Abstract
The validity of a mathematical rationale for preparation of a fast-dissolving buccal mucoadhesive was tested. A buccal mucoadhesive biopolymeric formulation has been developed having pullulan as the main component. The formulation was duly evaluated physicochemically, via assays for intrinsic viscosity (resulting in 71.61 cm3 g−1), differential scanning calorimetry analysis (resulting in a Tg = 63 °C), thermogravimetric analysis (244–341 °C), moisture content determinations (14%, w/w), dissolution timeframe (41.6 s), mucoadhesion force (40 kg/cm2), scanning electron microscopy analyses (critical ray under 1.0 μm), mechanic strength (tensile strength = 58 N/mm2, deformation = 4.4%). The mucoadhesive formulation exhibited important characteristics for a drug carrier, that is, a 6 cm2 area, a fast dissolution timeframe, an adequate mucoadhesivity, resistance to both oxygen and water vapor penetration, increased viscosity in solution (ranging from 33.2 cm3/g to 71.61 cm3/g), easy molding, suitable water solubility and transparency.
Introduction
Over the last two decades, alternative pharmacological presentations have been developed for direct application of medication in the oral cavity. The driving force for the use of adhesive biopolymers has been based on the pioneering work of Nagai et al. (Citation1981), in the late 1970s and beginning of the 1980s, after demonstrating that the oral aphthae resulting from cancer ulcers could be treated with an anti-inflammatory steroid locally applied with the aid of bioadhesive polymers (Lee et al., Citation2000). The development of mucoadhesive buccal films has increased dramatically over the last decade, much due to being a promising drug delivery alternative to various therapeutic drug classes (Jyoti et al., Citation2011; Morales & McConville, Citation2011). Biopolymeric films releasing drug compounds directly toward the buccal mucosa further exhibit the additional advantage of avoiding the effect of hepatic first pass, by directing the drug absorption through the venous blood system that drains from the cheek (Dixit & Puthli, Citation2009; Kharenko et al., Citation2009). A biopolymeric film or strip can be defined as a pharmaceutical dosage form that employs a water-dissolving polymer (generally a hydrocolloid, which may be a bioadhesive polymer) which allows the dosage form to (sequentially) quickly hydrate, adhere and dissolve, when placed in any part of the oral cavity (i.e. buccal, palatal, gingival, lingual, sublingual or cheek mucosa) (Gupta et al., Citation2011). The term “bioadhesion” was first stated as the bonding of synthetic or natural macromolecules either to the mucus or to the epithelial surface. Bioadhesion is generally referred to as when interaction occurs between biopolymer and an epithelial surface; furthermore, the term “mucoadhesion” is used when interaction occurs between biopolymer and the mucus layer covering a tissue. Mucoadhesion is a complex phenomenon involving wetting, adsorption and interpenetration of biopolymer chains (Carvalho et al., Citation2010; Deshmukh et al., Citation2011). The substances usually employed for the preparation of bioadhesive polymers are natural or synthetic hydrophilic macromolecules that include numerous functional groups such as carboxyls, hydroxyls, amides, amines, among others, capable of establishing hydrogen bonds and promoting adhesion to the mucosa (Villanova et al., Citation2010). For this purpose, cellulose derivatives (carboxymethyl cellulose, hydroxypropylmethyl cellulose), polymers based on poly(meth)acrylic acid (carbopol, polycarbophil, polyacrylic acid, polyacrylates, copolymer of acrylic acid), natural and modified natural (agarose, chitosan, gelatin, hyaluronic acid, carrageenan, pectin, sodium alginate), among others, are usually used (Leathers, Citation2003; Seo et al., Citation2012). The polymers most employed in the development of buccal mucoadhesives, as observed in a patent survey, are amide gums, carboxymethyl cellulose, carrageenan, locust bean gum, pullulan, xanthan and konjac, as well as plasticizers such as glycerol, maltitol, olive and canola oils (among other oils), propylene glycol, sorbitol and xylitol. Among these raw materials, pullulan has been extensively used as a drug delivery carrier due to its biocompatibility and biodegradability (Kumar et al., Citation2012; Xiao et al., Citation2012). Pullulan and its derivatives have numerous demonstrated uses in foods, pharmaceuticals, cosmetics, biomedical and pharmaceutical industries. Pullulan, also known as α-1,4- α-1,6-glucan, is a linear polysaccharide polymer consisting of maltotriose units interconnected to each other by α-1,6-glycosidic bonds (see ). Three glucose units in maltotriose are connected by an α-1,4 glycosidic bond, whereas consecutive maltotriose units are connected to each other by an α-1,6 glycosidic bond. This biopolymer is commercially obtained from a fermentation process by the mold Aureobasidium pullulans, grown on a carbohydrate substrate (i.e. starch). Following fermentation, the cells are harvested and duly disrupted using either enzymes or physical forces. Pullulan is then extracted using a simple water extraction process (Mishra et al., Citation2011; Kumar et al., Citation2012).
Figure 1. Structural formula of pullulan gum, a linear polysaccharide biopolymer consisting of maltotriose units interconnected to each other by α-1,6-glycosidic bonds.
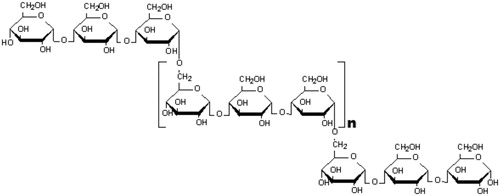
Considering the increasing need for the development and characterization of buccal mucoadhesive formulations for drug delivery, the research effort entertained herein aimed at developing a buccal mucoadhesive using pullulan as major ingredient, which exhibits mucoadhesivity, fast dissolution when in contact with salivary mucins, a low penetration of both oxygen and water, and possessing a sufficient free volume so as to be employed as a buccal drug carrier. To attain this goal, a reverse design of the formulation was pursued, departing from a mathematical model that allows prediction of the total amount of solids necessary to attain the formulation.
