Abstract
The skin accumulation of therapeutic agents affects the efficiency of topical drug delivery. In this study, in vitro distribution of finasteride of ethosomes and liposomes in human cadaver skin after percutaneous delivery were investigated. Experiments were performed using modified Franz diffusion cells. Finasteride ethosomes, liposomes or hydroethanolic solutions were used as donor medium. Drug distribution at different skin layers and depths were studied by hotplate separation and frozen horizontal slicing technique. The result showed that the accumulation of finasteride in skin ranged from 9.7–24.3 μg/cm2 at 12 or 24 hours. The ethosomes demonstrated better enhancing ability to deliver finasteride into the dermis layer than liposomes did. The finasteride concentration in the dermis layer from ethosomes was more than sevenfold higher than from liposomes. The finasteride accumulation in ethosomes group showed a distinctive reversed distribution profile. This distinctive reversed distribution profile is meaningful for exerting a favorable pharmacological effect for finasteride. The drug distribution profile in skin layers showed no significant difference between 12 and 24 hours application (p > 0.05). The study demonstrated that finasteride can be accumulated at target site more effectively and maintained at higher level through the application of novel ethosomal carriers.
Introduction
Vesicular carriers have been increasingly investigated to achieve targeted and controlled release of drugs, in contrast to their limited application in transdermal drug delivery or in topical drug delivery. Ethosomes, novel liposomes specially designed for percutaneous drug delivery, were used for enhancing the transdermal permeation of many therapeutic agents. The application was first put forward by Touitou in 1996 (Touitou, Citation1996) and was specially tailored to efficiently deliver various molecules into deep skin layers and across the skin (Ainbinder et al., Citation2010). Ethosomes has been studied for delivery of various drugs ranging from low molecular weight drugs such as acyclovir (Zhou et al., 2010), minoxidil (Jun-Bo et al., Citation2007), tacrolimus (Li et al., Citation2012), ligustrazine phosphate (Shi et al., Citation2012), econazole nitrate (Verma & Pathak, Citation2012), ibuprofen (Shumilov et al., Citation2010) to polypeptides and gene medicines (Godin & Touitou, Citation2003).
The novel vesicular and particulate drug delivery systems with sustained drug release profiles show promise for the topical application to reduce the side effects of drugs commonly used in the topical treatment. The action mechanism of ethosomes can be attributed to their ability to associate with the skin surface. The small particle size of ethosomes ensures close contact with the stratum corneum, which probably explains that the changes of the vehicle/stratum corneum partition coefficient by ethosomes facilitates encapsulated agents penetrating into the viable skin (Godin & Touitou, Citation2003). Studies have been conducted to explain the mechanism in which the ethosomes are able to increase the release of some drugs in the skin (Elsayed et al., Citation2006).
Finasteride, a 5α-reductase inhibitor, belonging to a 4-aza-3-oxosteroid compound, has been extensively studied and clinically used for the treatment of benign prostatic hyperplasia (BPH) and androgenetic alopecia (AGA) (Finn et al., Citation2006). The pharmacological mechanism of finasteride is to block the conversion of testosterone to stronger androgenic dihydrotestosterone (). As 5α-reductase is mainly located in the hair follicle and the prostate, it is more desirable to use percutaneous approach to deliver therapeutic agent directly into the targeted tissues to minimize systemic side effects (Liu & Yamauchi, Citation2008; Traish et al., Citation2011).
In previous study (Rao et al., Citation2008), a transdermal drug delivery system of finasteride-loaded ethosomes was developed. The influence of ethosomes on the percutaneous absorption of finasteride was studied.
In continuation with previous research (Rao et al., Citation2008), a study was initiated to qualitatively characterize the distribution of finasteride in human cadaver skin using modified Franz diffusion cells in vitro. The effects of ethosomes or liposomes on drug distribution in the different strata of the full-thickness human skin were evaluated. The flux and permeability coefficient were also studied. The feasibility of using ethosomal finasteride as a formulation for treating AGA or BPH was also evaluated.
