Abstract
Nanoemulsions were investigated as transdermal delivery systems for indomethacin. Six formulae were prepared using Triacetin, capryol 90 and labrafil as oils; Tween 80 and pluronic F127 as surfactants and transcutol and propylene glycol as co-surfactants. The continuous phase was that one with the larger volume fraction regardless of the hydrophile–lipophile balance of the surfactant/co-surfactant mixture. Surfactant type had significant effects on particle size and rheological properties of the nanoemulsions. Pluronic-based formulae recorded the lowest particle sizes and the highest viscosities. The prepared nanoemulsions increased drug solubility up to 610-fold compared with water. Refractive index measurements proved the compatibility between indomethacin and the used nanoemulsion components. Indomethacin was almost completely ionized at the pH values of the prepared nanoemulsions, suggesting drug absorption via the hydrophilic pathway of the skin upon topical application. Nanoemulsions controlled indomethacin release through semipermeable membrane and enhanced its permeation through excised newly born albino rat skin. The formulae were stable for six months at ambient conditions. Transdermal single application of selected formulae resulted in effective plasma levels up to 32 h in rats. Nanoemulsions were significantly superior to other investigated transdermal approaches at solubilizing indomethacin and achieving higher plasma levels.
Introduction
Indomethacin is a potent nonsteroidal anti-inflammatory and strong analgesic drug. It is used for the treatment of different inflammatory conditions such as rheumatoid arthritis, spondylosis deformans and acute gout syndrome. However, severe gastrointestinal side effects limit its use and its poor solubility requires special formulation techniques (Stephen et al., Citation1998; Kathiresan et al., Citation2010). The transdermal delivery of indomethacin has been extensively studied to avoid gastrointestinal and central nervous system-related side effects. These studies included the use of chemical penetration enhancers (Sasaki et al., Citation1991; Ogiso et al., Citation2006), design of prodrugs (Jona et al., Citation1995), complexation (Ito et al., 2001; Chauhan et al., Citation2003), driving drug molecules through the skin using iontophoresis (Kanebako et al., Citation2002) or pulsed output ultrasound (Miyazaki, 1997), formulating drug as polymeric films and patches (Rao & Diwan, Citation1998 and Li et al., Citation2007) or as cubosome dispersions (Esposito et al., Citation2005) as well as encapsulating the drug in self-microemulsifying and microemulsion systems (El Maghraby, Citation2010). Microemulsion is a thermodynamically stable, transparent, single optically isotropic liquid system of water, oil and surfactants. Microemulsions are proven to enhance transdermal delivery of both hydrophilic and hydrophobic drug molecules due to their thermodynamic stability, ease of formulation, low viscosity, high drug loading capacity and small droplet size (Hua et al., Citation2004). The potential of nanoemulsions as transdermal drug delivery for indomethacin has been evaluated by Shakeel et al. (Citation2009), but the study lacked in vivo evidence. The smaller particle size and the lower viscosity of nanoemulsions could be considered as additional advantages over microemulsions. This hypothesis needs to be investigated. Accordingly, the objective of this work was to formulate nanoemulsions as vehicles for transdermal delivery of indomethacin. The obtained results will be compared with the previously published work aiming to provide the pharmaceutical formulator with the necessary information about the efficacy of nanoemulsions in transdermal drug delivery. To achieve this objective, the study included nanoemulsion formulae with different compositions, particle sizes, phase behaviors and viscosities. Evaluation of the prepared systems included both in vitro and in vivo efficacy.
Methods
Materials
Indomethacin was purchased from Huasheng Drugs Manufacture Co. Ltd. (Shijiazhuang, China). Capryol 90 (propyleneglycol monocaprylic ester), Transcutol (diethyl-eneglycol monoethyl ether) and Labrafil (Oleoyl macroglycerides EP) were kindly provided by Gattefosse, Saint Priest, France. Poloxamer 407 (Pluronic F127) and acetonitrril (HPLC grade) were purchased from Sigma-Aldrich Chemical Company (St. Louis, MO). Propylene glycol and Tween 80 were obtained from El-Nasr Company, Cairo, Egypt. Triacetin (Glycerol triacetate) was kindly supplied by Reda Chemicals, Maadi, Cairo, Egypt. Farcomethacin gel, Pharco Co., Egypt.
Preparation of indomethacin-loaded nanoemulsions
The selection of different nanoemulsions was based on the previously published formulae and phase diagrams of Shakeel et al. (2007), Baboota et al. (Citation2007) and Ammar et al. (Citation2009). Six nanoemulsion formulae were prepared using triacetin, capryol 90 and labrafil as oil ingredients, Tween 80 and pluronic F127 as surfactants and transcutol and propylene glycol as co-surfactants. shows the composition of the prepared nanoemulsion formulae. Exactly 1% w/w of indomethacin was dissolved in the oil, surfactant and co-surfactant mixtures. Water was added and the whole system was vortexed for 2 min (Thermolyne Maxi Mix ΙΙ, model M 37610-26, USA).
