar to with a slight shifting. The results suggest that the PMAA chains are grafted onto the silica nanoparticles by free-radical copolymerization.
Figure 2. FTIR spectra of (a) silica nanoparticles, (b) ImIL, (c) ImIL-MSNs, (d) ImIL-MSNs-PMAA and (e) MTX-loaded ImIL-MSNs-PMAA.
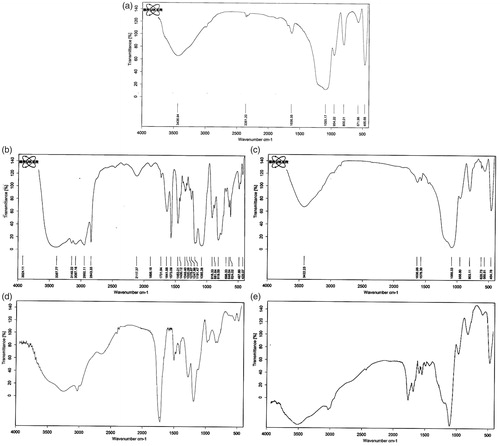
The FTIR spectra of MTX-loaded PMAA grafted silica nanoparticles (MTX@ImIL-MSNs-PMAA) () reveal a new peak at 1650 cm−1 that is attributed stretching vibration of CO–NH bond of MTX. The peak at 1720 cm–1 is weakened because of ionic interaction between the drug and carrier in the spectra of MTX-loaded PMAA-grafted silica nanoparticles (MTX@ImIL-MSNs-PMAA). Therefore, the FTIR results prove the successful encapsulation of MTX on the carrier.
1HNMR
shows 1H-NMR spectra of Imidazolium-based ionic liquid in CDCl3, in which the characteristic peaks of two component of ImIL are observed. The peaks at δ (ppm) 10.10 (1H, N–CH–N), 7.42 (1H, NCHCH), 7.23 (1H, NCHCH) and 3.83 (3H, N–CH3) are attributed to the protons of 1-Methylimidazole moiety of IL, and peaks at δ (ppm) 4.07 (2H, N–CH2), 3.29 (9H, O–CH3), 1.74 (2H, N–CH2CH2) and 0.36 (2H, Si–CH2) are attributed to the protons of trimethoxysylilpropyl moiety of IL.
Thermal analysis
The thermal stability and thermal decomposition of ImIL-MSNs-PMAA nanocomposites were investigated using TGA and DTG, shown in . TGA thermograms of ImIL-MSNs-PMAA nanocomposites exhibit the four-stage degradation behavior. The little weight loss below 100 °C (6.05% weight loss) is due to physically combined water (first step degradation). The second stage of degradation observed to occur at temperature range between 100 °C and 200 °C which is related to elimination of remaining organic solvents (9.2% weight loss). The third stage weight loss between 200 °C and 400 °C is attributed to the combustion (The maximum weight loss) of organic part (60.1% weight loss). The maximum rate degradation temperature (Tmax, first derivative peak temperature) was 400 °C. The final stage degradation at temperature range between 450 °C and 800 °C is ascribed to the dehydroxylation of silanol groups on poly-SiO2 samples and formation of siloxane groups (11.2% weight loss). The TGA curves demonstrated that the total weight loss was 85% at 750 °C.
The scanning electron microscopy
The morphologies and nanostructures of the prepared silica nanoparticles (), ImIL-modified silica nanoparticles (ImIL-MSNs) () and PMAA-grafted silica nanoparticles (ImIL-MSNs-PMAA) () are clearly revealed by SEM pattern shown in . SEM results reveal the uniformity in size/shape of synthesized silica nanoparticles () with a mean diameter of approximately 100 nm. ImIL-modified silica nanoparticles () were particles with the size range of about 150–200 nm. The prepared PMAA-grafted silica nanoparticles () are uniform spherical nanoparticles with a mean diameter of approximately 160 nm. After loading MTX in ImIL-MSNs ( and ImIL-MSNs-PMAA () nanoparticles, the pores of silica channels were filled with drug and the nanoparticles became more compact. The presence of silica nanoparticles was confirmed by the selected area EDX studies and the presence of all other elements are shown in .