Material and methods
Materials and reagents
Pullulan gum (PI-20), carrageenan gum (INS407), xanthan gum (INS415) and locust bean gum (Locust Bean INS410) were acquired from Genkor Ingredientes Ltda (Itupeva, Brazil). Sorbitol (92% minimum purity) was from Corn Products (Valinhos, Brazil). All the other reagents were of PA grade or better, and the water used was (ultra)purified in a Milli-Q Elga Purelab system (Molsheim, France) to a final conductivity of ∼18.2 MΩ•cm−1. Polyethylene plastic film (50 μm thickness) was from GDM Indústria e Comércio de Plásticos (Murialdo – Orleans, Brazil).
Analytical equipment
Ultrasonic thermostatted bath (40 kHz) was from Unique (model UltraCleaner, Indaiatuba, Brazil). Thermostatted water bath was from Cientec (model CT-269, Piracicaba, Brazil). Magnetic stirring plates were from Instrulab (model DLH Agitator, Porto Alegre, Brazil). Forced air circulation drying chamber was from Marconi (model MA035, Piracicaba, Brazil). The digital micrometer used was from Mitutoyo Sul Americana Ltda (model MDC-25, São Paulo SP, Brazil). The Infrared Moisture Analyzer was from Gehaka (model IV 2000, São Paulo SP, Brazil). The thermogravimetric analyses were performed in a thermogravimeter from TA-Instruments (model TGA 20-50, New Castle, USA). The Differential Scanning Calorimeter (DSC) was from TA Instruments (model MDSC2910, New Castle, USA). Scanning Electron Microscopy analyses were performed in a scanning electron microscope from JEOL (model JSM-63660 CV, Tokyo, Japan). All mechanical properties were determined using an Instron Universal Testing Machine from Tensile (Barueri, Brazil).
Experimental procedures
Glass transition temperature model
The most important change, characteristic of the amorphous state that involves a transition from a glassy solid state to a gummy or rubbery state or from a rubbery state to a glassy one, occurs at the so-called glass transition temperature (Tg) (Levine & Slade, Citation1992). The significance of the determination of Tg for the mucoadhesive polymer under development is intimately related to the dissolution of the material at the temperature prevailing in the buccal cavity (from 35 °C to 37 °C), because this facilitates the dissolution of the amorphous phase of the polymer (see ). The Tg value was calculated according to the specifications by ASTM (Citation1999). The glass transition temperature (see ) for the mucoadhesive film was 63.08 °C (between 61.47 °C and 65.23 °C), with an endothermic peak at 118.18 °C (with absorption of 356.7 J/g). The glass transition temperature obtained for the mucoadhesive film (i.e. 63.08 °C) was higher than the temperature prevailing at the buccal cavity which, from the perspective of the product logistics (from manufacturing to consumption), is of significance to the conservation of the product regarding the temperature fluctuations suffered during shelf life. According to Fennema (Citation2000) and Ordóñez (Citation2005), Tg is influenced by the water activity in the product, meaning that an increase in the amount of water in the product by absorption implies an increase in the product vapor pressure and, consequently, reduces Tg. The absorption of water vapor is more pronounced in the amorphous phase than in the crystalline phase. The surface area and the affinity for water vapor are generally higher in amorphous materials than in crystalline substances (Burnett et al., Citation2009). The effect of water activity upon the glass transition temperature can be predicted by a Tg = ƒ{aw} relationship (see ) and, although such relationship is sigmoidal in nature (Roos, Citation1995), a linear regression approximation is usually used. The linearity applies to aw values between 0.1 and 0.8 (Roos, Citation1995), and thus water acts as a plasticizer of amorphous materials with its content defining the location of Tg (Roos, Citation1995). By adapting the data of Fennema (Citation2000) (see ) for postulation of a mathematical formula relating aw with Tg, the following relationship was obtained (Equation (1)):
Figure 2. Glass transition temperature (Tg, °C) as a function of water activity (aw), in amorphous materials (Adapted from Fennema (Citation2000)).
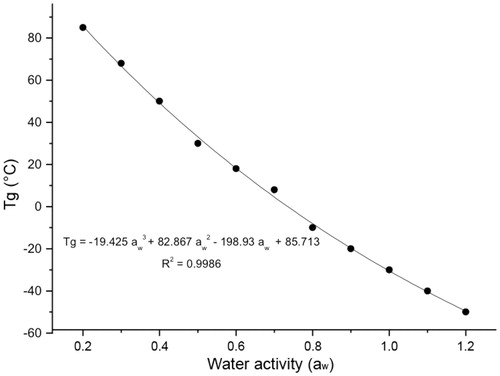
Figure 3. DSC thermogram of the mucoadhesive film, showing two endothermic peaks, one at 63.08 °C (Tg, glass transition temperature) and another at 118.18 °C (Tm, crystalline melting temperature, with energy absorption of 356.7 J/g).
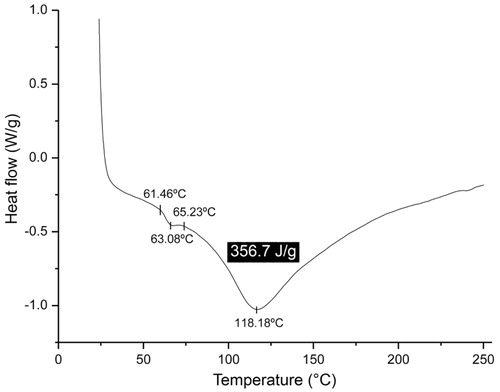
When applying Equation (1) to the Tg value of the mucoadhesive film being developed, that is Tg = 63.08 °C, a value of 0.11 was obtained for the water activity of the film. Therefore, according to Equation (1), to be able to reduce the Tg value from 63.08 °C down to 36 °C (the average temperature of the buccal cavity), so as to transform the mucoadhesive film from a “solid” state to a gummy state, it is necessary for the water activity to increase from 0.11 up to 0.27 (see ). Because water activity can be represented in terms of relative humidity, in order for the mucoadhesive film to achieve a gummy state, its relative humidity must be increased from 11% up to 27%. Hence, for an average film weight of 0.040 g, an extra 0.0052 g of water must be added [0.0108 g (water content needed in the film so as to achieve 27% relative humidity) – 0.0056 g (final water content of the film after drying, resulting in 14% relative humidity)], which is easily provided by the saliva in the buccal mucosa.
