Abstract
Enhancing the deposition and permeation of 5-fluorouracil across human epidermis assisted by appropriately charged and well-defined peptide dendrimers was investigated. Peptide dendrimers with arginine as the terminal amino acid and having a range of terminal positive charges (4+, 8+ and 16+) were synthesized by solid phase peptide synthesis. Various parameters including effect of peptide dendrimers on the solubility and partition coefficient of 5-FU, degradation of drug in skin as well as deposition and permeation of 5-FU in/through skin were studied. All the tested dendrimers increased the aqueous solubility and partition coefficient of 5-FU with each also significantly (p < 0.05) enhancing the deposition and permeation of 5-FU in/across human epidermis in a concentration-dependent manner. Of the three peptide dendrimers examined, R8 dendrimer (bearing 8+ charge derived from four terminal arginines and MW of ≈1000 Da) showed greatest values for flux, Q48 (cumulative amount of drug permeated at the end of 48 h) and amount of drug retained in human skin. Furthermore, this study also scrutinized and reports on the likely mechanisms by which peptide dendrimers act as transdermal permeation enhancers.
Introduction
In recent years, there has been increasing interest and drive toward deriving practical and reliable approaches toward skin-mediated delivery of drugs, for both local and systemic therapies. Effective transdermal drug delivery offers many advantages over conventional routes of administration as it avoids first-pass metabolism, provides controlled and constant administration of drug while eliminating pulsed entry into the systemic circulation, which can often result in undesirable side effects. Furthermore, compared to parenteral and oral routes of administration, it has been shown to greatly improve patient compliance rates and, unlike the aforementioned routes, locally applied skin delivery devices can be halted with ease, by simple removal of the device when required (Chien, Citation1987). The effective and reliable delivery of drugs, however, presents an entirely new set of challenges, which are stringently controlled, albeit less so than oral/parental dosage forms, by regulatory bodies such as the US Food and Drug Administration (US-FDA). To meet the criteria for reliable and reproducible skin-mediated drug delivery, a range of approaches and enhancement techniques, primarily aimed at disrupting the formidable barrier effect of the (epi)dermal layer, have been proposed, and these are typically physical or chemical in origin (Howard & Kenneth, Citation1996; Benson, Citation2005; Ritesh & Anil, Citation2007; Tiwary et al., Citation2007; Wang et al., Citation2007; Nikhil et al., Citation2008; Dhamecha et al., Citation2009; Pathan & Setty, Citation2009; Kumar et al., Citation2010; Madishetti et al., Citation2010; Sharma et al., Citation2011). The physical techniques primarily include iontophoresis, sonophoresis and laser-based treatments, all of which are laborious, require specialized equipment and there are also cases of irreversible skin damage being attributed to such approaches (Meidan et al., Citation1999; Fang et al., Citation2004; Brahma & Shyam, Citation2008; Clara et al., Citation2008).
Chemical approaches and in particular “chemical enhancers” are considered by far the most cost-effective and convenient means of achieving transdermal permeation of drugs, with efficiencies on par with the key physical enhancement techniques outlined above. However, the vast majority of chemical enhancers employed in transdermal (drug) delivery to-date have not been without their considerable drawbacks, with a range of unacceptable side effects such as skin irritation, erythema and edema having been widely reported (Raja et al., Citation1998; Pathan & Setty, Citation2009). Hence, the availability of safe and efficacious permeation enhancing systems, able to reliably and reproducibly deliver therapeutic agents across human skin, are urgently sought. To this end, a handful of reports describing the application of highly branched molecules termed “dendrimers” have been reported in the literature. Dendrimers possess a range of attractive features such as multi-valency, nano-size, spherical architecture, surface-functional groups, low polydispersity and precise molecular weight, all of which render them versatile vectors in drug delivery (El-Sayed et al., Citation2002; Kannan et al., Citation2005; Vandamme & Brobeck Citation2005; Bai & Ahsan, Citation2009; Menjoge et al., Citation2010). Of the few reports available on the application of dendrimers for skin-mediated delivery of therapeutics, poly(amidoamine) (PAMAM) dendrimers have been among the most widely reported. Although PAMAM dendrimers have demonstrated potential in the delivery of a range of drugs such as riboflavin, tamsulosin, indomethacin, ketoprofen, diflunisal and 5-fluorouracil, toxicity issues remain an important concern and impediment in their broader use and applicability (Chauhan et al., Citation2003; Wang et al., Citation2003; Na et al., Citation2006; Parekh, Citation2007; Venuganti & Perumal, Citation2008; Filipowicz & Wolowiec, Citation2011).
In contrast, peptide dendrimers possess many notable advantages over and above previously reported PAMAM dendrimers including negligible toxicity and biodegradation to harmless endogenous amino acids. Peptide dendrimers are wedge-like molecules comprising an amino acid branching core that is most typically decorated with various basic amino acids (e.g. Lys, His, Arg) on their head groups (Cloninger, Citation2002; Niederhafner et al., Citation2005). With respect to the application of peptide dendrimers in transdermal delivery, we have demonstrated the successful application of ultrasound and iontophoresis toward the deposition/permeation of synthesized peptide dendrimers in/across human skin (Mutalik et al., Citation2009a,Citationb, Citation2012, Citation2013). Upon establishing the fate of peptide dendrimers following passive diffusion as well as assessing the effects of sonophoresis and iontophoresis on the skin delivery of peptide dendrimers, we moved our attention to testing their applicability in enhancing the transdermal permeation of therapeutically relevant molecules, where no reports are presently available in the literature. In this regard, and to test whether our peptide dendrimers possessed permeation enhancing properties, we selected the relevant model drug, 5-fluorouracil (5-FU) for use in skin-based studies. 5-FU is an anticancer agent used in the treatment of skin, breast and gastrointestinal cancers, while also showing efficacy in the topical treatment of psoriasis, premalignant and malignant skin conditions (Tsuji & Sugai, Citation1975; Goette, Citation1981; Diasio & Harris, Citation1989). Separately, there are also a few reports on the use of chemicals to enhance the skin permeation of 5-FU. Here, azone, dodecyl N, N-dimethylamino isopropionate (DDAIP), dodecyl N, N-dimethylamino acetate (DDAA) and tetrahydrogeraniol are among some of the agents trialed and demonstrating marked enhancements in the permeation of 5-FU across shed snake skin (Elka & Lizette, Citation1985; Marjukka et al., Citation1993; Raja et al., Citation1998). Topical formulations of 5-FU are considered even less effective, this being attributed to the poor permeability of 5-FU through the stratum corneum (Brahma & Shyam, Citation2008). Hence, this study was undertaken with the primary objective of assessing the effect and primary mechanism underlying peptide dendrimer-assisted permeation of 5-FU across human epidermis.