Figure 5. SEM images of (a) silica nanoparticles, (b) ImIL-MSNs nanocomposites, (c) ImIL-MSNs-PMAA nanocomposites, (d) MTX-loaded ImIL-MSNs nanocomposites, (e) MTX-loaded ImIL-MSNs-PMAA nanocomposites, (f) selected area EDX analysis for ImIL-MSNs nanocomposite and its elemental composition and (g) selected area EDX analysis for ImIL-MSNs-PMAA nanocomposite and its elemental composition.
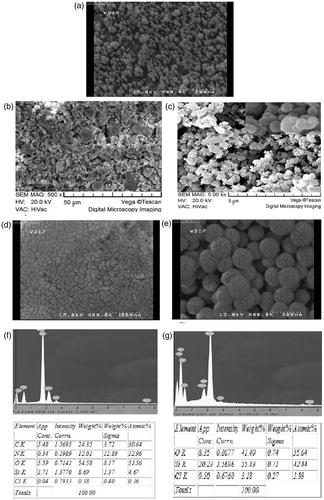
MTX loading and release
To evaluate the drug loading and releasing property of ImIL-modified silica nanoparticles (ImIL-MSNs) and PMAA-grafted silica nanoparticles (ImIL-MSNs-PMAA), MTX, a classic anionic anticancer drug, was used as a model drug. Previous studies have demonstrated that inorganic carriers such as MSN tended to have a high loading capacity, but with low encapsulation efficiency, opposite to the organic carriers such as micelles, liposomes and polymeric nanoparticles(Liu & Eisenberg, Citation2003; Carino et al., Citation2007; Vadia & Rajput, Citation2012). In this study, the ImIL-modified silica nanoparticles (ImIL-MSNs) and PMAA-grafted silica nanoparticles (ImIL-MSNs-PMAA) had high encapsulation efficiency of 80 ± 2.1% and 82.95 ± 5.3%, respectively. lists the drug storage data on mesoporous carriers.
Table 1. Loading percentages of various MTX-loaded MSN samples.
Schematic root of MTX loading and release on ImIL-MSNs (a) and ImIL-MSNs-PMAA (b) nanocomposite is presented in . MTX was loaded into the copolymers with drug/polymer ratio of 1/10 and 1/5 at pH of 7.4. The loaded drug by the composite nanoparticles may be attributed to the following reasons: (a) the drug loading experiment was performed at pH 7.4. The net charge of MTX was negatively charged at pH 7.4. There existed electrostatic attraction between MTX and the cationic ring of Imidazolium in silica-based nanoparticles. (b) There also existed hydrogen bonding complexation of the amino groups and COO– groups in MTX molecules with the ionized –COO− groups of PMAA and cationic ring of Imidazolium in silica-based nanoparticles at pH 7.4. (c) Also, there was another hydrogen bonding between amino groups in drug and deprotonated silanol groups. Therefore, large amount of the MTX molecules could be attached into the ImIL-modified silica nanoparticles (ImIL-MSNs) and PMAA-grafted silica nanoparticles (ImIL-MSNs-PMAA). It can be observed that drug decreased loading in 1/5 in comparison to 1/10 drug/polymer ratio may be because of the lack of adequate interaction between drug and polymer. However, a large amount of MTX was absorbed to the surface of silica and caused a large amount of burst release. depicts the release profiles of ImIL-modified silica nanoparticles (ImIL-MSNs) and PMAA-grafted silica nanoparticles (ImIL-MSNs-PMAA) at pH values of 7.4 and 4 released for more than two weeks at 37 °C. The release curves show that 38 and 40 wt% of MTX was released at first day from polymer/drug ratio of 5/1 compared to 20 and 7 wt% for 10/1 at pH 7.4, respectively. demonstrated that the MTX release rate was obviously pH dependent and increases with decrease in pH. When pH decreases to 4 (, a faster release behavior was obtained and the release amount reached 55 and 20 wt% (10/1) and 30 and 35(5/1) wt% in 24 h, respectively. In polymer/drug ratio of 5/1, the pH-sensitive release profile disappears because of surface absorption of MTX. In contrast, at polymer/drug ratio of 10/1, the drug release from the composite nanoparticles is completely pH sensitive and mainly determined by the effect of electrostatic interaction between MTX and polymer. Obviously, the pH-dependent release profile in ImIL-modified silica nanoparticles (ImIL-MSNs) was not as significant as that of