Crystalline melting temperature illations
At the crystalline melting temperature (Tm), melting of the crystals starts with a concomitant linear thermal expansion (Canevarolo, Citation2002). The melting of each small crystal produces an instantaneous and localized increase in the volume. The crystalline melting temperature of the mucoadhesive film, as can be seen in , is of 118.18 °C, which indicates a low content in crystals in the film formulation, thus favoring dissolution of the mucoadhesive film in the buccal cavity.
Preparation of the fast-dissolving mucoadhesive film
According to the mathematical rationale undertaken (see Results and Discussion section), 70 mL of ultrapure water was added to 25 g of pullulan and the resulting dispersion was left standing for 1 h. After this time period, the dispersion was placed in an ultrasonic bath (set at 40 kHz), heated up to 50 °C and kept at this temperature for 5 min to facilitate dissolution and avoid air bubble formation. Subsequently, 0.8 g sorbitol was added to the solution under constant magnetic stirring (150 rpm). The solid plasticizers were mixed apart from the liquid polymeric (pullulan) solution, following the proportion of 1.0 g k-carrageenan gum/0.15 g xanthan gum/0.15 g locust bean gum. At this stage, when preparing drug-loaded mucoadhesive films, the pharmaceutical compound was also dully added. The polymeric solution was then further heated at 80 °C in a thermostatted water bath, and the solid plasticizer mixture was carefully and slowly added under constant magnetic stirring for 5 min (150 rpm). This period of time was determined visually, considering the need for thickening and loss of suspension opacity. Afterwards, the resulting polymeric solution was poured into a polyethylene plastic film (50 μm thickness) placed on a cold surface (marble stone), and duly spread, after which the plastic film was bent to cover the polymeric film. The desired thickness of the polymeric film was achieved by compression with a metallic palette knife. The polymeric film was then allowed to cool and further dried in a forced air circulation drying chamber set at 40 °C, for 2 h, time after which its humidity was reduced to 14%. Following this cooling-drying timeframe, the films were cut into small strips of 2 cm (width) × 3 cm (length). The film thickness was obtained via measurements made with a digital micrometer in 10 film strips. The thickness defined for each strip sample was the result of 10 random measurements made in different parts of the same film strip, with calculation of the standard deviation associated therewith.
Moisture content determinations
The moisture content of the mucoadhesive films produced was determined by infrared analysis, using a linear temperature programming set at 0.1 °C/min up to 120 °C. All moisture content determinations were performed using 10 random film strip samples.
Thermogravimetric analyses (TGA)
Thermogravimetric analyses performed to the mucoadhesive film produced were carried out using 11.643 mg of film sample and a linear temperature programming up to 600 °C at a heating rate of 10 °C/min. The temperature for the onset of mass loss, considering the degradation starting point, was determined considering the maximum intensity of the first derivative of the TGA curve.
Determination of glass transition temperature (Tg)
Determination of the glass transition temperature (Tg) was accomplished using 7.1300 mg of film strip sample in a differential scanning calorimeter. A linear heating cycle from 10 °C up to 250 °C was imposed to the samples, at a heating rate of 10 °C/min, under inert (nitrogen) atmosphere.
Scanning electron microscopy (SEM) analyses
The surface of the mucoadhesive films was duly observed in a scanning electron microscope (from JEOL, model JSM-63660 CV, Tokyo, Japan). Film samples were sputter coated with colloidal gold under vacuum, and placed in the microscope chamber. Microphotographs were gathered using electron beams with acceleration speeds of 10–20 keV. The samples were randomly scanned and photomicrographed at magnifications of × 10 000 up to × 20 000.
Evaluation of the mechanical properties of the mucoadhesive film
Determination of both stress and strain of the polymeric mucoadhesive film was carried out using 10 film strip samples, following the Standard D882-91 methodology described by the American Society for Testing and Material Standard (ASTM) (Citation1999), in an Instron Universal Testing Machine. Ten samples of each formulation were cut, in longitudinal direction, with 50 mm in length and 20 mm in width and fit to the tensile grips. The crosshead speed was set at 0.8 mm/s and the initial distance between the grips was 30 mm. The tensile strength (MPa) and elongation at break (%) were determined. For the stress relaxation test, the samples were cut with 100 mm in length and 20 mm in width and fit to the tensile grips, with initial distance between the grips of 80 mm. The crosshead speed was set at 0.8 mm/s and the samples were tensioned (stretching) until reach 1.0% of deformation (based in the results of elongation at break). The stress developed by the film under tension was recorded at 0.04 s intervals, for 60 s.
Mucoadhesive film dissolution studies
To assess, in vitro, the film dissolution characteristics, the method of Chen et al. (Citation2006) was utilized. Hence, the time needed to achieve total dissolution of the films was determined in vitro. The tests consisted of adding small film strip pieces, each 10 s, to 25 mL of ultrapure water held at 37 °C in a 50-mL beaker, under magnetic stirring with vortex formation, until all material was totally dissolved. The lead time was dully recorded.
Viscosity determinations
Determination of the intrinsic viscosity of the pullulan aqueous solution in a molar weight range between 48 × 103 and 2.18 × 106, at 25 °C, was carried out by using Equation (3).