Materials and methods
Materials
Human abdominal skin (supplied by Anatomy Department of ZheJiang University, China, and approved by Institutional Review Board of the First Affiliated Hospital of Zhejiang University). Micronized finasteride (mol wt. 372.55 Da; Melting point 250 °C; solubility 0.046 mg/mL; LogP 3.03) was obtained from XianJu Pharmaceutical Co., Ltd., Taizhou, China. Soybean phospholipid (SPL) was obtained from Lipoid GmbH, Germany. Ethanol and other compounds used in this work were of analytical or pharmacopeial grade.
Sample preparation
Ethosomes and liposomes were prepared and characterized as previously described (Rao et al., Citation2008). The SPL (3% w/w) and drug (0.2% w/w) were dissolved in ethanol in a sealed glass container, and then the water was added slowly in a constant stirring condition, keeping in mixing for 5 min. The final preparation was obtained after sonication 30 times for 2 s with pulses of 400 W by Ultrasonic Probe (JY92-II, Ningbo Scientz Biotechnology Co., Ltd. Ningbo, China) and filtration with 0.22 μm cellulose membrane for three times. All the procedures were processed at room temperature. The liposomes consisting of the same quantity of SPL and drug were prepared using film-dispersing method. The SPL and drug were dissolved in chloroform in a pear-shaped flask and a thin lipid film was formed under reduced pressure by rotary evaporator. The film was dispersed with water and completely hydrated. The final sample was processed with the method described in ethosomes preparation (Rao et al., Citation2008). Each time 20 mg drug was used for the preparation of 10 g formulation. Additionally, drug-saturated solutions in 30% ethanol were also prepared.
Drug quantification in the vesicles
The content of finasteride in the vesicles was determined by a rapid and sensitive reversed phase-high performance liquid chromatography (RP-HPLC). Approximately, 1 g of each formulation was accurately weighed and placed in a 50 mL volumetric flask. Methanol was added and the flask was heated at 65 °C until the vesicles were completely dissolved. The solution was cooled to room temperature and properly diluted with methanol. After filtration through a 0.45 μm hydrophilic membrane, an aliquot of 10 μL solution was injected to the RP-HPLC for determination.
Encapsulation/loading efficiency of vesicles
The drug encapsulation efficiency (EE) and loading efficiency (LE) were determined by standard equilibrium dialysis technique (Qi et al., Citation2006; Rao et al., Citation2008). Briefly, a known amount of vesicle sample was added into the donor cell. A solution contained 30% ethanol or water was used as the receptor cell medium. The two cells were separated by a semipermeable cellulose membrane with a MW cutoff of 10 000 Dalton’s. After the equilibrium was reached, samples were taken from both cells to measure the drug concentrations. The EE and LE were calculated according to Equations Equation1(1) and Equation2
(2) , respectively.
(1)
(2)
where VL is the volume of lipid phase, Vaq is the volume of aqueous phase, CL and Caq are the corresponding concentration. MWD is the molecular weight of drug, MWL is the molecular weight of lipid, which is 800 Dalton in this study.
In vitro skin permeation studies
Franz’s diffusion experiments
The frozen human cadaver skin without subcutaneous fat was thawed at ambient temperature prior to use. The skin was mounted onto the modified Franz diffusion cells (interface area 2.8 cm2; receptor volume 6.8 mL) and trimmed to size. The diffusion cell contained isotonic saline for transport kinetic balance of skin. After one hour’s balance, the isotonic saline was substituted with receptor medium, that is, isotonic saline containing 20% PEG400 as solubilizer and 0.02% sodium azide as preservative. And 0.5 mL sample was applied on each donor chamber of Franz cells. At given time intervals (2, 4, 6, 8, 12, and 24 h), an aliquot of 500 μL sample was collected and the same amount of fresh receptor medium was added to compensate the volume removed. The receptor compartment was maintained at 37 ± 0.5 °C using a circulating water bath. The diffusion experiments of vesicles or the controls were carried out for 12 or 24 h. Triplicate trials were conducted on all samples tested.
The flux was determined directly as the slope of the curve between the steady state values of the cumulative amount of drug versus time. The permeability coefficient (Kp) was calculated using the following equation (Alves et al., Citation2007):
(3)
where Kp is the permeability coefficient, C0 is the initial concentration of drug in the vehicle applied to the donor compartment.
