Abstract
Context: Piroxicam (PXM), a non-steroidal anti-inflammatory drug which is poorly soluble in water and ulcerogenic. Milk has been used against the gastric disturbances caused by non-steroidal anti-inflammatory drugs. In this study, skimmed milk (SKM) is used as the carrier for inclusion complex (IC) due to its surface active agent and amino acid content.
Purpose: To enhance the solubility, dissolution rate and prevent ulcerogenicity of PXM though IC with SKM.
Methods: IC of PXM were prepared with SKM by solvent evaporation method using rota evaporator and were evaluated for solubility, dissolution, solid state characterization, drug excipient interaction, rat intestinal permeation, ulcerogenicity and histopathological studies.
Results: Solubility of PXM was enhanced 2.5 times with IC. The dissolution release and amount of PXM permeated through rat small intestine was enhanced significantly with IC. Decreases in the gastric lesion index values of IC were observed than physical mixture (PM) and free PXM. The histopathological studies revealed significant reduction in ulceration in rat stomach after treatment with IC.
Conclusion: It is concluded that SKM is a good carrier to prepare IC of PXM for oral administration.
Introduction
Although oral nonsteroidal anti-inflammatory drugs (NSAIDs) are valuable in the management of a variety of acute and chronic pain, fever and inflammation conditions, their use has been coupled with the generation of gastric ulcers, which is the most frequent adverse effect and have poor solubility (Bulbena et al., Citation1993; Reynolds, Citation1993). The most important mechanism of action of all NSAIDs is the inhibition of the biosynthesis of prostaglandins by inhibiting the activity of cyclooxygenase enzyme (Vane, Citation1971, Citation1998; Simon et al., Citation1998). In order to enhance solubility, dissolution release, bioavailability and modulation of ulcerogenicity of NSAIDs numerous strategies have been reported, such as inclusion complex (IC) with β-cyclodextrine (Ramprakash & Mangal, Citation2005; Ibrahim et al., Citation2010), addition of surface active agents and formation of water soluble salts (Topaloglu, Citation1981), solid lipid microparticles (Chime et al., Citation2013), drug–dextran conjugates (Savita et al, Citation2008, Citation2009; Shrivastava et al., Citation2010; Muhammad et al., Citation2011), pro-drugs (Rasheed et al., 2010, Citation2011) drug–bismuth complex (Abuznaid et al., Citation2008), suspensions (Hongyu et al., Citation2007), IC or solid dispersion with skimmed milk (SKM) (Topaloglu et al., Citation1997, Citation1999; Topaloglu & Yener, Citation1999; Vijaya Kumar & Mishra, Citation2006).
Piroxicam (PXM) has low solubility, limited absorption, less bioavailability and it causes severe gastric ulceration on oral administration (Avila et al., Citation1996). In order to reduce gastro-intestinal side effects of non-steroidal anti-inflammatory drugs, amino acids or their salts have been recommended as additives in the intragastric application (Urushidani et al., Citation1977; Topaloglu et al., Citation1999). In this study, SKM has been chosen as carrier to form IC due to its surface active agent and amino acid content (Dickinson & Stainsby, Citation1988; Topaloglu et al., Citation1999). Solubility, dissolution release studies, isolated rat intestinal permeation studies have been conducted to confirm enhanced solubility, dissolution and permeation characteristics of PXM in the form of IC and physical mixture (PM) with SKM. Differential scanning calorimetry (DSC), X-ray powder diffraction (X-RPD) and scanning electron microscopic (SEM) analysis were performed to determine solid state properties of the drug in PM and IC in comparison with the free PXM. Gastric tolerance study also performed to know the ulcerogenicity of IC, PM and free PXM in Wistar rats.
Materials and methods
Materials
PXM was a gift sample from Apex Laboratories, Gujarat, India. SKM with maximum 0.5% fat, 4% carbohydrate was purchased from local departmental store, Hyderabad, India. All other chemicals and solvents used were of analytical grade.