Results and discussion
Mathematical rationale for producing a mucoadhesive film
Pullulan was the gum elected to be the lead polymer in formulating a fast-dissolving mucoadhesive film due to several intrinsic properties, that is (i) being of fast dissolution, (ii) exhibit mucoadhesivity, (iii) being edible, (iv) being resistant to both oil migration and gas penetration (mainly to oxygen), (v) presents increased viscosity in solution, (vi) it is easily moldable, (vii) being water soluble and (viii) being transparent (Leathers, Citation2003). In addition, pullulan is nontoxic, nonimmunogenic, nonmutagenic and noncarcinogenic (Scott et al., Citation2001; Leathers, Citation2003; Mishra et al., Citation2011). Pullulan has characteristics of mucoadhesiveness because this polymer possesses carboxylic and hydroxyl groups in its molecule, as can be observed through the values encountered (40 kg.cm−2) (Cheng et al., Citation2011). Formulations produced solely with pullulan [i.e. with more than 90% (w/w) in the final film] as solute in the mucoadhesive film present a rigid and breakable texture, with the rigidity of the materials occurring as a function of the intramolecular forces. Therefore, a reduction in such forces can be achieved by adding other types of solutes, so as to allow a relaxation of the intramolecular forces within the polymeric folded structure with concomitant formation of volumetric spacing, hence allowing to accommodate the drug. Another important characteristic to be attained is the proper dissolution of the mucoadhesive film at a temperature close to the buccal temperature (between 35 °C and 37 °C). Therefore, the best procedure found was to attain the glass transition temperature (Tg), because at this point the product dissolves passing from a solid state to a gummy (viscous) state. For the film to dissolve in the buccal cavity, at its local temperature, it needs to attain 97% (w/w) solids in the final product formulation, according to the relationship between the glass transition temperature (Tg) and the (aqueous) solute (X) content (%, w/w) reported by Fennema (Citation2000) (see Equation (2)).
For the other components to be added in the formulation, k-carrageenan [in the proportion of 1.5% (w/w) relative to the added water] can be used due to its emulsifying, gellifying and stabilizing characteristics that allow this gum to maintain particles in suspension, to control fluidity and to impart an oral tactile sensation of creaminess. The k-carrageenan produces a gel texture, cohesive and very elastic, due to a strong electrostatic attraction (Nussinovitch, Citation1997). The indications of use of k-carrageenan in food products suggest amounts ranging from 0.01% (w/w) to 1.2% (w/w) of the total formulation mass. According to Glicksman (Citation1986), the best result is obtained by using 1.5% (w/w) of the mass of added water, and this was indeed the amount adopted in the formulation prepared herein. Due to being a linear polymer with thixotropic characteristics, when added to an aqueous solution k-carrageenan starts to form hydrogen bonds with the galactose moieties in its own molecule, thus increasing the volume, decreasing the free water in the system and promoting formation of double helices (Nishinari & Doi, Citation1993). In addition to k-carrageenan, locust bean gum and xanthan gum can also be included in the formulation. According to Wypych (Citation2005), the proportion of these gums that allows to obtain the maximum strength in the gel is of 50/50 (%, w/w). By using xantham gum with a high content in pyruvirate (>4%), macromolecular association with formation of microcrystalline regions is promoted, increasing the polymer–polymer affinity comparatively to the polymer–solvent (Smith et al., Citation1981), and producing a highly thermoresistant gel (Tako, Citation1991). The interaction between these solutes, added to the pullulan, make the final product more elastic, cohesive, resistant, with improved water retention, reducing syneresis and producing a creamy tactile texture in the mouth (Wypych, Citation2005). The formulation chosen was formed by high molecular weight polymers (pullulan, k-carrageenan, xanthan and locust bean gums), which produced a polymeric film in amorphous form. The next step was to develop the elasticity in the formulation, that is, to provide molecular flexibility aiming at producing molecular freedom while keeping at the same time the global cohesion of the structure (Wypych, Citation2005). The plasticizers are responsible for providing flexibility and control the microbrownian molecular motions in the structure (Wypych, Citation2005), while maintaining the hygroscopicity (Glicksman & Farks, Citation1974). To avoid the tendency of the polymeric film to lose water, an osmotically positive (hygroscopic) substance was used (Glicksman & Farks, Citation1974), that is, sorbitol (possessing humectant properties) at 0.8% (w/w) (Nussinovitch, Citation1997; Wypych, Citation2005). Hence, the formula chosen to produce the fast-dissolving buccal mucoadhesive film, based on both published data and preliminary assays, used pullulan gum (25.00%, w/w); k-carrageenan gum (1.00%, w/w); xanthan gum (0.15%, w/w); locust bean gum (0.15%, w/w); sorbitol (0.80%, w/w); and ultrapure water up to 100% (w/w), according to the procedure described in the section “Preparation of the fast-dissolving mucoadhesive film”.
Rheology and viscosity model
The intrinsic viscosity of polymers in a given solvent determines their hydrodynamic volume, being dependent on the polymer molecular mass (Wypych, Citation2005). Equation (3) expresses the intrinsic viscosity of an aqueous solution of pullulan with a molecular weight range between 48 × 103 and 2.18 × 106 g/mol at 25 °C (Kato et al., Citation1982).
The weighted average molecular weight (Mw) of the mucoadhesive film increased from 59.924 g/mol (dispersion) to 188.545 g/mol (film after drying). When comparing the values obtained in this research effort (which produced the relationship represented by Equation (4)) with the ones produced by Equation (3) (Kato et al., Citation1982), the following values of intrinsic viscosity were obtained: (i) In the dispersion, we found 33.02 cm3.g−1 comparing with 30.34 cm3.g–1 (within the range 26.89–34.22 cm3.g−1) as predicted by Equation (3); and (ii) in the dry mucoadhesive film, we found 71.61 cm3.g−1 comparing with 65.39 cm3.g−1 (within the range 57.31–74.69 cm3.g−1) as predicted by Equation (3).