Materials and methods
Materials
Fmoc amino acids (Fmoc-Gly-OH, Fmoc-Lys(Fmoc)-OH, Fmoc-Arg(Pbf)-OH, O-(1H-benzotriazol-1-yl)-1,1,3,3-tetramethyluroniumhexafluorophosphate (HBTU) and Rink amide resin (0.70 mmol/g) were obtained from Merck biosciences, Darmstadt, Germany. Acetonitrile, dichloromethane (DCM) and N,N-dimethylformamide (DMF) were purchased from RCI Labscan, Samutsakorn, Thailand. 5-Fluorouracil (5-FU), trifluoroacetic acid (TFA), N,N-diisopropylethylamine (DIEA), triisopropyl silane (TIPS), HEPES, piperidine and diethyl ether were purchased from Sigma-Aldrich, St. Louis, MO, USA. All other chemicals used were of analytical grade.
Synthesis of peptide dendrimers
A panel of arginine-terminated peptide dendrimers of varying positive charge (R4: 4+ charge, C-to-N sequence Gly-Lys-(Arg)2, [M + H]+ of 515.8; R8: 8+ charge, C-to-N sequence Gly-Lys-(Lys)2-(Arg)4, [M + H]+ of 1084.4; R16: 16+ charge, C-to-N sequence Gly-Lys-(Lys)2-(Lys)4-(Arg)8, [M + H]+ of 2222.5) were synthesized by Fmoc-solid phase peptide synthesis, purified and characterized, as described previously (Parekh et al., Citation2006; Mutalik et al., Citation2013). Rink amide resin was swollen with dimethylformamide (DMF) and deprotected with piperidine (20% v/v in DMF). After washing with DMF, Fmoc-Gly-OH (previously activated with HBTU and DIEA) was coupled to the rink amide resin. The resulting product was treated with piperidine (20% v/v in DMF) and washed with DMF. Then next amino acid was coupled. Coupling of amino acids and deprotection was performed continuously until the desired peptide dendrimer was obtained. Ninhydrin test was performed to confirm the efficiency (≥95% coupling) of amino acid coupling. After the synthesis process was completed, the final Fmoc group was cleaved off-resin by treating it with piperidine (20% v/v in DMF). Then, the product was washed with DMF and dried product was stirred in a mixture of TFA, DCM, water and TIPS. After removing TFA, the residue was azeotroped with acetonitrile followed by trituration with diethyl ether. It was then dissolved in water and lyophilized. Synthesized dendrimers were purified by using a preparative RP-HPLC system (Waters). They were analyzed by RP-HPLC for single peak purity and ESI+-MS (2000 QTRAP Nano spray™, MDS Sciex, Ontario, Canada) for the molecular ion ([M + H]+) peak.
Solubility studies
Saturation solubility of 5-FU was determined in aqueous buffer solutions of differing pH (pH 4.5, 7.4 and 8). An excess amount of drug was added to different buffer solutions and the mixture was placed on rotary shaker for 24 h at room temperature. The concentration of 5-FU in these saturated solutions was determined spectrophotometrically at 261 nm after passing through a 0.45-µm membrane filter.
Partition coefficient determination
The partition coefficient values of 5-FU in the presence and absence of peptide dendrimers was determined. Briefly, into a separating funnel containing n-octanol solution (10 mL) was added an equal volume of saturated drug solution in water/pH7.4 phosphate buffer containing different peptide dendrimers. The system was kept at room temperature for 24 h with intermittent shaking. Finally, aqueous and organic layers were separated, clarified by centrifugation at 1000 rpm for 5 min, and assayed by RP-HPLC after passing through a 0.45-µm membrane.
Stability of 5-FU in skin extracts
The degradation of 5-FU was assessed in different skin extracts namely, epidermal, dermal and homogenized skin extracts. Freshly excised human skin was mounted in a diffusion cell, with stratum corneum facing the donor compartment and the dermis facing the receptor compartment, and both compartments were filled with donor and receptor solutions, respectively. After 8 h, the epidermal facing solution (from donor compartment) and dermal facing solution (from receptor compartment) were collected separately. Skin extract was prepared by homogenizing freshly excised skin (1 cm2) in distilled water (10 mL) for 10 min in an ice bath. The mixture was centrifuged at 5000 rpm for 15 min and supernatant subsequently collected. The drug solution (in water) was mixed with 5 mL of the epidermal, dermal and skin extracts separately. The samples were shaken in a water bath at 32 °C and at 50 rpm. At different time intervals up to 6 h, the concentration of drug in each extract was determined by RP-HPLC. Drug solution in water alone was used as control.
In vitro skin permeation studies
Human epidermis prepared from full thickness skin taken from the abdominal area was used as a membrane in permeation studies. The abdominal skin obtained following elective surgery was subjected to blunt dissection to remove extraneous tissue. The full thickness skin obtained was immersed in water at 60 °C for 1 min, after which the epidermal layer was gently peeled off using forceps. The epidermal membrane was placed flat on aluminum foil and stored at −20 °C. The collection of skin was approved by the University of Queensland Medical Research Ethics Committee.