Preparation of SKM powder
Accurately measured volume (100 ml) of SKM was dried at 40 °C, 100 rpm under vacuum for 40–60 min using rotary vacuum evaporator (Hei-VAP advantage/561-01300, Heidolph, Germany). The obtained sticky semisolid mass was placed in desiccator for 48–72 h to get free flowing powder (100 ml milk yielded about 10.11 g powder) and passed through a sieve # 65 and stored in well tight container till further use (Vijaya Kumar & Mishra, Citation2006).
Preparation of PXM loaded IC
Accurately weighed (1 g) PXM was mixed with SKM (10, 20, 30, 40, 50, 60 ml) in a water bath at a temperature of 40 °C and stirred for 30 min using a magnetic stirrer. The resulting suspension was subjected for same procedure described above to obtain well-dried free flowing PXM loaded IC powder (Vijaya Kumar & Mishra, Citation2006).
Preparation of PMs
Weighed amount of PXM (1 g) was uniformly mixed with 1, 2, 3, 4, 5 and 6 g of SKM powder separately using a mortar and pestle. The prepared mixtures were kept in a desiccator over calcium chloride at room temperature for 72 h and passed through sieve #65 and stored in well tight container until further use.
Solubility studies of IC and PM
Excess amounts (100 mg) of PXM, its IC and PMs with SKM were added in 2 ml clicklok microcentifuges (Tarsons Products Pvt. Ltd., Kolkata, India) filled with 1 ml of pH 1.2 buffer (SGF) and they were kept in a orbital shaking incubator at 25 °C for 48 h. The solutions were filtered through nylon membrane filter (pore diameter: 0.2 μm and membrane diameter: 13 mm) using a syringe filter unit. The samples were diluted appropriately and drug dissolved was measured using UV-visible spectrophotomer at 331 nm (UV 3200, Labindia, Mumbai, India).
In-vitro dissolution release study
The dissolution rates of PXM pure drug (10 mg), dose (10 mg) equivalent amount of PXM-loaded IC and PM was determined using the USP dissolution apparatus II (DS 8000, Labindia, India). The dissolution medium was simulated gastric fluid (SGF) at 37 °C and stirred at 100 rpm. Samples of the dissolution medium were withdrawn at predetermined time intervals (5, 10, 15, 30, 45, 60, 90, 120 min), filtered through Millipore membrane of 0.2 mm pore diameter and assayed for the drug content using UV-visible spectrophotomer at 331 nm (UV-3200, Labindia, India).
Dissolution efficiency (DE) was calculated from the area under the dissolution curve (measured using the trapezoidal rule) and expressed as a percentage of the area of the rectangle described by 100% dissolution in the same time (Sammour et al., Citation2006; Alekhya et al, Citation2012). The relative dissolution rate (RDR) was determined by dividing the DE of PM and IC with PXM (control) separately (Costa & Sousa Lobo, Citation2001; Valleri et al., Citation2004; Menegola et al., Citation2007).
For the comparison of dissolution release data, similarity factor (f2) was calculated using following equation:
where Rt and Tt are the cumulative percentage dissolved at each of the selected n time-points of the reference and test product, respectively. Factor f2 is inversely proportional to the average squared difference between the two profiles, with emphasis on the larger difference among all the time-points. The factor f2 measures the closeness between the two profiles. In dissolution profile comparisons, especially to assure similarity in product performance, regulatory interest is in knowing how similar the two curves are, and to have a measure which is more sensitive to large differences at any particular time-point. When the two profiles are identical, f2 = 100. An average difference of 10% at all measured time-points results in a f2 value of 50. Food and drug administration has set a public standard of f2 value between 50 and 100 indicate similarity between two dissolution profiles (Dave et al., Citation2004).Solid state characterization
Differential scanning calorimetry
To determine the crystallinity and characterize the solid state interaction between PXM and SKM 3 mg each of the PXM, SKM powder, PM and IC were weighed separately, placed in aluminium pans and analyzed using a differential scanning calorimeter (Shimadzu DSC-60, Tokyo, Japan). The scanning speed was 5 °C/min in the range 0–300 °C.