The intrinsic viscosity values are an indication of the hydrodynamic volume, that is, the volumetric expansion changed from 33.02 cm3.g−1 to 71.61 cm3.g−1. The values produced in this research effort for the mucoadhesive film solution under development are in close agreement with the upper limit values encountered by Kato et al. (Citation1982). Confirmation of the hydrodynamic volume expansion is carried out by estimating the distance between the two edges of the polymeric chain, using Equation (5) (Kato et al., Citation1982), when the interactions are of the type long distance, and the expansion factor (α) can be calculated by:
where r0 is the quadratic initial average distance and r is the quadratic final average distance. The distance between the two edges of the pullulan polymeric solutions was estimated by using Equation (6), where MW is the molecular weight (Kato et al., Citation1982), producing a value of 6.7x10−17 cm2.mol.g−1
Upon substitution by the molecular weights produced in the present research effort (MW0 = 59 924 g mol−1 and MW = 188 545 g mol−1) an expansion factor equal to 1.8 was obtained, which means that the average distance between the edges of the pullulan polymeric chain increased by 1.8 times. According to Canevarolo (Citation2002), this factor depends on the type of solvent, and therefore when α = 1 the solvent is ineffective, but when α > 1 the solvent is considered to be a good one. Through viscometry the concentration can be determined at which the skeins of the macromolecules begin to intertwine (see Equation (7)), which is termed as critical overlap concentration (C*), where η is the intrinsic viscosity (Van der Maarel, Citation2008).
For the pullulan solution, the value of 0.033 g.cm−3 was obtained for the critical overlap concentration.
The order of magnitude for the decrease in the viscosity of the polymeric solution when the temperature decreases from the crystalline melting temperature to the glass transition temperature is given by the WLF (WILLIANS-LANDEL-FERRY) equation (Equation (8)) (Fennema, Citation2000):
By applying Equation (8) to the pullulan polymeric solution, where Tg is the glass transition temperature (63.08 °C), T is the crystalline melting temperature (118.18 °C), η is the viscosity of the solution at temperature T and ηg is the viscosity at temperature Tg, when substituting the temperature values, viscosity increases by at least 1 billion times (ηg = 109 η), causing immobilization of the molecules and hindering the formation of crystals; at this level of viscosity, their free Gibbs energy is negative, and because the molecules are disordered they begin to form amorphous regions (Downton et al., Citation1982; Roos, Citation1995).
The other components that were utilized were k-carrageenan gum, xanthan gum and locust bean gum, to obtain a film product suitable for oral use and with a rapid dissolution when in contact with the buccal mucosa. The interaction between these solutes makes the film product more elastic and cohesive, increases water retention, reduces syneresis and produces a creamy-like texture oral tactile sensation. This occurs due to the synergy between the different gums in the galactomannan region, which makes the gel thermoreversible and increases its viscosity (Glicksman & Farks, Citation1974; Wypych, Citation2005). k-Carrageenan gum was used in the mass proportion of 1.5 % relative to the water mass added to the initial polymeric solution. The indications for the use of k-carrageenan in foodstuff suggest amounts between 0.01% and 0.2% of the total formula mass. However, according to Glicksman and Farks (Citation1974), the best results are achieved by using 1.5% (w/w) k-carrageenan relative to the water content. This proportion was therefore adopted in the development of our formulation, proving to be most appropriate. As k-carrageenan is a linear polymer with thixotropic characteristics, when in aqueous solution it starts to form hydrogen bonds with galactose residues from its own molecule, thus resulting in a volume increase (from ∼2.4 cm3 mol−1 to 4.9 cm3 mol−1), reducing free water in the system and causing formation of double helices (Nishinari & Doi, Citation1993). The mixture of locust bean gum and xanthan gum in the proportion of 50:50 (%, w/w) of each gum proved to be adequate to obtain a maximum gel strength. The use of only high molar weight polymers (pullulan, k-carrageenan, xanthan and locust bean gums) allowed to produce a polymeric film in amorphous form. To develop the required elasticity and allow for flexibility of the mucoadhesive film, while keeping the global cohesion of the structure, addition of a plasticizer is mandatory. The function of the plasticizer used (sorbitol) was solely to maintain the dynamic balance between solvation and desolvation. The performance of the plasticizer arises from its polar groups that interact with the polar groups in the polymer, while the apolar groups function as spacers between dipoles. The plasticizer is able to enhance flexibility and hygroscopicity (Wypych, Citation2005). The substance tested for moisturizing power was also sorbitol; as it presents a low vapor pressure (19.5 mmHg, at 25 °C), a low diffusion rate and a low volatility (volatile above 160 °C), it is compatible with the main polymer in the formulation. It is hygroscopic and remains stable in the presence of acids, bases and heat. The recommended concentrations of sorbitol fall between 0.5% and 1.5% (w/w) (Nishinari & Doi, Citation1993; Wypych, Citation2005). The experimental formulation of the mucoadhesive film entertained in the present research effort led to an ideal value of 0.8 % (w/w). Hence, the aforementioned mathematical rationale together with the above considerations allowed definition of a final formula for the mucoadhesive film, that is, pullulan gum (25.00%, w/w), k-carrageenan gum (1.00%, w/w), xanthan gum (0.15%, w/w), locust bean gum (0.15%, w/w), sorbitol (0.80%, w/w) and a sufficient amount of ultrapure water up to 100% (w/w).