The in vitro skin permeation studies were conducted using vertical-type diffusion cells having a surface area of 1 cm2 and a receptor compartment capacity of 3.5 mL. Excised human epidermis was mounted on the diffusion cell between the donor and the receptor compartments of each diffusion cell, with the epidermis facing the donor chamber. The donor solution (2.5 mL; pH 7.4) comprised of 50 mM HEPES and 75 mM NaCl, with varying concentrations of dendrimer and 5-FU in saturated suspension form placed in the donor compartment. A saturated drug solution was prepared in donor solution. Then a minute quantity of 5-FU was added to make it as a suspension to maintain high thermodynamic activity. The receptor compartment was filled with a solution containing 3.5 mL of 50 mM HEPES and 75 mM NaCl solution (pH 7.4). Before commencing permeation studies, each diffusion cell mounted with epidermis was allowed to equilibrate for 45 min using blank donor and receptor solutions. Uniform mixing of the solution in the receptor compartment was achieved using a magnetic flea. Electrical resistance of the membrane was determined before and after each set of experiments to ensure its integrity for the validity of any generated data. Samples from receptor compartment (500 µL) were withdrawn at regular time intervals of 0, 2, 4, 8, 10, 12, 24, 28, 32, 36 and 48 h and assayed for 5-FU using RP-HPLC. The samples collected from the receptor compartment were replaced with fresh receptor solutions of the identical volume, at each sampling. Cumulative amount of drug permeated versus time was plotted and flux was calculated by using this graph.
At the end of the experiment, the mounted skin piece was dismounted and cleaned first with running water and then with distilled water. The skin piece was sliced into small pieces and the drug retained in skin was extracted with distilled water. The mixture was centrifuged at 5000 rpm for 15 min, filtered (through a 0.45-µm membrane filter) and the amount of drug in the resulting solution was estimated using RP-HPLC. The amount of drug remaining in the donor solution at the end of each permeation study was also determined to provide a value for the loss of drug during permeation experiments (Mutalik & Udupa, Citation2002; Chandra & Sharma, Citation2008; Mutalik et al., Citation2009b).
Effect of pH on 5-FU permeation
To assess the effect of pH of donor solution on the transdermal permeation of 5-FU, the permeation study was conducted at different donor solution pHs (pH 4.5, 7.4 and 8.0). The procedure was carried out in the manner outlined above except where the pH of the donor solution differed (pH 4.5, 7.4 or 8).
Skin permeation studies in presence of peptide dendrimers
The permeation studies were conducted in a similar manner to that outlined in the section “In vitro skin permeation studies”; with the only difference being that dendrimer was present alongside 5-FU in the donor compartment. The studies in the presence of dendrimers were conducted either by (i) simultaneously application of drug and dendrimer or (ii) pretreating skin with dendrimers 2 h prior to application of drug solution (Mutalik et al., Citation2012).
In the simultaneous application studies, initially a saturated drug solution was prepared in plain donor solution and dendrimer was dissolved in this donor solution. Then a little quantity of 5-FU was added to make it as a suspension. This entire mixture containing drug and dendrimer was used as the donor solution. The donor solution (containing 5-FU) without dendrimer was served as control.
In pretreatment studies, the skin (≈1 cm2) was treated with 0.5 mL of 25 mg/mL dendrimer in HEPES solution (pH 7.4) for a predetermined time (2 h) after hydration. After complete removal of the dendrimer solution, the permeation studies were conducted in the same way as outlined earlier (Section “In vitro skin permeation studies”).
Differential scanning calorimetry
Differential scanning calorimetry (DSC) analysis of stratum corneum: stratum corneum was obtained from the full thickness skin by treating the skin overnight with 0.5% sodium bicarbonate solution containing 0.1% of trypsin (Renata et al., Citation2000). The stratum corneum layers were dried in a desiccator at room temperature for seven days. On the 8th day, a small piece of stratum corneum (1 cm2) was treated with 0.5 mL of either pH 7.4 buffer or R8 dendrimer solution (10 mg/mL) for 2 h. The solution was then wiped off and the stratum corneum pieces were dried in a vacuum desiccator for ≈4 h. Sliced pieces of stratum corneum were sealed in an aluminum pan and scanned using DSC-60 Differential scanning calorimeter (Shimadzu, Kyoto, Japan) up to 150 °C at a heating rate of 1 °C/min under a carpet of N2 (30 mL/min). Empty aluminum pan was used as a reference. The heat flow as a function of temperature was measured for the samples. Temperature calibration was made using indium as standard.
DSC analysis of drug–dendrimer complex: The drug-R8 dendrimer complex was prepared at the drug: dendrimer ratio of 1:0.5 by the co-precipitation method, as per previously reported protocols (Devarakonda et al., Citation2007; Yiyun et al., Citation2007). Pure 5-FU, pure R8 dendrimer and the drug-R8 dendrimer complex were subjected to DSC analysis by following the method explained above, except the maximum temperature for scanning and heating rate were 300 °C and 5 °C/min, respectively.
Zeta potential measurements
Zeta potential was determined using a NanoZS (Malvern Instrument Limited, UK) for the solutions of 5-FU, R8-dendrimer and the complex of 5-FU and R8-dendrimer in pH 7.4 phosphate buffer.
HPLC analysis of 5-FU
5-FU was analyzed using an integrated HPLC system (LC-2012CHT, Shimadzu, Japan) equipped with low pressure quaternary gradient pump along with dual wavelength UV detector, auto sampler and column oven. The chromatographic data was processed using LC solution 1.24 SP1 software. The column used was a RP C18 column (GraceVydac; particle size 5 µm; 250 × 4.6 mm), maintained at 25 °C. The mobile phase consisted of acetonitrile:phosphate buffer (10 mM, pH 3.5, adjusted with dilute o-phosphoric acid) in a ratio of 10:90 v/v and flow rate was 0.8 mL/min. The total run time for each sample was 20 min. The detector wavelength was set at 264 nm and the volume of injection was 25 µL. The method was validated with respect to its calibration curve (R2: >0.9990, over the concentration range of 10–1000 ng/mL), precision of the area (RSD values ranging between 0.05% and 2%) and accuracy (between 98% and 102% at three different concentrations). The retention time of 5-FU was found to be 7.7 min and the peak was devoid of any interfering peaks from skin. There was no interference on the peak of 5-FU from any skin constituents from epidermal, dermal or skin extracts.