X-ray diffraction
X-ray diffraction patterns of PXM, SKM powder, PM and IC was recorded with an X-ray diffractometer (Shimadzu, Maxima XRD-7000, Tokyo, Japan) under conditions of “Cu” radiation target, nickel filter, 40 kV voltage, 30 mA current with a scan range of 10–80° with a scan speed of 3°/min and an intensity range of 0–1500 counts.
Scanning electron microscopy
To determine the surface morphology of PXM, SKM powder, PM and IC were analyzed using a SEM (Joel 1200 EX-11, Tokyo, Japan) under various magnifications.
Fourier transform infra-red spectroscopy
Fourier transform infra-red spectra of PXM, SKM powder, PM and IC were subjected to FTIR analysis (Bruker Alpha-T-1020, Ettlingen, Germany). Potassium bromide pellets were prepared on KBr press. The samples were prepared using KBr discs by means of KBr pellet press at a pressure of 8 tons. The spectra were recorded over the range of 4000 cm−1 to 400 cm−1.
Stability studies
Stability studies were carried out according to ICH guidelines by storing the optimized formulation at 40 °C and 75% ± 5% RH for a period of three months. The sample was withdrawn after 90 days and analyzed for drug content and in vitro release studies.
Ex-vivo rat intestinal permeation studies
Male and female Wistar albino rats weighing between 150 and 200 g were used in the study. The animals were housed in separate cages and maintained under controlled temperature, relative humidity and illumination cycle set to 12 h dark and 12 h light. The rats had free access to water and food until they were sacrificed. The study was performed with the earlier approval of Institutional Animal Ethical Committee, School of pharmacy Anurag Group of Institutions, Hyderabad, India. The animals were sacrificed with excess ether inhalation. The abdomen was opened and an ileum portion was isolated and all mucus and adhered contents were cleaned with freshly prepared Krebs–Ringer solution. One end of the ileum portion was tied and 10 mg of PXM, equivalent amount of PM and IC was introduced into the separate lumen of ileum and was tightly closed. The prepared tissue was kept in an organ bath with continuous aeration and maintained at a temperature of 37 °C. The receptor compartment consists of 150 ml of phosphate buffer pH 6.8. At predetermined time intervals, 1 ml of sample was collected and replenished with equal volume of fresh medium. The samples were treated with an equal volume of methanol, centrifuged and the supernatant was estimated for PXM using UV-visible spectrophotometer (UV-3200, Labindia, India) at 331 nm. Free PXM (drug dispersion) was considered as control for comparison (Pradip et al., Citation2006).
The steady state flux (Jss) was calculated from the slope of the linear portion of the cumulative amount permeated per unit area versus time plot. The permeability coefficient (Kp) was calculated from Jss and initial concentration of PXM. ER of PM and IC was calculated from Jss of the pure PXM (Alekhya et al., Citation2012).
Assessment of ulcerogenecity
Male and female Wistar rats (100–200 g) were divided into three groups each consists of six animals. Group I served as the standard (PXM 80 mg/kg body weight); group II served as PM test group (80 mg equivalent amount of PM); group III served as the IC test group (80 mg equivalent amount of PXM-loaded IC). The animals were fasted for 72 h before the test substance administration but had free access to water. The test substance was administered by oral gavage to each rat as a single dose, using an intubation needle fitted onto a syringe of appropriate size. The dose administered to each individual rat was calculated according to its body weight recorded on the day of test substance administration. The study was performed with the approval of Institutional Animal Ethical Committee, School of Pharmacy, Anurag Group of Institutions, Hyderabad, India. Following completion of the experiment, rats were killed using ether anesthesia and stomach was taken for macroscopic scoring and histopathological studies.