Heat-separation of epidermis
After the completion of the diffusion experiments, the permeation setup was dismantled. The skin was removed from the cell and washed three times in 10 mL methanol for 15 s, and then was wiped with tissue paper to remove residual drug from the surface of the skin. After the skin was dehydrated in ambient air, the skin was immobilized on the surface of a clean glass board and the epidermis (stratum corneum and viable epidermis) was carefully removed from the dermis using forceps (Heard et al., Citation2003). The epidermis layers were cut into small pieces with scissors and extracted in 200 µL of methanol for 4 h at 60 °C. The extract was filtered by 0.45 μm filter and refrigerated until being analyzed with HPLC.
Frozen horizontal slicing of dermis
The quantitative determination of finasteride at different depths of dermis was performed on frozen horizontal slices. The remained dermis was punch biopsied and mixed with embedding medium before frozen in liquid nitrogen and mounted on a cryostat. Horizontal slices of 50 μm in thickness were cut, and sequentially collected in Eppendorf microtubes. From each slice, finasteride was extracted in 200 μL of methanol for 4 h at 60 °C. After samples were cooling, an aliquot of 200 µL of 1 N HClO4 was added to precipitate residual proteins. After the mixture was centrifuged at 5000 g for 5 min, the supernatant was filtered by 0.45 μm filter and refrigerated until being analyzed with HPLC.
RP-HPLC analysis
A rapid and sensitive RP-HPLC method was developed to simultaneously measure finasteride concentrations in the receptor medium and in the extracts. Samples were analyzed at the temperature of 40 °C using an Agilent 1100 series automated HPLC system. The instrument was fitted with an Eclipse XDB-C18 column (150 × 4.6 mm, 5 μm, Agilent). Detection wavelength was set at 230 nm. The injection volume was 100 μL. An isocratic elution program was used with the mobile phase of methanol: phosphate-buffer (75:25, pH = 3.0) and a flow rate of 1 mL/min. The impurities in skin samples are unlikely interfere with the analysis of finasteride due to a good separation of impurity and finasteride with the developed HPLC condition (). Calibration curves were constructed in the range of 0.40–20.0 μg/mL with a correlation coefficient >0.999 (). The detection limit of finasteride was 5 ng, as estimated based on the signal-to-noise (S/N) ratio of 3.
Statistical analysis
All skin permeability experiments were repeated at least three times and the results were expressed as mean ± standard deviation. Differences among different groups were analyzed with ANOVA and p < 0.05 were considered to be statistically significant.
Results
The encapsulation efficiency (EE) and loading efficiency (LE) were determined by the standard equilibrium dialysis technique. The EE of finasteride in two kinds of vesicles was calculated as percentages of the total drug amount in the vesicular system, while the LE was described in unit of mole of drug per mole lipid. The results showed that the EE of ethosomes was 78.1 ± 3.4%, which was nearly 20% higher than that of conventional liposomes. The LE of ethosomes was more than 5.59 × 10–2 mole/mole, almost six times higher than that of liposomes. The result suggested that the presence of ethanol in the vesicular systems increase the partition of the lipophilic finasteride (log P = 3.3) into the bilayer membrane to make the ethosomes a more efficient solubilization matrix than that of the conventional liposomes for transdermal delivery. These partition enhancement and solubilization effect are especially important for the drugs of low solubility or high lipophilicity.
The permeation and accumulation of finasteride in human cadaver skin was studied in vitro using modified Franz diffusion cell under occlusion condition. The percutaneous absorption profiles () and the kinetic permeation parameters () were compared through ethosomal suspensions, liposomal suspensions and the 30% hydroethanolic solutions.
Figure 3. The finasteride permeated through human cadaver skin in % of the applied amount, as a function of time. Each point represents the mean ± SD (n = 6).