Statistical analysis
One-way analysis of variance (ANOVA) followed by Tukey’s post hoc test was used to analyze the results (Graph Pad Prism 5.0 software) and a “p” value less than 0.05 was considered statistically significant.
Results and Discussions
Dendrimer synthesis and characterization
Peptide dendrimers designed with arginine as terminal amino acids and having a range of positive charges and molecular weights (4+, 8+ & 16+; 515.8, 1084.4 & 2222.5 Da, respectively (Section “Synthesis of peptide dendrimers”) were synthesized by solid phase peptide synthesis. The yield of all dendrimers after synthesis was at least 70% with each dendrimer being of >95% purity before being subjected to skin delivery experiments. All the peptide dendrimers were successfully purified by preparative RP-HPLC (semi-automated) and were collected within the first 10 min of the total run time. During characterization using ESI+-MS, all dendrimers clearly showed the desired molecular ion ([M + H]+) which was in accordance with the theoretically calculated molecular weights of each dendrimer, thus confirming the formation of each target peptide dendrimer. All the synthesized peptide dendrimers also exhibited single peak profiles in analytical RP-HPLC.
5-FU solubility studies
The aqueous solubility of 5-FU as well as that in different buffer solutions (pH 4.5, 7.4 and 8) was determined. 5-FU showed greater solubility at higher pH values, which can be attributed to a greater proportion of drug being ionized under alkaline conditions. The results of the 5FU solubility studies are given below:
Distilled water: 13.56 ± 0.46 mg/mL; pH 4.5: 12.40 ±0.51 mg/mL; pH 7.4: 16.76 ± 0.36 mg/mL; pH 8: 23.88 ±0.61 mg/mL. The solubility of 5-FU in pH 7.4 phosphate buffer increased in the presence of peptide dendrimers (22.46 ± 0.86, 23.98 ± 0.45 and 24.56 ± 0.44 mg/mL in presence of R4, R8 and R16 dendrimers, respectively). The results also showed that the solubility of 5-FU increased, albeit slightly, with increasing charge (molecular weight) of peptide dendrimer (i.e. R16 showed better solubility than R8 and R4 dendrimers). Our results are in accordance with those findings from earlier PAMAM dendrimers studies, which showed solubility of 5-FU improved in a generation-dependent manner, although the extent of increase in solubility with peptide dendrimers was less than PAMAM dendrimers (Venuganti & Perumal, Citation2008).
5-FU partition coefficient determination
The n-octanol/water and n-octanol/phosphate buffer (pH 7.4) partition coefficient values for 5-FU were determined. The partition coefficient values of 5-FU in n-octanol/water and n-octanol/phosphate buffer were found to be 0.14 (log P: −0.85) and 0.30 (log P: 0.52), respectively, which are in agreement with earlier reported values (Carla & Angela, Citation2003; El Maghraby et al., Citation2005). The n-octanol/phosphate buffer partition coefficient values of 5-FU were found to increase in the presence of peptide dendrimers. Here, the n-octanol/phosphate buffer partition coefficient values of 0.76 (log P: 0.12), 0.78 (log P: 0.10) and 0.79 (log P: 0.10) were observed for 5-FU in the presence of R4, R8 and R16 dendrimers, respectively. These increased partition coefficient values indicate that the peptide dendrimers enhance the solubility and partitioning of 5-FU into the highly lipidic stratum corneum layer (Venuganti & Perumal, Citation2008). The published range of ideal partition coefficient values of drugs considered good candidates for transdermal permeation is 0.1–10 000 (i.e. a log P from −1 to 4) (Chandrashekar & Shobha Rani, Citation2008), indicating that 5-FU has an appropriate partition coefficient value for transdermal delivery.
5-FU stability in skin extracts
The stability of 5-FU was studied in different extracts of skin such as the epidermis, dermis and whole-skin extracts up to 6 h. 5-FU displayed excellent stability in all the tested extracts at the end of 6 h with values of 98.90%, 98.46% and 98.06% calculated in epidermal, dermal and skin extracts, respectively. The stability of 5-FU in phosphate buffer (pH 7.4) alone was also tested (control) with the drug showing 99.26% stability at the end of 6 h. These findings indicate that 5FU does not undergo degradation to any appreciable extent during skin permeation studies, and is a good candidate for transdermal delivery.
5-FU in vitro skin permeation studies
The in vitro transdermal permeation of 5-FU across human skin was investigated under a range of experimental conditions (i.e. differing donor solution pH, differing concentrations of dendrimer in donor solution, simultaneous application of 5-FU and dendrimer in donor solution and finally, conducting a 5-FU permeation study after treating the skin (“pretreatment”) with dendrimeric solution).
The effect of varying pH levels (4.5, 7.4 and 8) of donor solution on the transdermal permeation of 5-FU is shown in and . As the pH of donor solution increased from 4.5 to 7.4, there was an increase in the flux and cumulative amount of drug permeation values. Highest flux and cumulative drug permeation values (16.48 ± 1.57 µg/cm2/h and 756.11 ± 33.12 µg, respectively) were observed at pH 7.4. However, further pH increases (to pH 8) were associated with a decrease in flux and cumulative amount of 5-FU permeation. These findings are in accordance with previously published results where the flux value also decreased under alkaline conditions, although the solubility of 5-FU improved (Brahma & Shyam, Citation2008). The amount of 5-FU depositing in skin also varied in agreement with flux values and again, the highest content of drug in skin was observed at pH 7.4 (96 ± 3.80 µg/cm2). Loss of drug (%) during skin permeation studies at all pH conditions was found to be negligible (<2%) and based on these collective outcomes the donor solution was adjusted to pH 7.4 in all subsequent studies.