Polymeric solution rheology and viscosity
Manufacture of the mucoadhesive film was carried out via dissolution of the polymeric gums and the plasticizer until the intended film solution formed. Following a drying process, the film solution started with a lower initial viscosity which increased during the drying process. The average molecular weight (MW) of the mucoadhesive solution under development was mathematically calculated, changing from 59.924 g/mol (dispersion) to 188.545 g/mol (film after drying). The intrinsic viscosity determined gave an idea of the hydrodynamic volume, that is, there was a volumetric expansion from 33.02 cm3 g−1 (in the dispersion) to 71.61 cm3 g−1 (film after drying). The values determined for the product being developed were in close agreement with the upper limit values found by Kato et al. (Citation1982).
Glass transition temperature (Tg) and crystalline melting temperature (Tm)
The significance of the determination of Tg for the mucoadhesive polymer is to know if the polymer dissolution will effectively occur at the buccal cavity temperature (ranging from 35 °C up to 37 °C). The glass transition temperature of the mucoadhesive film produced in the present research effort was 63.08 °C, as can be seen from inspection of . The Tg value observed was higher than the temperature prevailing in the buccal cavity (35 °C to 37 °C). Thus, to reduce the Tg from 63 °C to 36 °C (the average temperature in the buccal cavity) and hence transforming the mucoadhesive film from a “solid” state to a gummy state, it was necessary to increase the water activity of the polymeric film. Considering an average mass of the film of 0.040 g, it would be necessary to add 0.0052 g water (see section “Glass transition temperature model”), an amount which is easily supplied by the saliva produced in the buccal mucosa. Moreover, a Tg of 63 °C, according to production logistics, is of high significance for product maintenance during its shelf life.
At the crystalline melting temperature (Tm), crystal fusion starts concomitantly with a linear thermal expansion (Canevarolo, Citation2002) and with the fusion of each small crystal causing a localized and instantaneous increase in the volume. The crystalline melting temperature of the mucoadhesive film, as can be seen in , is of 118.18 °C, indicating the existence of a low content in crystals in the film formulation, thus favoring dissolution of the mucoadhesive film in the buccal cavity.
Scanning electron microscopy (SEM) analyses and crystallization
A decrease in the cooling temperature leads to an increase in the viscosity, reducing molecular mobility and, thus, the size of the resulting crystals will be smaller, acquiring amorphous characteristics. The amorphous “crystals” are small and facilitate the dissolution upon contact with the solvent (water). In , SEM microphotographs of the mucoadhesive film produced show a large ordering of the amorphous “crystals”, and a uniform morphology. The small amorphous “crystals” allow a reduction in the (free) space between crystals on the surface of the pullulan-based mucoadhesive film. The large amount of amorphous crystals that appeared as collections of tiny grains with no defining shape or features explains, in turn, the low permeability of this polymeric gum to oxygen (14 mL•mm•m−2•day•atm) and to water vapor (0.059 mL•mm•m−2•day•atm) (Gontard et al., Citation1996).
Figure 4. Scanning electron microphotographs of the mucoadhesive film [magnification: (a) ×10 000, (b) ×20 000].
![Figure 4. Scanning electron microphotographs of the mucoadhesive film [magnification: (a) ×10 000, (b) ×20 000].](/cms/asset/65752cf3-6a6c-4196-b8d6-f86a0f2a057b/idrd_a_851301_f0004_b.jpg)
The crytical ray found in our research effort was smaller than 1.0 μm, thus in close agreement with the data published in the specialty literature for polymers (between 0.25 µm and 1.0 µm) (Carpenter & Evans, Citation1990; Guilbert et al., Citation1996) and demonstrated for pullulan.
Moisture content determinations and thermogravimetric analyses
The results obtained from the TGA analysis performed to the mucoadhesive film produced, under a controlled atmosphere, are displayed as a TGA curve in . The volatile components of the polymeric film (moisture and solvents), representing ∼13.21% (w/w) of the initial mass, were removed during the volatilization process (from 26.5 °C up to 217 °C). The devolatilization process (pyrolysis) started around 244 °C, and the highest rate of mass loss occurred in the temperature range from 244 °C up to 341 °C, with a total mass loss of ∼73.16% (w/w) due to decomposition of the organic material. The remaining mass was ∼13.63% (w/w), and consisted of ashes and inorganic materials. The moisture content of the mucoadhesive polymeric film was determined by infrared heating analyses of 10 film samples, resulting in an average moisture content of 14% (w/w) ± 1% (w/w).
Mechanical properties of the mucoadhesive film
The mechanical properties of polymers are characterized by the way these materials respond to applied mechanical solicitations, which can be of stress and/or strain types (Callister, Citation2007). Polymers, due to their molecular structures, provide a viscous behavior such as liquids, superimposed with an elastic behavior such as Hookean solids. This phenomenon is called viscoelasticity, which can be defined as the phenomenon by which the polymer displays simultaneously the characteristics of a fluid and of an elastic solid (Ferry, Citation1980). The elastic fraction of the strain arises due to variations in both the angle and bond distance between the atoms of the polymeric chain. The plastic fraction arises due to friction and flowing between polymeric molecules (Van der Maarel, Citation2008). The results from stress and strain tests carried out on the mucoadhesive film are displayed in . According to Rhim (Citation2003), amorphous pullulan films with plasticizers present values for mechanical effects of tensile strength between 29.6 N•mm−2 and 54.13 N•mm−2, and of deformation (strain) between 4.0% and 33.8%. The results obtained for the mucoadhesive film produced in the present research effort resulted in a stress of 58 N•mm−2 and in a deformation (strain) of 4.4%, values being close to the expected ranges. These results allow to characterize the mucoadhesive film produced herein as a semicrystalline or amorphous material.
The elastic module determined was 1590 ± 200 N•mm−2, for a stress of 53.0 ± 2.3 N•mm−2. The flow limit was obtained at the highest value of the curve, being of 58 N•mm−2, which is indicative that it occurs after the end of the linear elastic region. Comparing the results obtained for the mucoadhesive film produced with those of a commercial one (i.e. Listerine Citrus Oral®), it can be concluded that the commercial film reached higher tensile (stress) (95.2 N mm−2) and deformation (5.5%) values (Chen et al., Citation2006) than does the one produced in our research effort. The stress values of the film developed herein presented with a lower flow limit (58 N•mm−2) than the commercial film (92.5 N•mm−2).