Table 1. Composition and physicochemical evaluation parameters of the prepared nanoemulsion formulae.
Thermodynamic stability studies
The thermodynamic stability of the prepared nanoemulsion formulae was assessed by centrifugation at 3500 rpm for 30 min (Moreno et al., Citation2003). Heating/cooling cycles between 4 °C and 25 °C, as well as freeze–thaw cycles between −21 °C and 25 °C were also carried out (Shafiq et al., Citation2007). The formulae were examined for liquefaction and phase separation after each test.
Physicochemical characterization of the prepared nanoemulsions
Physicochemical characterization started with the determination of phase behavior. Drug-free and drug-loaded nanoemulsion formulae were titrated with water till the appearance of phase separation or milky appearance. In addition, the hydrophile–lipophile balance (HLB) values of the surfactant/co-surfactant mixtures were calculated.
Particle size distribution by intensity was determined using dynamic light scattering at 25 °C with detector at scattering angle of 173° to the incident beam (Zetasizer, Nanoseries, Malvern, UK) (Zhang et al., Citation2006).
The refractive index of drug-free and drug-loaded nanoemulsion formulae was determined at 25° (Abbe refractometer, Carl zeiss, Germany).
The viscosity of 1 g of each of the prepared formulae was determined using cone and plate Brookfield viscometer, model HBDV-I (Brookfield Engineering Laboratories, Inc., MA) at 25 °C and angular velocities ranging from 10 to 100 rpm with 30 s wait for each revolution per minute. Measurements were done in triplicate and mean viscosity values were plotted against angular velocities to determine the flow type.
The pH of different nanoemulsion formulae with and without the drug was determined at room temperature (PH-Meter, Jenway 3510, USA). The ratio of ionized and nonionized drug was calculated according to Henderson–Hasselbalch equation at the pH of each formula.
Solubility of indomethacin in the prepared nanoemulsions
An excess of indomethacin was added to screw-capped tubes containing 1 ml of prepared formulae. Tubes were shaken mechanically at 37 ± 0.5 °C for 72 h. Samples were centrifuged at 3000 rpm for 5 min. Excess insoluble drug was discarded by filtration using a 0.45-µm membrane filter (Whatman, USA). The concentration of indomethacin was quantified by high-performance liquid chromatography (HPLC) following a slight modification of the method reported by Goosen et al. (Citation1998). HPLC analyses were performed using a Shimadzu apparatus (10A Vp, Kyoto, Japan). Elution was conducted with a mobile phase constituted of a mixture of acetonitrile/water/acetic acid (50:46:4) at a flow rate of 1.5 ml/min. Indomethacin was detected at a wavelength of 254 nm using a UV detector. Solubility experiment was conducted in triplicate.
In vitro release studies
In vitro release was determined using USP II dissolution basket assembly (Hanson Research Dissolution tester, Chatsworth, CA), following the procedure described by Badawi et al. (Citation2008). One gram indomethacin-loaded nanoemulsion was placed in glass cylindrical tubes (3.14 cm in diameter and 10 cm in length) tightly covered with cellulose membrane as permeation barrier from one end (Spectrapore membrane tubing No.2, Spectrum Medical Industries, SC). The tubes were attached to the shaft of USP dissolution tester and immersed in 50 ml of phosphate buffer pH 7.4 as release medium. The study was carried out at 37 ± 0.5 °C and paddle speed of 50 rpm. At definite time intervals, 3 ml samples were withdrawn and analyzed spectrophotometrically for indomethacin content at λmax 319 nm.
The release extent was determined by measuring the release efficiency after 6 h (RE6h) according to Khan (Citation1975).
The release kinetics from the prepared formulae were investigated by fitting the release data to zero order, first order and Higuchi’s models and applying linear regression analysis. For further evaluation of the drug release mechanism, Korsmeyer–Peppas model (Korsmeyer et al., Citation1983) was applied.
Where Mt/M∞ is the fraction of drug released at time t, k is the kinetic release constant and n is an exponent that characterize the release mechanism. If the n value is ≤0.5, the release mechanism follows Fickian diffusion and higher values 0.5 < n < 1 for mass transfer follows a non-Fickian model (anomalous transport). The drug release follows zero-order drug release and case-II transport if the n value is 1. For the values of n > 1, the mechanism of drug release is regarded as super case-II transport.