Table 1. Effect of pH on the permeation of 5-FU across human skin.
The effect of differing peptide dendrimer concentrations (5, 10 and 20 mg/mL, each for R4, R8 and R16) on the skin permeation of 5-FU with simultaneous application (5-FU + dendrimer) are shown in and . The results indicated that each peptide dendrimer at all the tested concentrations increased the flux and cumulative amount of 5-FU permeation upon simultaneous application in the donor compartment. There was an increase in the flux, Q48 and drug retention in skin when the charge (and so MW) of dendrimer was increased from 4+ to 8+. However, further increases in the charge (to 16+) led to a decline in the flux, Q48 and skin retention of 5-FU. Overall, the arginine-terminated dendrimer (R8) with 8+ charge and intermediate MW (≈1000) showed good permeation enhancing properties for 5-FU. Similarly, we also found there to be an increase in the flux, Q48 and skin retention of 5-FU as the concentration of each dendrimer was increased from 5, 10 to 20 mg/mL. Among the tested dendrimers, R4 (at 10 and 20 mg/mL) and R8 (at 5, 10 and 20 mg/mL) showed significant (p < 0.05) increases in flux, Q48 and drug retention in skin values compared to control (without dendrimer). R8 dendrimer showed the greatest 5-FU permeation enhancing effect among all dendrimers, with 20 mg/mL showing highest skin permeation, Q48 and skin deposition for 5-FU, although there was negligible difference between the permeation parameters observed at 10 mg/mL for R8 (flux: 74.94 ± 6.63 µg/cm2/h; Q48: 3468.37 ± 149.3 µg; skin retention of drug: 328 ±9.43 µg/cm2) and 20 mg/mL (flux: 78.07 ± 7.52 µg/cm2/h; Q48: 3666.44 ± 162.70 µg; skin retention of drug: 378 ± 15.11 µg/cm2). In all the simultaneous application diffusion studies, the (%) loss of drug during permeation experiments was again negligible (<2%), indicating the greater stability of 5-FU during permeation across skin.
Figure 2. Influence of R4-dendrimer concentrations on the in vitro skin permeation profiles of 5-FU (simultaneous application).
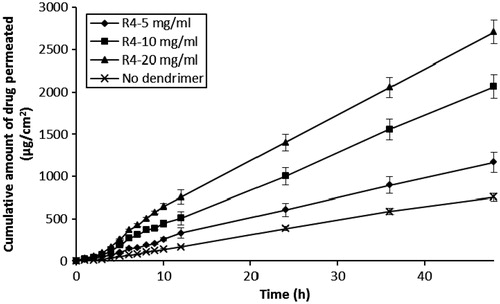
Figure 3. Influence of R8-dendrimer concentration on the in vitro skin permeation profiles of 5-FU (simultaneous application).
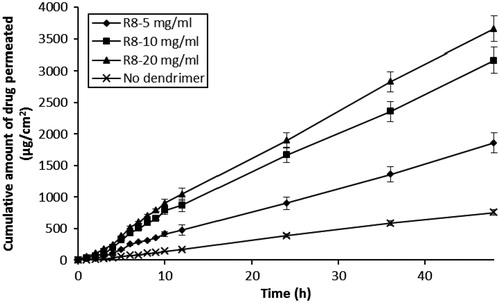
Figure 4. Influence of R16-dendrimer concentration on the in vitro skin permeation profiles of 5-FU (simultaneous application).
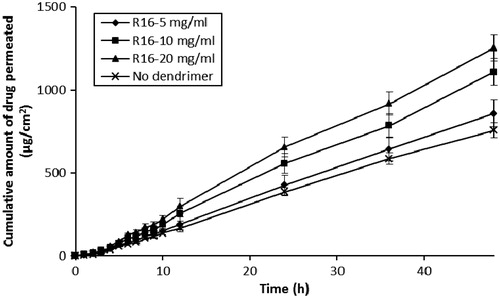
Table 2. Effect of differing dendrimer concentration on the permeation of 5-FU across human skin following simultaneous application.
Next, permeation studies were performed by pretreating skin with dendrimeric solution for two hours prior to application of 5-FU (0.5 mL dendrimeric solution at 25 mg/mL) to ascertain whether the interaction of dendrimers with skin was independent of its interaction/association with 5-FU. Interestingly, all three dendrimers (R4, R8 and R16) increased the skin permeation of 5-FU in pretreatment studies ( and ) c.f. control (application of 5-FU alone). Of the three dendrimers tested, R8 and R16 dendrimers showed significant (p < 0.05) increases in flux, Q48 and drug retention in skin values compared to control while R8 exhibited highest values for flux (63.33 ± 5.88 µg/cm2/h), Q48 (3035 ±140.50 µg) and skin content of drug (236 ± 9.97 µg/cm2). As in the earlier simultaneous application studies, the (%) loss of drug was negligible (<2%) in pretreatment studies once again indicating the greater stability of 5-FU in skin permeation studies ().
Table 3. Effect of pretreatment with dendrimers on the skin permeation of 5-FU.
A number of plausible mechanisms, acting alone or in unison can be attributed to peptide dendrimers and their ability to enhance the skin permeation of 5-FU. They may increase the oil/water partition coefficient of 5-FU (as shown above) through simultaneous interaction of 5-FU with the dendrimer core/charged head groups, facilitating migration into the highly lipidic stratum corneum layer. The dendrimer, with its surface cationic charge, may be responsible for disrupting the highly ordered, compact arrangement of viable cells within skin via interaction with lipids and/or protein structures, thus enhancing the transdermal permeation of 5-FU.
Our partition coefficient determinations performed with 5-FU (+/− dendrimer) certainly indicate that peptide dendrimers increase log P values of 5-FU, which result in improved lipophilicity, and hence stratum corneum deposition and migration properties.