Mucoadhesive film dissolution studies
The mucoadhesive film developed in this research effort presented as dimensions: thickness, 100 ± 20 µm; size, (2.0 ± 0.1 cm) width × (3.0 ± 0.1 cm) length; volume, 0.06 ± 0.018 cm3; and contact area, 6.0 ± 0.5 cm2. Commercial products of the same type present a contact area with the mucosa between 5 and 10 cm2 and can receive drug amounts up to 15 mg (Vollmer & Galfetti, Citation2006). The dissolution tests were carried out with five film samples according to the procedure described by Chen et al. (Citation2006). The average dissolution timeframes obtained were of 41.6 ± 7.9 s, hence demonstrating a fast dissolution profile. In the preparation of the mucoadhesive film under study, the pharmaceutical compound meloxicam at a dosage of 15 mg was incorporated to evaluate the dissolution time of the film with impregnated drug. The pharmaceutical compound meloxicam was incorporated during the production of the mucoadhesive film, and its addition during the production process was the only change to the production protocol described in section “Preparation of the fast dissolving mucoadhesive film”. The dissolution tests were carried out using film samples with and without impregnated drug, according to the procedure described by Chen et al. (Citation2006), in a total fivefold for each formulation. The results obtained clearly demonstrate that the film without drug exhibits a fast dissolution profile (41.6 ± 7.9 s) whereas for the film counterpart with impregnated drug the dissolution profile was much slower (6.6 ± 3.0 min). These results are in close agreement with the ones found for other (commercial) film products with impregnated drugs, that is, Ambroxol (10 mg), Dextromethorphan (10 mg) and Metoclopramd (10 mg), where the total dissolution times were lower than 5 min (Vollmer & Galfetti, Citation2006).
Conclusions
As major conclusions arising from the experimental development entertained herein, one can state that the mathematical rationale utilized for developing a pre-formulation of a mucoadhesive film proved to be fully successful, and that the mucoadhesive formulation designed and produced complied with adequate standards of similar marketed products, particularly relative to mucoadhesivity, dissolution speed, use of edible materials, resistance to gas migration, ease of molding, solubility in water and transparency. The polymeric film developed exhibited adequate characteristics to be employed as a carrier of active pharmaceutical ingredients. The successful development of novel buccal adhesive formulations requires assimilation of a great deal of information pertaining to the chemical nature, physical structure and behavior of the several components integrating the formulation of such new buccal mucoadhesives. The viscosity of the mathematically designed formulation increased when in solution and during the cooling-drying process, allowing formation of amorphous crystals which facilitate the dissolution of the film when in contact with the buccal mucosa and improve the texture of the mucoadhesive film. The glass transition temperature of the film, although higher than the average temperature prevailing in the buccal cavity, helps in maintaining the integrity of the mucoadhesive film product during storage. The Tg obtained did not impair the film dissolution process, because the difference between this temperature and the one prevailing in the buccal cavity is considered to be small and needs only a small amount of water and a low amount of heat for the film to pass from a solid state to a gummy state. Regarding crystallization, the crystals in the mucoadhesive film based on pullulan were small amorphous crystals exhibiting low empty spaces between them, which reduced the available space for the entry of gases. When assessing the mechanical resistance of the film, the resistance to strain was lower than that of commercial films, however did not interfere with the structural integrity of the film produced. The drug loading capacity of the film produced was also within the quantitative standards found in the specialty literature. Notwithstanding the above considerations, for the formulation developed in the research effort entailed herein to be made commercially available, it is necessary to carry out additional studies, both in vitro and in vivo, focused on the application of several (different) pharmaceutical compounds and performing extensive bioavailability and stability assays.
Declaration of interest
The authors report no declarations of interest.
Financial support to Victor M. Balcão, via an Invited Research Scientist fellowship (FAPESP Ref. No. 2011/51077-8) by Fundação de Amparo à Pesquisa do Estado de São Paulo (FAPESP, São Paulo, Brazil), is hereby also gratefully acknowledged. The authors are also grateful to CNPq and FAPESP for financial support.
Acknowledgements
The authors are grateful to Prof. Carol Hollingworth Collins, affiliated with the Institute of Chemistry of the University of Campinas (Campinas, Brazil), for English revision of the manuscript. The authors are also grateful to Cláudio M. Barroso (BSc.), Graphic Designer at University of Sorocaba (UNISO), for computer-designing the schemes/drawings integrating this scientific paper.