PMAA-grafted silica nanoparticles (ImIL-MSNs-PMAA), where 63% and 100% of the MTX was released from ImIL-modified silica nanoparticles (ImIL-MSNs) after 250 h at pH values of 7.4 and 4, respectively, while 20% and 100% of MTX was released from PMAA-grafted silica nanoparticles (ImIL-MSNs-PMAA) after 250 h at pH values of 7.4 and 4, respectively. Therefore, PMAA-grafted silica nanoparticles (ImIL-MSNs-PMAA) display a more pronounced pH-dependent property than ImIL-modified silica nanoparticles (ImIL-MSNs). The pKa value for PMAA carboxylic groups is 5.5–6 (based on the copolymer composition). Therefore, at pH 4, the PMAA section in the polymer was in a loose state due to the hydrophilic effect of –COOH groups, thus, the drugs could diffuse faster (Yan et al., 2009). The pKa of MTX are 3.8, 4.8 and 5.6, and it is negatively charged at the pH 7.4 values. However, at pH 4, the drug is in a single positively charged state and the ionic interaction between drug/polymer disappears and the drug may be released from the carrier. Consequently, the higher the pH of the medium, the more negative charges are on the MTX (), and the stronger attraction exists between the negatively charged MTX and the positively charged carriers, thus, the more difficult for the loaded MTX to be released out of the carriers. Considering the fact that the tumor tissues are more acidic than normal tissues, the prepared composite microspheres would be able to minimize the side effect of MTX. Together with the specific drug release behavior and simultaneous high loading capacity and encapsulation efficiency for MTX, the PMAA-grafted silica nanoparticles (ImIL-MSNs-PMAA) composite microspheres are highly expected to be used in cancer treatment without frequent interval medication administrations. The reason for slower release of the drug from silica-based copolymers may be the interaction of carrier with the MTX. These results indicate that the release of MTX followed a sustain release pattern. PMAA-grafted silica nanoparticles (ImIL-MSNs-PMAA) have two parts, namely, cationic ring and PMAA section. The selection of these segments in carrier and MTX, as anticancer drugs, was to achieve the larger drug loading and pH-dependent drug release. It is preferable that MTX releases at mild acidic pH with its release being prohibited considerably at physiological pH (7.4). In this way, the normal cells will be protected from toxic side effect of MTX and only the cancer cells will be targeted. Therefore, the organic molecules that are coupled to MSN, act as stimuli-responsive switchable gates for controlled and targeted drug release.
In vitro cell assay
To investigate the biocompatibility of ImIL-MSNs-PMAA and anticancer activity of MTX@ImIL-MSNs-PMAA, MTT, cell assays have been conducted in . The cytotoxic effect of ImIL-MSNs-PMAA, without anticancer drug against MCF7 cancer cells after 48 h incubation, was studied using the MTT assays (). The ImIL-MSNs-PMAA shows no cytotoxicity to MCF7 cancer cells in concentration of up to 800 μg/mL, owing to the high biocompatibility of ImIL-MSNs-PMAA. The nanostractured ImIL-MSNs-PMAA at various concentrations shows no cytotoxicities to MCF7cancer cells, upon exposure for 48 h, revealing the safeness of the pH-responsive delivery systems in applications. On the other hand, the inhibition effect has been observed when MCF7 cancer cells are incubated with MTX@ImIL-MSNs-PMAA, which is an indication of the presence of high anticancer activities. In contrast, the cytotoxicity of MTX@ImIL-MSNs-PMAA unlike MCF7 cells is slightly lower than that of free MTX at all concentrations. This can be attributed to the sustained and part-release of MTX from the MTX@ImIL-MSNs-PMAA.