Furthermore, there exists ample evidence in the literature of nonpeptidic dendrimers increasing skin permeation/deposition of drugs through complex formation, this compared to drug alone (Yiyun et al., Citation2007; Venuganti & Perumal, Citation2008; Borowska et al., Citation2012). In this study, this attractive property can be extended to peptide dendrimers, given that 5-FU-peptide dendrimer complexes were allowed to form in the donor solution (2–3 h) prior to conducting diffusion studies. To confirm whether complexes were indeed being formed between 5-FU and dendrimer molecules, we subjected the lyophilized donor solution (containing R8 dendrimer at 10 mg/mL and 5-FU) to analysis using differential scanning calorimetry (DSC, ). Prior to testing any complexes the endotherms of 5-FU and R8 (alone) were first determined, with peaks at 291.82 °C (melting point of 5-FU, ) and 226.76 °C [corresponding to the melting point of L-arginine (222 °C); ] recorded. In contrast, the thermogram of a mixture of 5-FU+R8 dendrimer from the donor solution () was notably different, with the peak of R8 dendrimer no longer detected and the intensity of the endothermic peak corresponding to 5-FU was reduced. In addition to this, there was a decrease in melting point value of 5-FU (285.33 °C) in comparison with that of native drug. These observations indicate that a firm association between 5-FU and dendrimer in the donor solution existed, and this could well be responsible for their greater skin deposition and permeation properties.
Figure 6. DSC of (A) 5-FU, (B) R8 dendrimer, (C) R8 dendrimer+5-FU from donor solution and (D) R8 dendrimer-5-FU complex (1:0.5 molar ratio).
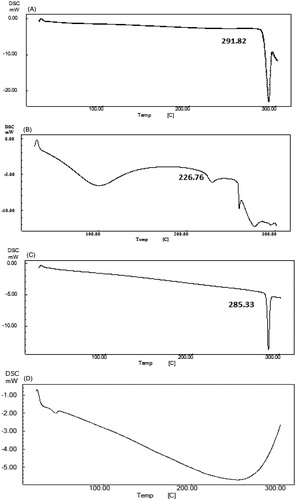
To ascertain whether peptide dendrimers increase permeation of 5-FU by disrupting the highly ordered lipid and/or protein structures of stratum corneum, DSC analysis of plain (untreated) stratum corneum as well as R8-dendrimer-treated stratum corneum was carried out (). Untreated stratum corneum showed three major endothermic transition peaks at 59.3 °C, 80.9 °C and 104.8 °C which can be assigned essentially to lamellar lipids, lipid–protein (keratin) complex and proteins, respectively, in the stratum corneum () (Yamane et al., Citation1995; Diksha & Bozena, Citation2010). Additionally, another endothermic peak was observed at 39.84 °C, which we postulate arise from sebaceous lipids that are loosely bound to the stratum corneum surface (Yamane et al., Citation1995). In contrast, a thermogram of stratum corneum treated with R8 dendrimer was absent in all the aforementioned peaks [lamellar lipids, lipid–protein (keratin) complexes and proteins, as well as sebaceous lipids], indicating a highly probable interaction of peptide dendrimers with these components of stratum corneum, and so enhancements in deposition and permeation of 5-FU () in/across skin through a “softening” of skin-based lipids (Diksha & Bozena, Citation2010). Although not studied here, it also has been reported with PAMAM dendrimers that pretreatment results in increased transepidermal water loss and decreased skin resistance due to the interaction of dendrimers with skin lipids (Venuganti & Perumal, Citation2008). And similar mechanisms might also be at play here, cooperatively enhancing the transdermal permeation of 5-FU.
Figure 7. DSC thermogram of (A) untreated stratum corneum and (B) R8 dendrimer-treated stratum corneum.
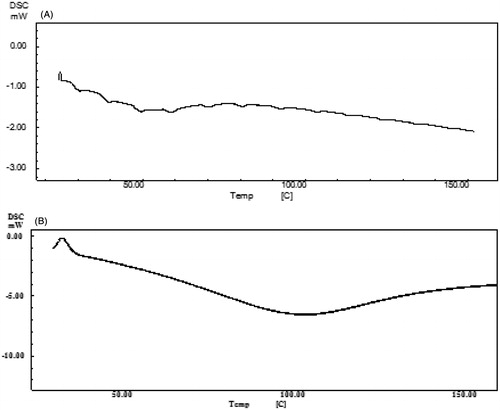
To elucidate further, 5-FU-R8 dendrimer complexes were prepared at a molar ratio of 1:0.5 following a coprecipitation method reported previously (Devarakonda et al., Citation2007; Yiyun et al., Citation2007) and skin permeation properties of these complexes was determined. If a complex formed in the presence of excess drug relative to dendrimer, then the melting point, intensity of endothermic peak and charge would also be expected to change in comparison to 5-FU alone. The prepared drug–dendrimer complexes were characterized using DSC and zeta potential measurements were also taken. The DSC thermogram of this prepared complex was entirely different than that of individual R8 dendrimer and 5-FU and the endothermic peaks of both (dendrimer and 5-FU) molecules disappeared. To measure the change in the charge upon complexation, the zeta potential was measured next and found to be −4.52 mV for 5-FU solution in pH 7.4 phosphate buffer. The zeta potential of R8 dendrimer solution however was +6.16 mV. On the other hand, the zeta potential of R8 dendrimer–drug complexes (1:0.5) was +2.36 mV, clearly suggesting that complexes were formed, due to a fall in the net cationic charge, most likely being offset (partial neutralization) by 5-FU.