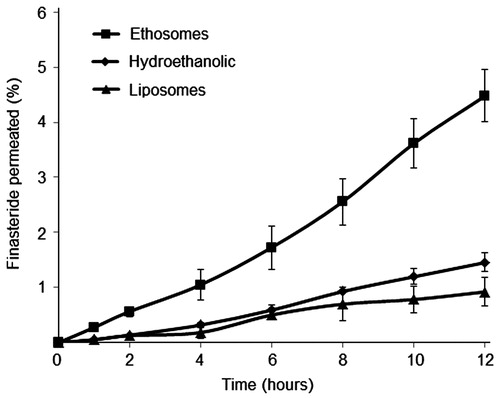
Table 1. Profiles of vesicular finasteride delivering drug into the strata of human cadaver skin using a Franz diffusion cell in vitro after 12 h (mean ± SD, n = 3).
showed the cumulative amount of finasteride permeated per unit area across skin as a function of time. The flux and lag time were calculated from slope and x-intercept of the linear portion, respectively, and the permeability coefficients (Kp) was calculated according to Equation (Equation3(3) ). The final finasteride concentrations of two vesicular suspensions were 1.93 × 103 μg/mL for ethosomes and 1.31 × 103 μg/mL for liposomes. The value of steady-state transdermal flux for finasteride ethosomal suspensions was 1.34 ± 0.11 μg/cm2/h with the Kp of 6.94 × 10−4 cm/h and the lag time of 2.2 h (). The conventional liposomes and 30% hydroethanolic solution provided significantly lower flux with value of 0.47 ± 0.06 μg/cm2/h and 0.58 ± 0.08 μg/cm2/h, respectively. No significant difference (p > 0.05) of flux and skin accumulation was found for prolonged permeation study (between 12 and 24 h). The drug permeability profiles showed a zero-order kinetics with the typical time lag of transdermal delivery. Among the three groups, the 30% hydroethanolic showed the shortest lag time of 1.5 h, followed by ethosomes of 2.2 h and liposomes of 4.7 h. The results confirmed the theory that ethanol could reduce the time needed for delivery systems to reach the steady state (Godin & Touitou, Citation2003; Kuo et al., Citation2009).
Twelve-hour of percutaneous permeation induced an accumulation of finasteride in skin ranging from 9.7 to 24.3 μg/cm2. showed that hydroethanolic solution induced a significantly high epidermis drug accumulation (11.3 ± 1.8 μg/cm2). Although the drug content in the epidermis for the ethosomes (6.11 ± 1.42 μg/cm2) and liposomes (6.89 ± 1.33 μg/cm2) showed no statistically significant difference, the enhanced percutaneous delivery of ethosomes was clearly demonstrated. The ethosomes showed the highest finasteride amount in skin (18.2 ± 1.1 μg/cm2) and in receptor fluid (42.7 ± 3.5 μg), especially in deeper dermis layers, in which the finasteride concentrations was 6.5, 3.4 times higher than that of liposomes and hydroethanolic solution, respectively (p < 0.01). The result also showed that 84.6% finasteride (46.0% in dermis and 38.6% in receptor fluid) from ethosomes was penetrated to or through the deeper skin layer. In the contrast, nearly half of finasteride from liposome (47.9%) or hydroethanolic solution (48.5%) were confined in external layer of the skin after 12 h in vitro penetration. The difference in the permeability of the finasteride from ethosomes versus liposomes and the 30% hydroethanolic solutions was due to the synergic effect of the presence of ethanol and SPL (Touitou et al., Citation2000). As is well known, the stratum corneum is considered to be the major diffusion barrier of human skin to the percutaneous permeation of topically applied substances (Barry, Citation2001). It is strongly suggested that the lower molecular weight ethanol has a high affinity of with the stratum corneum.
In the course of transdermal transport studies, large quantity of skin slice samples has already been analyzed using the ultrathin slicing technique, with the thickness value in range of 10–100 μm. The human abdominal skin specimen was assumed to have a thickness of about 40 μm for hydrated stratum corneum, 120 μm for the viable epidermis and the remaining for the dermis (Da et al., Citation2008). To further investigate drug distribution in deeper skin layer, the remaining dermis was uniformly sliced to 50 μm thickness to determine the content in different skin depth. showed the distribution profiles in dermis slices after 12 h diffusion experiment of ethosomes, liposomes and the hydroethanolic. The result showed that the ethosomes induced significant highly homogeneous accumulation of finasteride in dermis compared with liposomes or the hydroethanolic. Liposomes, however, showed a gradient distribution of drug in the dermis. The gradient distribution of drug was more obvious in the deep dermis layers (in 400 to 800 μm depth). This result indicated that ethanol can induce homogeneous distribution of drug in the deep dermis layer. However, SPL confines more diffusion molecule in the outer epidermis layer, especially in stratum corneum. Topical drug delivery can be modulated by these different profiles of vesicular carriers according to the different therapeutic purposes (Schroeter et al., Citation2010).