Conclusion
A novel versatile approach for preparing stimuli-responsive silica-PMAAc nanoparticles was demonstrated. MPS-modified silica nanoparticles were prepared by incorporation of pyridinum-based IL and abundant double bonds on the surface. Upon the free-radical copolymerization, the PMAA chains were grafted on the silica nanoparticle surface to form ImIL-MSN-PMAA composite nanoparticles. Cationic pH-sensitive PMAA-grafted silica nanoparticles synthesized in this study exhibited relatively high drug encapsulation efficiency (76%), due to the strong electrostatic interaction between both synthesized nanoparticles and MTX. The cumulative release of MTX@ ImIL-MSN-PMAA showed a low leakage at pH 7.4 with only 40% amount released after 400 h, while, significantly enhanced to 100% at pH 4 after 400 h. Faster MTX release under mild acidic conditions was due to the decrease of the electrostatic interactions between drug and matrix. We believe that these results may open the possibilities for pH-responsive targeted delivery of MTX to cancer tissues. The in vitro cellular cytotoxicity test revealed that the ImIL-MSN-PMAA is highly biocompatible and suitable to utilize as drug carriers. Therefore, the combination of MSNs, PMAAA polymer and pyridinum-based ionic liquid by creation of novel carrier resulted in the high loading capacity and pH-triggered controlled release of drug simultaneously, which would be a potential and promising strategy to design a stimuli-responsive delivery system.
Declaration of interest
The authors report no conflicts of interest. The authors alone are responsible for the content and writing of this article.
Supplementary Material
Download PDF (1.2 MB)References
- Bitar A, Ahmad NM, Fessi H, Elaissari A. (2012). Silica-based nanoparticles for biomedical applications. Drug Discov Today 17:1147--54
- Carino IS, Pasqua L, Testa F, et al. (2007). Silica-based mesoporous materials as drug delivery system for methotrexate release. Drug Deliv 14:491–5
- Chruściel J, Ślusarski L. (2003). Synthesis of nanosilica by the sol-gel method and its activity toward polymers. Mater Sci 21:461–9
- Colilla M, González B, Vallet-Regí M. (2013). Mesoporous silica nanoparticles for the design of smart delivery nanodevices. Biomater Sci 1:114–34
- Deng Z, Zhen Z, Hu X, et al. (2011). Hollow chitosan–silica nanospheres as pH-sensitive targeted delivery carriers in breast cancer therapy. Biomatter 32:4976–86
- Gan Q, Lu X, Yuan Y, et al. (2011). A magnetic, reversible pH-responsive nanogated ensemble based on Fe3O4 nanoparticles-capped mesoporous silica. Biomater 32:1932–42
- Gao Q, Xu Y, Wu D, et al. (2010). Synthesis, characterization, and in vitro pH-controllable drug release from mesoporous silica spheres with switchable gates. Langmuir 26:17133–8
- Gao Y, Chen Y, Ji X, et al. (2011). Controlled intracellular release of doxorubicin in multidrug-resistant cancer cells by tuning the shell-pore sizes of mesoporous silica nanoparticles. ACS Nano 5:9788–98
- He Q, Gao Y, Zhang L, et al. (2011). A pH-responsive mesoporous silica nanoparticles-based multi-drug delivery system for overcoming multi-drug resistance. Biomatter 32:7711–20
- He Q, Shi J. (2011). Mesoporous silica nanoparticle based nano drug delivery systems: synthesis, controlled drug release and delivery, pharmacokinetics and biocompatibility. J Mater Chem 21:5845–55