Next, skin permeation studies of 5-FU-R8 dendrimer complex (1:0.5 molar ratios) was performed and values of flux, Q48 and the retention of drug within skin were calculated and found to be considerably greater than when 5-FU alone was trialled. Drug-R8 dendrimer complexes showed flux, Q48 and drug content within skin values of 44.16 ± 2.68 µg/cm2/h, 1846.98 ± 91.98 µg and 186 ± 6.86 µg/cm2, respectively which were significantly (p < 0.05) different to those observed in permeation studies comprising 5-FU alone (i.e. flux: 20.48 ± 1.57 µg/cm2/h; Q48: 780.06 ± 29.44 µg; drug content within skin: 85.1 ± 2.9 µg/cm2). However, the permeation values observed with 5-FU-dendrimer complexes (1:0.5) were less encouraging when compared with simultaneous application of drug and R8 dendrimer (Flux: 78.07 ± 7.52 µg/cm2/h; Q48: 3666.44 ± 162.7 µg; drug content within skin: 378 ± 15.11 µg/cm2) and also those observed pretreatment of skin with R8 dendrimer (Flux: 63.33 ± 5.88 µg/cm2/h; Q48: 3035 ± 140.50 µg; drug content within skin: 236 ± 9.97 µg/cm2). These observations collectively suggest that “free” peptide dendrimer (uncomplexed) in any topical formulation is required and indeed essential for superior skin deposition and permeation of 5-FU.
In surmising, we can confirm that peptide dendrimers are effective transdermal permeation enhancers acting through a number of mechanisms: (i) increased oil/water partition coefficient of drug by peptide dendrimers; (ii) formation of drug–peptide dendrimer complex which shows better skin permeation compared to plain drug; and (iii) disruption of the well-organized structure of skin by peptide dendrimers via interacting with lipid and/or protein structures of skin. However, the results of this study and underlying mechanisms by which peptide dendrimer act as transdermal permeation enhancers deduced in this study can be confirmed after conducting similar type of studies with other drug candidates.
Conclusion
The panel of synthesized peptide dendrimers (R4, R8 and R16) considerably increased the permeation of 5-FU across human epidermis, with R8 dendrimer showing maximal skin deposition and permeation enhancing effects for 5-FU, among those trialled. The broader application of peptide dendrimers in skin delivery of other therapeutically relevant therapeutics such as anti-nociceptive and anti-inflammatory agents now warrants attention, and the studies described above pave the way for such studies in the future.
Declaration of interest
The authors report no conflicts of interest. The authors alone are responsible for the content and writing of this article.
Authors express sincere thanks to Department of Biotechnology, Government of India, New Delhi, for the financial assistance.
Acknowledgements
The authors are also thankful to the School of Pharmacy (PACE), The University of Queensland, Brisbane, Australia, and Manipal College of Pharmaceutical Sciences, Manipal University, Manipal, India, for providing necessary facilities.
References
- Bai S, Ahsan F. (2009). Synthesis and evaluation of pegylated dendrimeric nanocarrier for pulmonary delivery of low molecular weight heparin. Pharm Res 3:539–48
- Benson HAE. (2005). Transdermal drug delivery: penetration enhancement techniques. Curr Drug Del 2:23–33
- Brahma NS, Shyam BJ. (2008). Iontophoretic delivery of 5-fluorouracil through excised human stratum corneum. Drug Discov Ther 2:128–35
- Borowska K, Wołowiec S, Rubaj A, et al. (2012). Effect of polyamidoamine dendrimer G3 and G4 on skin permeation of 8-methoxypsoralene – in vivo study. Int J Pharm 426:280–3
- Clara G, Angel C, Inmaculada G, et al. (2008). Laser treatments on skin enhancing and controlling transdermal delivery of 5-fluorouracil. Lasers Sur Med 40:6–12
- Carla AMC, Angela MM. (2003). Encapsulation of 5-fluorouracil in liposomes for topical administration. Acta Sci Technol 25:53–61
- Chauhan AS, Sridevi S, Chalasani KB, et al. (2003). Dendrimer mediated transdermal delivery: enhanced bioavailability of indomethacin. J Control Release 3:335–43
- Chandra A, Sharma PK. (2008). Proniosome based drug delivery system of piroxicam. Afr J Pharm Pharmacol 2:184–90
- Chandrashekar NS, Shobha Rani RH. (2008). Physicochemical and pharmacokinetic parameters in drug selection and loading for transdermal drug delivery. Indian J Pharm Sci 70:94–6
- Chien YW. (1987). Transdermal therapeutic systems. In: Robinson JR, Lee VHL, eds. Controlled drug delivery. New York: Marcel Dekker Inc, 523–49
- Cloninger MJ. (2002). Biological applications of dendrimers. Curr Opin Chem Biol 6:742–8
- Dhamecha DL, Rathi AA, Saifee M, et al. (2009). Drug vehicle based approaches of penetration enhancement. Int J Pharm Pharm Sci 1:24–36
- Diasio RB, Harris BE. (1989). Clinical pharmacology of 5-fluorouracil. Clin Pharmacokinet 16:215–37
- Diksha K, Bozena M. (2010). Percutaneous penetration modifiers and formulation effects: thermal and spectral analyses. AAPS PharmSciTech 11:1068–83
- Devarakonda B, Otto DP, Judefeind A, et al. (2007). Effect of pH on the solubility and release of furosemide from polyamidoamine (PAMAM) dendrimer complexes. Int J Pharm 345:142–53
- El Maghraby GMM, Williams AC, Barry BW. (2005). Drug interaction and location in liposomes: correlation with polar surface areas. Int J Pharm 292:179–85
- Elka T, Lizette A. (1985). Effect of propylene glycol, azone and n-decylmethyl sulphoxide on skin permeation kinetics of 5-fluorouracil. Int J Pharm 27:89–98
- El-Sayed M, Ginski M, Rhodes C, Ghandehari H. (2002). Transepithelial transport of poly(amidoamine) dendrimers across Caco-2 cell monolayers. J Control Release 3:355–65