In vitro skin permeation studies
In vitro skin permeation studies were performed using a modified diffusion cell with exposed diffusional area of 3.14 cm2 and 50 ml receiver chamber capacity. Full-thickness hairless albino rat skin was used as skin membrane (Fang et al., Citation1999). Newly born albino rats (2- to 3-d age) were subjected to cervical dislocation and full-thickness skin was excised. The subcutaneous tissue was surgically removed and the skin was examined under a magnifying lens for any damage or disease. The study protocol was approved by the Research Ethical Committee of Faculty of Pharmacy, Cairo University.
The skin was soaked for 2 h in phosphate buffer pH 7.4, washed with distilled water and stored at −21 °C till use. The skin was brought to room temperature before use and was mounted between donor and receiver compartments of the diffusion cell with the epidermal surface facing the donor compartment. The receiver chamber was filled with phosphate buffer pH 7.4 and magnetically stirred at 50 rpm. The assembled diffusion cell was kept at a constant temperature of 37 ± 0.5 °C. Accurately weighed 1 g of each indomethacin-loaded formula was placed in one donor compartment. Samples were withdrawn at predetermined time intervals up to 6 h, filtered through a 0.45 µm membrane and assayed by HPLC. An equal volume of phosphate buffer pH 7.4 was added to maintain constant volume.
Cumulative amount of indomethacin permeated through the skin (µg/cm2) was plotted as a function of time for each formula. Rate of drug permeation at steady state (Jss) was determined from the slope of the linear portion of the graph (Shakeel & Ramadan, Citation2010). Permeability coefficient (KP) was calculated by dividing Jss by initial concentration of the drug in the donor compartment (Co).
Permeation data was subjected to statistical analysis using one way analysis of variance (ANOVA) and Tukey multiple comparison tests. Statistical differences yielding p ≤ 0.05 were considered significant.
Stability studies
The six prepared drug loaded nanoemulsion formulae were stored in sealed containers at ambient conditions of temperature and humidity and were re-evaluated after six months for their physical appearance, particle size, refractive index and indomethacin content.
In vivo evaluation
Twenty-four male albino rats weighing between 150 and 200 g were divided randomly into four groups each receiving one formula, namely, nanoemulsion formula B, nanoemulsion formula C, marketed indomethacin gel (Farcomethacin gel) and a control group. The animals were housed in poly propylene cages under standard laboratory conditions (25 ± 1 °C and 55 ± 5% relative humidity) with free access to standard laboratory diet and water. The animals were anesthetized with IM injections of ketamine hydrochloride 90 mg/kg and xyloject 10 mg/kg. Hairs at the dorsal surface were shaved off and the skin area was washed with distilled water the day before the experiment, except for the control group. Nanoemulsion formulae and marketed gel (100 mg/kg) were applied on the skin surface (15 cm2), then the rats were housed in individual containers to restrict movement and prevent loss of the applied formulae.
Blood samples were withdrawn from the eye vein using heparinated capillary tubes at hours 0, 2, 4, 6, 12, 24, 28 and 32 after transdermal application. Samples were immediately transferred in heparinated veneject tubes and centrifuged at 4000 rpm for 10 min. Plasma proteins were precipitated by adding 1 ml acetonitrile and vortexing for 1 min twice. A 20-µl aliquot of the supernatant was injected onto the HPLC column for analysis. The study protocol was approved by the Research Ethical Committee of Faculty of Pharmacy, Cairo University.
The area under curve (AUC0–32), maximum plasma concentration (Cmax) and time for maximum plasma concentration (Tmax) were calculated for each formula and compared statistically using one-way ANOVA test at p < 0.05.
Results and discussion
Preparation of indomethacin-loaded nanoemulsions
Six nanoemulsion formulae with the compositions presented in were prepared and subjected to thermodynamic stability studies. No liquefaction or phase separation was recorded after centrifugation and cooling–heating cycles. Slight turbidity was recorded during storing at −21 °C during the freeze–thaw cycles. This turbidity disappeared by storing the formulae at ambient temperature. These results confirmed thermodynamic stability of the selected formulae and suggested their use as transdermal delivery systems for indomethacin.
Physicochemical characterization of the nanoemulsions
An appropriate physicochemical characterization of colloidal formulations is highly challenging due to their small particle sizes and their fluctuating interfaces. The combination of different characterization techniques is strongly required.
For the determination of phase behavior and type of nanoemulsion (nanoemulsion microstructure), drug-free and drug-loaded formulae were titrated with water and the HLB values of their surfactant/co-surfactant mixtures were calculated. Nanoemulsion formulae A and B that contain higher water percentages (40% and 32% w/w, respectively) accepted dilution without any phase separation suggesting nanoemulsion with droplet-like structure of o/w type. Drug-loaded formulae A and B showed the same behavior on dilution in addition to slight bluish turbidity, probably due to the poor solubility of indomethacin in water. This result was in agreement with the previously stated observations for nanoemulsion formulae with the same composition (Baboota et al., Citation2007; Shakeel et al., Citation2007).