- Jafarzadeh M, Rahman I, Sipaut C. (2009). Synthesis of silica nanoparticles by modified sol–gel process: the effect of mixing modes of the reactants and drying techniques. J sol-gel Sci Technol 50:328–36
- Kang H, Kim J-D, Han S-H, Chang I-S. (2002). Self-aggregates of poly (2-hydroxyethyl aspartamide) copolymers loaded with methotrexate by physical and chemical entrapments. J Control Release 81:135–44
- Knežević NŽ, Trewyn BG, Lin VSY. (2011). Light-and pH-responsive release of doxorubicin from a mesoporous silica-based nanocarrier. Chem Eur J 17:3338–42
- Li L, Takemura G, Li Y, et al. (2006). Preventive effect of erythropoietin on cardiac dysfunction in doxorubicin-induced cardiomyopathy. Circ 113:535–43
- Liu F, Eisenberg A. (2003). Preparation and pH triggered inversion of vesicles from poly (acrylic acid)-b lock-polystyrene-b lock-Poly (4-vinyl pyridine). ACS 125:15059–64
- Mahkam M. (2012). Synthesis and characterization of novel positive-charge functionalized silica nanoparticles for oral drug delivery. IJAPBC 1:275--80
- Mahkam M, Hosseinzadeh F, Galehassadi M. (2012). Preparation of ionic liquid functionalized silica nanoparticles for oral drug delivery. J Biomater Nanobiotechnol 3:391–5
- Meng H, Xue M, Xia T, et al. (2010). Autonomous in vitro anticancer drug release from mesoporous silica nanoparticles by pH-sensitive nanovalves. ACS 132:12690–7
- Muhammad F, Guo M, Qi W, et al. (2011). pH-Triggered controlled drug release from mesoporous silica nanoparticles via intracelluar dissolution of ZnO nanolids. ACS 133:8778–81
- Najafi M, Yousefi Y, Rafati A. (2012). Synthesis, characterization and adsorption studies of several heavy metal ions on amino-functionalized silica nano hollow sphere and silica gel. Sep Purif Technol 85:193–205
- Ramteke K, Dhole S, Patil S. (2012). Transdermal drug delivery system: a review. J Adv Sci Res 3:22--35
- Salehi R, Irani M, Rashidi M-R, et al. (2013). Stimuli-responsive nanofibers prepared from poly (N-isopropylacrylamide-acrylamide-vinylpyrrolidone) by electrospinning as an anticancer drug delivery. Des Monomers Polym 16:515–27
- Singh N, Karambelkar A, Gu L, et al. (2011). Bioresponsive mesoporous silica nanoparticles for triggered drug release. ACS 133:19582–5
- Singh R, Lillard Jr JW. (2009). Nanoparticle-based targeted drug delivery. Exp Mol Pathol 86:215–23
- Tung W-L, Hu S-H, Liu D-M. (2011). Synthesis of nanocarriers with remote magnetic drug release control and enhanced drug delivery for intracellular targeting of cancer cells. Acta Biomater 7:2873–82
- Vadia N, Rajput S. (2012). Study on formulation variables of methotrexate loaded mesoporous MCM-41 nanoparticles for dissolution enhancement. Eur J Pharm Sci 45:8–18
- Wen H, Guo J, Chang B, Yang W. (2013). pH-responsive composite microspheres based on magnetic mesoporous silica nanoparticle for drug delivery. Eur J Pharm Biopharm. 84:91--8
- Xi J, Qin J, Fan L. (2012). Chondroitin sulfate functionalized mesostructured silica nanoparticles as biocompatible carriers for drug delivery. Int J Nanomedicine 7:5235
- Xue M, Zhong X, Shaposhnik Z, et al. (2011). pH-operated mechanized porous silicon nanoparticles. J Am Chem Soc 133:8798–801
- Yan EY, Ding Y, Chen CJ, et al. (2009). Polymer/silica hybrid hollow nanospheres with pH-sensitive drug release in physiological and intracellular environments. Chem Commun 19: 2718--20