- Filipowicz A, Wolowiec S. (2011). Solubility and in vitro transdermal diffusion of riboflavin assisted by PAMAM dendrimers. Int J Pharm 1:152–6
- Goette DK. (1981). Topical chemotherapy with 5-fluorouracil – a review. J Am Acad Dermatol 4:633–49
- Howard DB, Kenneth BS. (1996). Transdermal delivery of 5-fluorouracil (5-FU) by 1-alkylcarbonyl-5-FU prodrugs. Int J Pharm 129:203–10
- Fang J, Chi-Feng H, Yi-Ping F, Te-Fu C. (2004). Transdermal iontophoresis of 5-fluorouracil combined with electroporation and laser treatment. Int J Pharm 270:241–9
- Kannan RM, Perumal OP, Kannan S. (2005). Dendrimers and hyperbranched polymers in drug delivery. In: Labhateswar V, Pelecky L, eds. The biomedical applications of nanotechnology. New Jersey: Wiley Inc, 105–30
- Kumar JA, Pullakandam N, Prabhu SL, Gopal V. (2010). Transdermal drug delivery system: an overview. Int J Pharm Sci Rev Res 3:49–54
- Madishetti SK, Palem CR, Gannu R, et al. (2010). Development of domperidone bilayered matrix type transdermal patches: physicochemical, in vitro and ex vivo characterization. DARU 18:221–9
- Marjukka TT, Servet B, Nadir B, et al. (1993). Enhanced delivery of 5-fluorouracil through shed snake skin by two new transdermal penetration enhancers. Int J Pharm 92:89–95
- Meidan VM, Walmsley AD, Docker MF, Irwin WJ. (1999). Ultrasound-enhanced diffusion into coupling gel during phonophoresis of 5-fluorouracil. Int J Pharm 185:205–13
- Menjoge AR, Navath RS, Asad A, et al. (2010). Transport and biodistribution of dendrimers across human fetal membrane: implications for intravaginal administration of dendrimer-drug conjugates. Biomaterials 18:5007–21
- Mutalik S, Udupa N. (2002). Transdermal delivery of glibenclamide and glipizide: in vitro permeation studies through mouse skin. Pharmazie 57:838–41
- Mutalik S, Hewavitharana AK, Shaw PN, et al. (2009a). Development and validation of a reversed-phase high-performance liquid chromatographic method for quantification of peptide dendrimers in human skin permeation experiments. J Chromatogr B 29:3556–62
- Mutalik S, Parekh HS, Davies NM, Udupa N. (2009b). A combined approach of chemical enhancers and sonophoresis for the transdermal delivery of tizanidine hydrochloride. Drug Deliv 2:82–91
- Mutalik S, Parekh HS, Anissimov YG, et al. (2013). Iontophoresis-mediated transdermal permeation of peptide dendrimers across human epidermis. Skin Pharmacol Physiol 26:127–38
- Mutalik S, Nayak UY, Kalra R, et al. (2012). Sonophoresis-mediated permeation and retention of peptide dendrimers across human epidermis. Skin Res Technol 1:101–17
- Na M, Yiyun C, Tongwen X, et al. (2006). Dendrimers as potential drug carriers. Part II- Prolonged delivery of ketoprofen by in vitro and in vivo studies. Eur J Med Chem 41:670–4
- Niederhafner P, Sebestik J, Jezek J. (2005). Peptide dendrimers. J Pept Sci 11:757–88
- Nikhil K, Dilip D, Jeetendra N, Kushal G. (2008). Effect of permeation enhancers on the release and permeation kinetics of meloxicam gel formulations through rat skin. Asian J Pharm Sci 3:193–9
- Parekh HS, Marano RJ, Rakoczy EP, et al. (2006). Synthesis of a library of polycationic lipid core dendrimers and their evaluation in the delivery of an oligonucleotide with hVEGF inhibition. Bioorg Med Chem 14:4775–80
- Parekh HS. (2007). The advance of dendrimers: a versatile targeting platform for gene/drug delivery. Cur Pharm Des 27:2837–50
- Pathan IB, Setty CM. (2009). Chemical penetration enhancers for transdermal drug delivery systems. Trop J Pharm Res 8:173–9
- Raja MH, Ping Q, Gao Z. (1998). Penetration enhancing effect of tetrahydrogeraniol on the percutaneous absorption of 5-fluorouracil from gels in excised rat skin. J Control Release 55:297–302
- Renata FVL, Collet JH, Bentley LB. (2000). Influence of cyclodextrin complexation on the in vitro permeation and skin metabolism of dexamethasone. Int J Pharm 2000:127–32
- Ritesh K, Anil P. (2007). Modified transdermal technologies: breaking the barriers of drug permeation via the skin. Trop J Pharm Res 6:633–44
- Sharma N, Agarwal G, Rana AC, et al. (2011). A review: transdermal drug delivery system: a tool for novel drug delivery system. Int J Drug Dev Res 3:70–84
- Tiwary AK, Sapra B, Jain S. (2007). Innovations in transdermal drug delivery: formulations and techniques. Recent Pat Drug Deliv Formul 1:23–36
- Tsuji T, Sugai T. (1975). Topically administered fluorouracil in psoriasis. Arch Dermatol 105:208–12
- Vandamme TF, Brobeck L. (2005). Poly(amidoamine) dendrimers as ophthalmic vehicles for ocular delivery of pilocarpine nitrate and tropicamide. J Control Release 1:23–38
- Venuganti VVK, Perumal OP. (2008). Effect of poly(amidoamine) (PAMAM) dendrimer on the skin permeation of 5-fluorouracil. Int J Pharm 361:230–8
- Wang Z, Itoh Y, Hosaka Y, et al. (2003). Novel transdermal drug delivery system with polyhydroxyalkanoate and starbust polyamidoamine dendrimer. J Biosci Bioeng 5:541–3
- Wang R, Yaw-Bin H, Pao-Chu W, et al. (2007). The effects of iontophoresis and electroporation on transdermal delivery of indomethacin evaluated in vitro and in vivo. J Food Drug Anal 15:126–32
- Yamane MA, Williams AC, Barry BW. (1995). Effects of terpenes and oleic acid as skin penetration enhancers towards 5-fluorouracil as assessed with time; permeation, partitioning and differential scanning calorimetry. Int J Pharm 116:237–51
- Yiyun C, Na M, Tongwen X, et al. (2007). Transdermal delivery of nonsteroidal anti-inflammatory drugs mediated by polyamidoamine (PAMAM) dendrimers. J Pharm Sci 96:595–602