On the other hand, dilution of oil-rich formulae (formula C, E and F containing 30% w/w triacetin oil) resulted in spontaneous phase separation indicating w/o nanoemulsions. Upon dilution with water, nanoemulsion formula C showed separation of oil globules. Pluronic-based formulae E and F showed rapid formation of white gel-like mass. This mass was not redispersible on shaking and turned to waxy precipitate on the tube’s walls.
Nanoemulsion formula H with equal oil and water amounts gave rise to initial turbidity that decreased upon further dilution and finally disappeared. The same response occurred with the formula H loaded with drug. This formula could be described as bicontinous phase where both water and oil exist as a continuous phase in the presence of a continuously fluctuating surfactant-stabilized interface with a net curvature of zero. Another support for the formation of bicontinuous structure is the large amount of surfactant/co-surfactant mixture (60% w/w) in formula H that is necessary to cover the interface (Strey, Citation1994; Heuschkel et al., Citation2008).
The HLB values of the surfactant/co-surfactant mixtures for the selected formulae were calculated and presented in . The HLB values ranged from 9.6 to 18. Although it is generally accepted that surfactants with low HLB values (3–6) favor the formation of w/o nanoemulsions, high HLB values (8–18) result in o/w nanoemulsion systems (Gradzielski et al., Citation1996). Yet, it is clear that the HLB does not adequately rationalize the phase behavior of the six prepared nanoemulsions. This could be attributed to the use of oily components with different degrees of polarity (Triacetin, Capryol 90 and labrafil).
The critical packing parameter (CPP), which is a measure of the preferred geometrical structure adopted by the surfactant, would be considered as additional factor affecting surfactant behavior. Changes in nanoemulsion composition can also modify the microenvironment of the surfactant leading to changes in its apparent CPP (Lawrencea & Rees, Citation2000).
Particle size analysis revealed that all nanoemulsion formulae had monomodal size distribution with polydispersity values between 0.022 and 0.294. The Z-average globule size mean values ranged from 40.4 to 130.8 nm except for Formulae E and F, which recorded smaller particle sizes (15.0 and 4.4 nm, respectively; ). This marked decrease in particle size of those two formulae could be due to the presence of Pluronic surfactant. Similarly, Sharma and Bhatia (Citation2004) stated that hydrophobic drugs, such as indomethacin, could decrease the aggregation numbers of Pluronic molecules leading to a decrease in micelles’ size and in boundaries in between liquid gel and gel liquid.
Formula H recorded the greatest particle size among all the prepared nanoemulsions (131.8 nm). This could be attributed to its high surfactant/co-surfactant mixture content (60%). A similar result was reported by Wang et al. (Citation2009), who stated that the increase in surfactant concentration above 50% leads to an increase in nanoemulsion particle size.
Particle size analysis supported the previously suggested phase behavior of the prepared nanoemulsions. Where, o/w nanoemulsions (formulae A and B) showed higher average particle sizes (101–110 nm) than w/o ones (formulae C, E and F) (4–40 nm). The effect of emulsion type on the size of the formed globules was explained by Lawrencea & Rees (Citation2000). The same number of surfactant molecules shows different orientation according to the type of continuous phase. For o/w nanoemulsions with surfactant heads extending outward into a continuous aqueous phase, a greater effective particle size occurs due to the hydration shell associated with the polar head groups and the steric hindrance due to the predominant repulsive effect between them. However, arrangement of surfactant molecules is easier at interfaces with high curvatures (smaller droplets). The surfactant tails will extend outward into a continuous oil phase. This is also entropically more favorable as the hydrocarbon tails have more directional freedom.
The values of the refractive index of drug-free formulae ranged from 1.406 to 1.4428. No remarkable differences were recorded on drug loading (values for drug-loaded nanoemulsions ranged from 1.4129 to 1.4343). This result excludes interactions between the drug and the used nanoemulsion components.
Rheological characterization of drug-free nanoemulsions showed that all formulae were of liquid consistency except formula E which was gel-like. The viscosity values at 30 rpm for all formulae are listed in . The gel-like behavior of formula E could be attributed to its concentrated high percentage of pluronic (33.3% w/w). Pluronic solutions show reverse thermal gelation (they are liquid at refrigerated temperatures and gel at room temperature). At low temperatures in aqueous solutions, a hydration layer surrounds Pluronic molecules. However, when the temperature is raised, the hydrophilic chains of the copolymer become desolvated as a result of the breakage of the hydrogen bonds that had been established between the solvent and these chains. This phenomenon favors hydrophobic interactions between the polyoxypropylene groups and leads to gel formation. It is thought that the gel is micellar in nature. A liquid micellar phase is stable at low temperatures but transforms into the cubic structure by increasing the temperature.