References
- ASTM. (1999). Annual book of ASTM standards. Method D 3418-99: method for transition temperatures of polymers by differential scanning calorimetry. West Conshohocken, PA: ASTM International
- Burnett D, Malde N, Williams D. (2009). Characterizing amorphous materials with gravimetric vapour sorption techniques. Pharma Technol Europe 21:41–5
- Callister JWD. (2007). Ciência e engenharia de materiais: uma introdução. Rio de Janeiro: LTC
- Canevarolo SV. (2002). Ciência dos polímeros. São Paulo: Artliber
- Carpenter JC, Evans TL. (1990). Use of cyclic carbonate oligomers in the synthesis of bisphenol-A polycarbonate/polydimethylsiloxane copolymers. Polym Prep 31:18--19
- Carvalho FC, Bruschi ML, Evangelista RC, Gremião MPD. (2010). Mucoadhesive drug delivery systems. Braz J Pharm Sci 46:1–17
- Chen MJ, Tirol G, Schmitt R, et al. (2006). Film forming polymers in fast dissolve oral films (T3200). Poster presented at the 2006 Annual Meeting and Exposition of the American Association of Pharmaceutical Scientists; 2006. Oct. 29--Nov. 2; San Antonio, Texas, USA
- Cheng KC, Demirci A, Catchmark JM. (2011). Pullulan: biosynthesis, production, and applications. Appl Microbiol Biotechnol 92:29–44
- Deshmukh GD, Varma MM, Manjunath SY. (2011). Development and evaluation of propranolol hydrochloride buccal mucoadhesive gel using natural mucoadhesive agent obtained from the fruits of Ficus carica L. Am J Pharm Tech Res 1:135–45
- Dixit R, Puthli S. (2009). Oral strip technology: overview and future potential. J Control Release (Mumbai, India) 139:94–107
- Downton GE, Floresluna JL, King CJ. (1982). Mechanism of stickiness in hygroscopic, amorphous powders. Ind Eng Chem Fund 21:447–51
- Fennema OR. (2000). Water and ice. In: Fennema OR, ed. Food chemistry, 3rd ed. New York: Marcel Dekker
- Ferry JD. (1980). Viscoelastic properties of polymers, 3rd ed. New York: John Willey & Sons, 529
- Glicksman M. (1986). Food hydrocolloids, Vol. III. Boca Raton, FL: CRC Press, pp. 3–121
- Glicksman M, Farks HE. (1974). Gum gelling system: xanthan-tara dessert gel. U.S. Patent No. 3,784,712
- Gontard N, Thibault R, Cuq B, Guilbert S. (1996). Influence of relative humidity and film composition on oxygen and carbon dioxide permeabilities of edible films. J Agric Food Chem 44:1064–9
- Guilbert M, Vinouze B, Trubert C, Bosc D. (1996). Validation of a scattering state model for liquid crystal polymer composites. Appl Phys Lett USA 68:2498
- Gupta MM, Patel MG, Kedawat M. (2011). Enhancement of dissolution rate of rapidly dissolving oral film of meclizine hydrochloride by complexation of Meclizine hydrochloride with β-cyclodextrine. J Appl Pharm Sci 1:150–3
- Jyoti A, Gurpreet S, Seema S, Rana AC. (2011). Fast dissolving films: a novel approach to oral drug delivery. Int Res J Pharm 2:69–74
- Kato T, Okamoto T, Tokuya T, Takahashi A. (1982). Solution properties and chain flexibility of pullulan in aqueous solution. Biopolymers 21:1623–33
- Kharenko EA, Larionova NI, Demina NB. (2009). Mucoadhesive drug delivery systems. Pharm Chem J 43:200–8
- Kumar D, Saini N, Pandit V, Ali S. (2012). An insight to pullulan: a biopolymer in pharmaceutical approaches. Int J Basic Appl Sci 1:202–19
- Leathers TD. (2003). Biotechnological production and applications of pullulan. Appl Microbiol Biotechnol 62:468–73
- Lee JW, Park JH, Robinson JR. (2000). Bioadhesive-based dosage forms: the next generation. J Pharm Sci 89:850–66
- Levine H, Slade L. (1992). Glass transitions in foods. In: Schwartzberg HG, Hartel RW. eds. Physical chemistry of foods. New York: Marcel Dekker, 83–221
- Mishra B, Vuppu S, Rath K. (2011). The role of microbial pullulan, a biopolymer in pharmaceutical aaproaches: a review. J Appl Pharm Sci 1:45–50
- Morales JO, McConville JT. (2011). Manufacture and characterization of mucoadhesive buccal films. Eur J Pharm Biopharm 77:187–99
- Nagai T, Machida Y, Suzuki Y, Ikura H. (1981). Method and preparation for administration to the mucosa of the oral or nasal cavity. United States Patent no. 4,250,163, Feb 10, 1981
- Nishinari K, Doi E. (1993). Food hydrocolloids: structures, properties and functions. New York: Plenum Press
- Nussinovitch A. (ed.) (1997). Seed gums. In: Hydrocolloid applications -- gum technology in the food and other industries. Blackie Academics & Professional. New York: Chapman & Hall, Chapter 8, 140--51
- Ordóñez JA. (2005). Tecnologia de alimentos. Porto Alegre: Artmed
- Rhim JW. (2003). Characteristics of pullulan-based edible films. Food Sci Biotechnol 12:161–5
- Roos YH. (1995). Characterization of food polymers using state diagrams. J Food Eng 24: 339–60
- Scott R, Cade D, Xiongwei HE. (2001). Pullulan film compositions with improved surface properties, European Patent Application EP1157691 (http://www.freepatentsonline.com/EP1157691A1.html)
- Seo S, Lee CS, Jung YS, Na K. (2012). Thermo-sensitivity and triggered drug release of polysaccharide nanogels derived from pullulan-g-poly(L-lactide) copolymers. Carbohydr Polym 87:1105–11
- Smith IH, Symes KC, Lawson CJ, Morris ER. (1981). Influence of the pyruvate content of xanthan on macromolecular association in solution. Int J Biol Macromol 3:129–34
- Tako M. (1991). Synergistic interaction between a deacylated xanthin and galactomannan. Carbohydr Polym 16:239–52
- Van der Maarel JRC. (2008). Introduction to biopolymer physics. Singapore: World Scientific
- Villanova JCO, Oréfice RL, Cunha AS. (2010). Aplicações farmacêuticas de polímeros [Pharmaceutical applications of polymers]. Polímeros: Ciência e Tecnologia 20:51–64
- Vollmer U, Galfetti P. (2006). Rapid film: oral thin films as an innovative drug delivery system and dosage form. Drug Dev Report 5:64–7
- Wypych G. (2005). Handbook of plasticizers. Toronto: William Andrew Publishing and Chemtec Publishing
- Xiao Q, Lim LT, Tong Q. (2012). Properties of pullulan-based blend films as affected by alginate content and relative humidity. Carbohydr Polymers 87:227–34