Discussions
A broad range of strategies has been tested for enhancing percutaneous absorption, including chemical enhancers, vesicular carriers, iontophoresis, electroporation, acoustical method, microneedle, jet injection, etc. (Dhote et al., Citation2012; van der Maaden et al., Citation2012). Among these strategies, novel vesicular and particulate drug delivery systems are the most extensively studied noninvasive routes for drug delivery to minimize side effects, while preserving their efficacy (Castro & Ferreira, Citation2008). Research continues into the interactions between novel particles and skin, and the influence of particle composition on drug distribution within the skin strata (Gupta et al., Citation2012). The vesicular carriers consist mainly of liposome, transfersome, noisome, and ethosome. Numerous studies have shown that conventional liposomes can enhance the accumulation of various molecules only in the upper skin strata, while ethosome and transfersome, novel liposomes, modified by appropriate composition could enhance and modulate the drug transport into or through the skin (Elsayed et al., Citation2007; Azeem et al., Citation2009).
Androgenetic alopecia (AGA) is a common disorder affecting both men and women. It affects at least 50% of men by the age of 50 years, and up to 70% of all males in later life and affects 6% of women aged under 50 years, and about 30–40% of women aged 70 years and over (Trueb, Citation2002). AGA is caused by androgen-dependent miniaturization of scalp hair follicles, with scalp dihydrotestosterone (DHT) implicated as a contributing factor. Finasteride decreases scalp DHT by blocking the conversion of testosterone to stronger androgenic DHT. Because 5α-reductase is mainly located in dermis layer of scalp, a successful AGA treatment requires constant topical drug levels throughout the course of the therapy (Libecco & Bergfeld, Citation2004; Finn et al., Citation2006). Hence, maintenance of constant drug levels via the percutaneous route using vesicular carriers with modulated delivery profiles could be a promising strategy in combating AGA.
In previous study, we discovered that high concentration of ethanol within ethosome reduced the size of the bilayer vesicles and induced a charge transition from positive to negative, which was a favorable physicochemical change for transdermal penetration (Gillet et al., Citation2011). Furthermore, ethanol has a fluidizing effect on both the ethosomal lipid bilayers and the highly ordered lipophilic structure of stratum corneum. In comparison to liposomes, ethosomes, similar to transfersome are more soft and flexible (Pierre & Dos, Citation2011). It was the synergistic effect of soft flexible vesicular structure, penetration enhancing and thermodynamic properties that makes ethosome an ideal delivery system for enhanced percutaneous transport.
Additionally, in many studies, to increase the solubility of poorly soluble drugs, many supersaturated solutions were prepared in vesicular preparations (Barichello et al., Citation2006; di Cagno & Luppi, Citation2013). In this study, the supersaturated strategy was adopted to increase the loading efficiency of liposomes. However, as the results shown, the drug loading efficiency of ethosomes was still much higher than that of liposome (1.93 × 103 μg/mL versus 1.31 × 103 μg/mL). The solubilizing effect of ethanol is apparently superior to regular supersaturated strategy in improving drug loading ability of vesicular preparations.
In vitro permeation studies provide valuable information about the product behavior in vivo. The amount of drug that diffuses through the epidermis dictates the amount of drug available for absorption. The results of human cadaver skin permeation study of finasteride demonstrated the enhanced transdermal delivery potential of ethosomes. Finasteride-loaded ethosomes had a maximum flux value, which is 2.9 and 2.3 times higher than that of conventional liposomes and hydroethanolic solution, respectively. These findings were consistent with previous observations on ethosomes loaded with a broad range of agents which induced a considerable skin accumulation and higher flux (Elsayed et al., Citation2006; Zhou et al., 2010).