All formulae, except formula E, showed Newtonian flow (determination coefficients (Rxy) between shear rate and shear stress were close to 1). Formula E showed a decrease in viscosity upon increasing rpm (shear thinning behavior). Drug loading into nanoemulsions didn’t change the rheological properties of any of the six prepared formulae.
The pH values of drug-free nanoemulsions ranged from 6.23 to 7.39. Indomethacin incorporation lowered the pH values due to its acidic nature. shows that the pH values of drug-loaded formulae ranged from 5.92 to 6.23. Indomethacin (pKa = 4.5) was almost totally ionized in the pH range of the prepared formulae (percent ionized species ranged from 96 to 98). These results suggest the absorption of the drug via the hydrophilic pathway of the skin upon topical application. Similarly, Hayashi et al. (Citation1992) proved that the permeation of ionized species greatly contributed to the total permeation of indomethacin at higher pH, and that the total permeation rate of indomethacin was determined by the permeation of unionized species at lower pH.
Solubility of indomethacin in the prepared nanoemulsions
The calibration curve of indomethacin was linear between 20 and 3000 ng/ml with determination coefficient of 0.999. Solubility of indomethacin in the nanoemulsion formulae is shown in . Indomethacin solubility in nanoemulsions increased by 166- to 610-fold compared to its solubility in water. This was related to solubilizing power of the formulation components that implies the possibility of preparing supersaturated solutions from these systems. In addition, the high loading capacity of the colloidal formulations can be attributed to the unique structural organization of the micellar and nanoemulsion systems that can add solubility regions. The highest drug solubility was recorded in nanoemulsion formula with the lowest water content and vice versa. Moreover, the solubility of indomethacin was greater in w/o nanoemulsions than in o/w ones. This could be attributed to the lipophilic nature of the drug. The obtained results prove the great solubilizing power of the prepared nanoemulsions in comparison with other stated solubilization techniques, where a maximum solubility of 12 960 µg/ml was obtained in Formula C. Jona et al. (Citation1995) achieved maximum indomethacin solubility of 13.8 µg/ml in phosphate buffer pH 7.4 (4.76-fold enhancement) by synthesis of a series of indomethacin esters containing tertiary amine functional groups (pro-drugs). Complexation of indomethacin with polyamidoamine dendrimers resulted in maximum drug solubility of 700 µg/ml at pH 7 (Chauhan et al., Citation2003). El Maghraby (Citation2010) achieved solubility enhancement up to 30.25-fold by incorporation in self-microemulsifying and microemulsion systems composed of Span 20 and Tween 80 with ethyl oleate as oil component.
In vitro release studies
The release profiles of indomethacin from the prepared nanoemulsions through cellulose membrane are depicted in and the release efficiency values after 6 h (RE6h) are listed in . The nanoemulsion formulae could be arranged in a descending order according to their RE6h values as B > C > F > H > E > A. No clear relation could be established between the release efficiency of all formulae and the nanoemulsion composition or any of the nanoemulsion characteristics, such as particle size, phase behavior or viscosity. This could be attributed to the rapid diffusion of water molecules through cellulose membrane during the release study. This rapid diffusion of water could lead to dilution of formulae and consequently phase transition and/or phase separation. Similarly, Trotta (Citation1999) found that rate of indomethacin release from some microemulsion formulae was dependent on the size of the disperse phase after dilution with the release medium.
Figure 1. In-vitro release profiles of indomethacin from different nanoemulsion formulae through cellulose membrane. Formulae compositions are shown in .
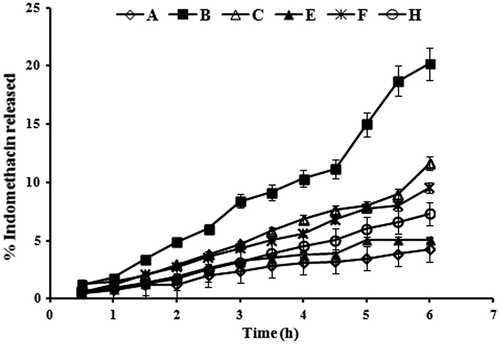
Table 2. Results of in vitro release and skin permeation studies of indomethacin from the prepared nanoemulsions.
Fitting the release data to the different kinetic models showed that all formulae followed zero order kinetics. This finding was confirmed by calculating the exponent (n) in Krosmear Peppas equation, which ranged from 0.9 to 1.1 ().
In vitro skin permeation studies
In vitro skin permeation studies are usually used to provide a correlation with biological response as the major limitation to transdermal drug delivery is the skin itself.
The drug permeation of indomethacin from all the prepared formulae had a linear release profile after a lag period of 1 h (). The drug flux at steady state (Jss) and the permeation coefficient (Kp) values are shown in . Permeation of the prepared formulae could be arranged in descending order as A > E > H > B > F > C. The highest drug permeation through rat skin was recorded for formulae with the lowest drug release efficiencies through cellulose membrane. This could be attributed to the different nature of the barrier in both experiments [cellulose membrane is hydrophilic, whereas skin has a dual nature and dual pathway for passage of solutes (Hayashi et al., Citation1992)].
Figure 2. Indomethacin transdermal permeation profiles through excised newly born albino rat skin. Formulae compositions are shown in .
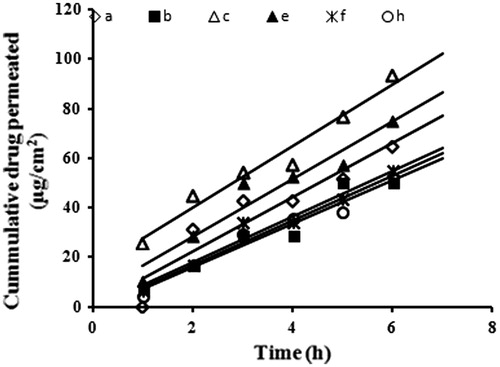
Statistical analysis showed that no significant differences were found between different formulae except for the two formulae with the highest and lowest mean values only (formulae A and C). This proves that neither the particle size nor viscosity of the prepared nanoemulsions had a significant effect on their efficiency. This could be explained by the small droplet sizes and the low viscosity of all formulae which made them all excellent carriers for enhancing percutaneous uptake of indomethacin. The number of vesicles that can interact on a fixed area of stratum corneum increases when droplet size and viscosity decrease.
The superiority of the prepared nanoemulsions as transdermal drug delivery systems for indomethacin can be easily figured by comparing the obtained indomethacin flux values (5.79–55.81 µg/cm2/h) to other transdermal drug delivery systems of indomethacin. Li et al. (Citation2007) formulated indomethacin as transdermal patches and achieved drug flux values of 9.6, 13.2 and 27.7 µg/cm2/h for enhancer-free patch, patch with 7% azon and patch with 5% azone + 5% (2-isopropyl-5-methylcyclohexyl heptanoate), respectively. Transdermal indomethacin flux ranging from 4.97 to 19.86 µg/cm2/h was obtained by incorporating the drug in self-microemulsifying system and microemulsions (El Maghraby, Citation2010).
Dermally applied nanoemulsions enhance the percutaneous uptake of drugs by altering its lipid and polar pathways of penetration. The lipid domain of nanoemulsions destabilizes the bilayer structure of the stratum corneum with consequent increase in the lipid pathway permeability to drugs. On the other hand, the hydrophilic domain of nanoemulsions hydrates the stratum corneum and increases the interlamellar volume of its lipid bilayer, resulting in disruption of its interfacial structure (Baboota et al., Citation2007).
An inverse correlation could be figured out between the drug solubility in the nanoemulsion formula and its permeation rate (Jss) from this formula (). Slower permeation rates were observed from formulae with higher affinities to the drug. This suggests that in addition to penetration enhancing effect of nanoemulsion composition, the transdermal delivery of indomethacin was also a function of its release rate from its base.
Stability studies
Visual examination of the stored drug-loaded formulae showed no signs of phase separation, discoloration or precipitation. No significant differences were recorded between the average particle sizes of the fresh and six months stored nanoemulsion indicating good physical stability (). Comparing the refractive index values of fresh and six months stored formulae revealed no remarkable changes and reflected the chemical stability of the prepared nanoemulsions. Indomethacin content ranged from 95.9% to 98.4% for all formulae.
Table 3. Results of stability study of indomethacin-loaded nanoemulsion formulae at ambient conditions after six months.
In vivo evaluation studies
The aforementioned in vitro evaluations proved that all the tested nanoemulsion formulae had acceptable particle sizes, pH values and viscosities for transdermal application. No significant differences were recorded between the six formulae concerning their in vitro release profiles and their permeation enhancement effect through excised skin. All showed good physical and chemical stability. Thus, two formulae B and C were selected for further in vivo evaluation as examples for hydrophilic and lipophilic formulae, respectively.
illustrates the plasma concentration of indomethacin after topical application of a single dose of selected nanoemulsion formulae and the commercial indomethacin gel (Farcomethacin). Indomethacin plasma concentration ranged from 1.37 to 2.02 µg/ml for formula B and from 1.07 to 2.12 µg/ml for formula C. The plasma concentration of all indomethacin formulae remained within the therapeutic range for at least 32 h. Indomethacin monograph (medscape.com) has been suggested 0.5–3 µg/ml as therapeutic range. The permeation enhancement effect of drug-loaded nanoemulsions was comparable with the results of Wang et al. (Citation2008). He reported an indomethacin plasma concentration in range of 1.15–2.15 µg/ml after application of indomethacin in citrate phosphate buffer pH 6.5 in combination to applying iontophoresis and electroporation as physical enhancers. Moreover, the obtained drug plasma concentrations after topical application exceeded the maximum plasma level obtained after single oral administration of indomethacin-loaded self-nanoemulsifying formula in rats (0.13 µg/ml) (Taha et al., 2009).