The skin deposition profile of finasteride obtained from ethosomal carriers was also better as compared with liposomal carriers or hydroethanolic solution. In this study, an interesting reverse skin distribution phenomenon of ethosomes versus liposomes or hydroethanolic solution was confirmed for the first time. The ratio of finasteride deposited in epidermis to dermis was 15.4% versus 46.0% for ethosomes, while the corresponding ratio of liposomes and the hydroethanolic was 47.9% versus 19.4% and 48.5% versus 22.8%, respectively. This distinctive reversed distribution profile is meaningful for exerting a favorable pharmacological effect for finasteride. Both the enhancing efficacy and the reverse distribution property could be justified on the basis of dual function performed by ethanol present in the ethosomal carriers – that is, fluidizing both the vesicular lipid bilayers and stratum corneum lipids, thus providing a greater malleability to the vesicles and enhancing permeability of the skin (Xu et al., Citation2007).
Conclusions
This study showed that the insoluble drug finasteride can be effectively delivered into the human cadaver skin. After application of ethosomes under occlusion condition, finasteride was accumulated homogeneously in the deeper dermis layers. Twelve or twenty-four hours of percutaneous permeation experiments induced an accumulation of finasteride in skin ranging from 9.7 to 24.3 μg/cm2. The ethosomes showed stronger enhancing ability to deliver finasteride into the dermis layer, in which the drug concentration was nearly sevenfold of liposomes. The finasteride accumulation of ethosomes showed a distinct reverse distribution profile versus liposomes or hydroethanolic solution. All the results demonstrated that finasteride-loaded ethosomes is a promising percutaneous drug delivery strategy for treating AGA.
Acknowledgements
We also would like to thank Professor Feng Li from Hampton University for his proofreading of the English.
Declaration of interest
The authors report no declarations of interest. We are grateful for the financial support of the National Nature Science Foundation of China (81072231) and thank the support from the Chinese Postdoctoral Science Foundation (2012M510097, 2013T60478).
References
- Ainbinder D, Paolino D, Fresta M, et al. (2010). Drug delivery applications with ethosomes. J Biomed Nanotechnol 6:558–68
- Alves MP, Scarrone AL, Santos M, et al. (2007). Human skin penetration and distribution of nimesulide from hydrophilic gels containing nanocarriers. Int J Pharm 341:215–20
- Azeem A, Anwer MK, Talegaonkar S. (2009). Niosomes in sustained and targeted drug delivery: some recent advances. J Drug Target 17:671–89
- Barichello JM, Handa H, Kisyuku M, et al. (2006). Inducing effect of liposomalization on the transdermal delivery of hydrocortisone: creation of a drug supersaturated state. J Control Release 115:94–102
- Barry BW. (2001). Novel mechanisms and devices to enable successful transdermal drug delivery. Eur J Pharm Sci 14:101–14
- Castro GA, Ferreira LA. (2008). Novel vesicular and particulate drug delivery systems for topical treatment of acne. Expert Opin Drug Deliv 5:665–79
- Da SD, Thiago SB, Pessoa FA, et al. (2008). Penetration profile of taurine in the human skin and its distribution in skin layers. Pharm Res 25:1846–50
- Dhote V, Bhatnagar P, Mishra PK, et al. (2012). Iontophoresis: a potential emergence of a transdermal drug delivery system. Sci Pharm 80:1–28
- di Cagno M, Luppi B. (2013). Drug “supersaturation” states induced by polymeric micelles and liposomes: a mechanistic investigation into permeability enhancements. Eur J Pharm Sci 48:775–80
- Elsayed MM, Abdallah OY, Naggar VF, et al. (2006). Deformable liposomes and ethosomes: mechanism of enhanced skin delivery. Int J Pharm 322:60–6
- Elsayed MM, Abdallah OY, Naggar VF, et al. (2007). Lipid vesicles for skin delivery of drugs: reviewing three decades of research. Int J Pharm 332:1–16
- Finn DA, Beadles-Bohling AS, Beckley EH, et al. (2006). A new look at the 5alpha-reductase inhibitor finasteride. Cns Drug Rev 12:53–76
- Gillet A, Compere P, Lecomte F, et al. (2011). Liposome surface charge influence on skin penetration behaviour. Int J Pharm 411:223–31
- Godin B, Touitou E. (2003). Ethosomes: new prospects in transdermal delivery. Crit Rev Ther Drug Carrier Syst 20:63–102
- Gupta M, Agrawal U, Vyas SP. (2012). Nanocarrier-based topical drug delivery for the treatment of skin diseases. Expert Opin Drug Deliv 9:783–804
- Heard CM, Monk BV, Modley AJ. (2003). Binding of primaquine to epidermal membranes and keratin. Int J Pharm 257:237–44
- Jun-Bo T, Zhuang-Qun Y, Xi-Jing H, et al. (2007). Effect of ethosomalminoxidil on dermal delivery and hair cycle of C57BL/6 mice. J DermatolSci 45:135–7
- Kuo TR, Wu CL, Hsu CT, et al. (2009). Chemical enhancer induced changes in the mechanisms of transdermal delivery of zinc oxide nanoparticles. Biomaterials 30:3002–8
- Li G, Fan Y, Fan C, et al. (2012). Tacrolimus-loaded ethosomes: physicochemical characterization and in vivo evaluation. Eur J Pharm Biopharm 82:49–57
- Libecco JF, Bergfeld WF. (2004). Finasteride in the treatment of alopecia. Expert Opin Pharmacother 5:933–40
- Liu S, Yamauchi H. (2008). Different patterns of 5alpha-reductase expression, cellular distribution, and testosterone metabolism in human follicular dermal papilla cells. Biochem Biophys Res Commun 368:858–64
- Pierre MB, Dos SMCI. (2011). Liposomal systems as drug delivery vehicles for dermal and transdermal applications. Arch Dermatol Res 303:607–21
- Qi LW, Li P, Li SL, et al. (2006). Screening and identification of permeable components in a combined prescription of DangguiBuxue decoction using a liposome equilibrium dialysis system followed by HPLC and LC-MS. J Sep Sci 29:2211–20
- Rao Y, Zheng F, Zhang X, et al. (2008). In vitro percutaneous permeation and skin accumulation of finasteride using vesicular ethosomal carriers. AAPS Pharm Sci Tech 9:860–5
- Schroeter A, Engelbrecht T, Neubert RH, et al. (2010). New nanosized technologies for dermal and transdermal drug delivery. A review. J Biomed Nanotechnol 6:511–28
- Shi J, Wang Y, Luo G. (2012). Ligustrazine phosphate ethosomes for treatment of Alzheimer's disease, in vitro and in animal model studies. AAPS Pharm Sci Tech 13:485–92
- Shumilov M, Bercovich R, Duchi S, et al. (2010). Ibuprofen transdermal ethosomal gel: characterization and efficiency in animal models. J Biomed Nanotechnol 6:569–76
- Touitou E, Dayan N, Bergelson L, et al. (2000). Ethosomes – novel vesicular carriers for enhanced delivery: characterization and skin penetration properties. J Control Release 65:403–18
- Touitou E. (1996). Compositions for applying active substances to or through the skin. US patent 5540934
- Traish AM, Hassani J, Guay AT, et al. (2011). Adverse side effects of 5alpha-reductase inhibitors therapy: persistent diminished libido and erectile dysfunction and depression in a subset of patients. J Sex Med 8:872–84
- Trueb RM. (2002). Molecular mechanisms of androgenetic alopecia. Exp Gerontol 37:981–90
- van der Maaden K, Jiskoot W, Bouwstra J. (2012). Microneedle technologies for (trans)dermal drug and vaccine delivery. J Control Release 161:645–55
- Verma P, Pathak K. (2012). Nanosizedethanolic vesicles loaded with econazole nitrate for the treatment of deep fungal infections through topical gel formulation. Nanomedicine 8:489–96
- Xu DH, Zhang Q, Feng X, et al. (2007). Synergistic effects of ethosomes and chemical enhancers on enhancement of naloxone permeation through human skin. Pharmazie 62:316–18
- Zhou Y, Wei Y, Liu H, et al. (2010). Preparation and in vitro evaluation of ethosomal total alkaloids of Sophoraalopecuroides loaded by a transmembrane pH-gradient method. AAPS Pharm Sci Tech 11:1350–8
- Zhou Y, Wei YH, Zhang GQ, et al. (2010). Synergistic penetration of ethosomes and lipophilic prodrug on the transdermal delivery of acyclovir. Arch Pharm Res 33:567–74