Surprisingly, the tested commercial gel, which is intended for topical use, resulted in plasma levels similar to those achieved by transdermal formulations. This finding can be related to its hydroalcoholic base (product leaflet).The alcohol acts as permeation enhancer. In addition, alcohol volatilization at body temperature results in supersaturated solution of indomethacin on the skin surface. Supersaturation increases the concentration gradient and hence drug permeation. Statistical analysis of the mean AUC0–32 values using one way ANOVA revealed no significant differences between the three tested formulae at p < 0.05. This result gives an alarm and necessitates further investigations on larger numbers of experimental animals and on humans to regulate the use of hydroalcoholic-based products for local use.
The plasma drug concentration curves of the three tested products exhibited three peaks at 2, 12 and 28 h. This finding augments the postulation proposed by Goosen et al. (Citation1998), who suggested that indomethacin may form reservoir in the stratum corneum due to its lipophilic nature. One way ANOVA was applied to compare the obtained maximum plasma concentrations (Cmax) at the three peeks of each curve and the results revealed no significant difference at p < 0.05. So the value of Cmax for nanoemulsion formulae B, C and the commercial gel were in the range of (1.7–2.2 µg/ml), (1.69–2.12 µg/ml) and (1.56–1.94 µg/ml), respectively. Tmax was considered as 2 h.
Conclusion
Nanoemulsions are promising candidates for enhanced transdermal drug delivery system. Their smaller particle size can provide drug delivery system with superior transdermal drug delivery enhancement compared with the microemulsion. The study highlighted on the possible transdermal effect for hydroalcoholic gels intended for local effect and suggested the need for detailed investigation on this. The results proved a prolonged systemic effect (up to 32 h) after single topical application which suggests further in vivo studies to optimize the dose and dose regimen of transdermal indomethacin.
Acknowledgements
This research received no specific grant from any funding agency in the public, commercial or not-for-profit sectors.
Declaration of Interest
The authors report no conflicts of interest. The authors alone are responsible for the content and writing of this work.
References
- Ammar HO, Salama HA, Ghorab M, Mahmoud AA. (2009). Nanoemulsion as a potential ophthalmic delivery system for dorzolamide hydrochloride. AAPS PharmasciTech 10:808–19
- Baboota S, Shakeel F, Ahuja A, et al. (2007). Design, development and evaluation of novel nanoemulsion formulations for transdermal potential of Celecoxib. Acta Pharm 57:315–32
- Badawi AA, El-Laithy HM, El Qidra RK, et al. (2008). Chitosan based nanocarriers for indomethacin ocular delivery. Arch Pharm Res 31:1040–9
- Chauhan AS, Sridevi S, Chalasani KB. (2003). Dendrimer-mediated transdermal delivery: enhanced bioavailability of indomethacin. J Control Release 90:335–43
- El Maghraby GM. (2010). Self-microemulsifying and microemulsion systems for transdermal delivery of indomethacin: Effect of phase transition. Colloids Surfaces B Biointerfaces 75:595–600
- Esposito E, Cortesi R, Drechsler M, et al. (2005). Cubosome dispersions as delivery systems for percutaneous administration of indomethacin. Pharm Res 22:2163–73
- Fang J, Sung KC, Lin H, Fang C. (1999). Transdermal iontophoretic delivery of diclophenac sodium from various polymer formulations: in vitro and in vivo studies. Int J Pharm 178:83–92
- Goosen C, Plessis JD, Müller DG, Janse van Rensburg LF. (1998). Correlation between physicochemical characteristics, pharmacokinetic properties and transdermal absorption of NSAID’s. Int J Pharm 163:203–9
- Gradzielski M, Langevin D, Parago B. (1996). Experimental investigation of the structure of nonionic microemulsions and their relation to the bending elasticity of the amphiphilic film. Prog Colloid Polym Sci 100:162–9
- Hayashi T, Sugibayashi K, Morimoto Y. (1992). Calculation of skin permeability coefficient for ionized and unionized species of Indomethacin. Chem pharm Bull 40:3090–3
- Heuschkel S, Goebel A, Neubert RHH. (2008). Microemulsions—modern colloidal carrier for dermal and transdermal drug delivery. J Pharm Sci 97:603–31
- Hua L, Weisan P, Jiayu L, Ying Z. (2004). Preparation, evaluation, and NMR characterization of vinpocetine microemulsion for transdermal delivery. D Dev Ind Pharm 30:657–66
- Ito T, Sugafuji T, Maruyama M, et al. (2001). Skin penetration by indomethacin is enhanced by use of an indomethacin/smectite complex. J Supramol Chem 1:217–9
- Jona JA, Dittert LW, Crooks PA, et al. (1995). Design of novel prodrugs for the enhancement of the transdermal penetration of indomethacin. Int J Pharm 123:127–30
- Kanebako M, Inagi AT, Takayamab K. (2002). Transdermal delivery of indomethacin by iontophoresis. Biol Pharm Bull 25:779–82
- Kathiresan K, Kiran K, Vijin P, et al. (2010). Formulation and development of indomethacin sustained release tablets. Int J PharmTech Res 2:794–7
- Khan KA. (1975). The concept of dissolution efficiency. J Pharm Pharmacol 27:48–9
- Korsmeyer RW, Gurny R, Doelker E, et al. (1983). Mechanisms of solute release from porous hydrophilic polymers. Int J Pharm 15:25–35
- Lawrencea MJ, Rees JD. (2000). Microemulsion-based media as novel drug delivery systems. Adv Drug Deliv Rev 45:89–121
- Li T, Ren C, Wang M, et al. (2007). Optimized preparation and evaluation of indomethacin transdermal patch. Asian J Pharm Sci 2:249–59
- Miyazaki S. (1997). Effect of pulsed output ultrasound on the transdermal absorption of indomethacin from an ointment in rats. Biol Pharm Bull 20:288–91
- Moreno MA, Ballesteros MP, Frutos P. (2003). Lecithin–based oil-in-water microemulsion for parenteral use: pseudoternary phase diagrams, characterization and toxicity studies. J Pharm Sci 92:1428–37
- Ogiso T, Iwaki M, Paku T. (2006). Effect of various enhancers on transdermal penetration of indomethacin and urea, and relationship between penetration parameters and enhancement factors. J Pharm Sci 84:482–8
- Rao PR, Diwan PV. (1998). Formulation and in vitro evaluation of polymeric films of diltiazem hydrochloride and indomethacin for transdermal administration. D Dev Ind Pharm 24:327–36
- Sasaki H, Kojima M, Mori Y, et al. (1991). Enhancing effect of pyrrolidone derivatives on transdermal penetration of 5-fluorouracil, triamcinolone acetonide, indomethacin, and flurbiprofen. J Pharm Sci 80:533–8
- Shafiq S, Shakeel F, Talegaonkar S, et al. (2007). Development and bioavailability assessment of ramipril nanoemulsion formulation. Eur J Pharm Biopharm 66:227–43
- Shakeel F, Baboota S, Ahuja A, et al. (2007). Nanoemulsions as vehicles for transdermal delivery of aceclofenac. AAPS PharmSciTech 8:E104
- Shakeel F, Ramadan W. (2010). Transdermal delivery of anticancer drug caffeine from water-in-oil nanoemulsions. Colloids Surfaces B Biointerfaces 75:356–62
- Shakeel F, Ramadan W, Ahmed MA. (2009). Investigation of true nanoemulsions for transdermal potential of indomethacin: characterization, rheological characteristics, and ex vivo skin permeation studies. J Drug Targeting 17:435–41
- Sharma PK, Bhatia SR. (2004). Effect of anti-inflammatories on pluronic F127: micellar assembly, gelation and partitioning. Int J Pharm 278:360--77
- Stephen A, Mikulak C, Thomas V. (1998). Transdermal delivery and accumulation of indomethacin in subcutaneous tissues in rats. J Pharm Pharmacol 50:153–8
- Strey R. (1994). Microemulsion microstructure and interfacial curvature. Colloid Polym Sci 272:1005–19
- Taha E, Al-Suwayeh SA, El-Badry M. (2009). Bioavailability study of indomethacin self-nanoemulsifying oral formulation in rats. Australian J Basic Appl Sci 3:2944–8
- Trotta M. (1999). Influence of phase transformation on indomethacin release from microemulsions. J Cont Rel 60:399–405
- Wang L, Dong J, Chen J, et al. (2009). Design and optimization of a new self-nanoemulsifying drug delivery system. J Colloid Interface Sci 330:443–8
- Wang RJ, Wu PC, Ang YBH, Tsai YH. (2008). The effects of iontophoresis and electroporation on transdermal delivery of meloxicam salts evaluated in vitro and in vivo. J Food Drug Anal 16:41–8
- Zhang XG, Dong J-F, Zhang YG, et al. (2006). The effect of organic salts on the conductance of the water/AOT/alkanol w/o microemulsion. Acta Phys-Chim Sin 22:22–7