Macroscopic scoring
Animals were sacrificed 6 h after administration, the stomachs were removed, cleaned with isotonic saline solution and were incised along the greater curvature. The ulcers were examined under a magnifying lens and were measured with the help of digital vernier caliper (CD-6”CSX, Mitutoyo Corp., Kawasaki, Japan). The number of erosions per stomach was assessed for severity according to the scoring system described elsewhere (Skiba et al., Citation1995; Sammour et al., Citation2006). The gastric lesion index (GLI) caused by PXM, PM and IC was calculated using an arbitrary score (AS). AS of 0 was given to no lesions/ulcers, 0.5 was for one or more hemorrhagic ulcers of length < 1 mm, 1 was given for one or more hemorrhagic of length between 1 and 2 mm and 2 indicated one or more hemorrhagic ulcers of length > 2 mm. The mean AS for each group were calculated and expressed as the ulcer index (GLI)
Values are given as arithmetic mean ± SD.
The significance of differences between means was evaluated by one way analysis of variance (ANOVA) followed by Newman-Keuls Multiple Comparison Test with Instat Graphpad prism software (version 4.00; GraphPad Software, San Diego, CA).
Histopathological study
For the microscopic study, three representative animals of each group, selected according to the highest mean-GLI calculated in the previous study, were adequately processed; their stomachs were isolated and characteristic corpus gastric samples were isolated and placed in 10% buffered formaldehyde, dehydrated by increasing concentrations of ethanol, and embedded in paraffin. Thereafter, sections of tissue were cut at 5 µm with a Leica Ultracut (Leica Microsystems, Wetzlar, Germany), mounted on clean glass slides and dried overnight at 37 °C. Sections were cleared, hydrated, and stained with hematoxylin and eosin for histological evaluation of gastric damage. All tissue sections were examined in Metzer-M microscope (Vision Plus-5000 BM, Metzer Optical Pvt., Ltd, Mathura, India).
Results and discussion
Milk consists of water (88%), lactose (5%), fat (3%), protein (3.3%), and minerals (0.7%). Milk can be described as a multiple component system in which proteins and fat globules form colloidal particles dispersed in the aqueous phase (Fox & Mc Sweeny, Citation1998). The fat content of the milk can be simply isolated by gravity or centrifugation. The protein portion of SKM is composed of 80% caseins and 20% whey proteins. The principle components of caseins and whey proteins are αs1-, αs2-, β-, and κ-casein and α-, β-lactoglobulin and bovine serum albumin, respectively (Atherton & Newlander, Citation1977; Fox & Mc Sweeny, Citation1998; Walstra et al., Citation1999; Gaucheron, Citation2005; Walstra et al., Citation2006; Livney, Citation2010). The major protein fractions of SKM are casein. The majority of the casein proteins of SKM available in the form of colloidal particle identified as the casein micelle. It is implicit that these micelles encapsulate hydrophobic PXM inside with its surface active property improves its solubility and dissolution release in gastric or dissolution media. These drug entrapped micelles has a porous nature, which may responsible for the release of encapsulated PXM molecule from the IC into media. The other model also recommended is casein submicelle model. According to this model, there are small aggregates of whole casein, consisting of 10–100 casein molecules, called submicelles (Atherton & Newlander, 1977; Walstra, 1990; Walstra et al., 1999). It is assumed that there are two different types of submicelle: with and without κ-casein. Submicelle rich in κ-casein absorb surface location and show surface activity. The second principle protein fractions of SKM are whey proteins, which are surface active molecules and more aqueous soluble than casein (Atherton & Newlander, Citation1977; Fox & Mc Sweeny, Citation1998; Walstra et al., Citation1999, Citation2006; Sahin & Arslan, Citation2007).
However, in this present research work we have not evaluated which component in SKM responsible more to formation of an IC, we expect that casein micelles and whey proteins are concerned as they have significant surface activity to solubilize PXM entrapped in micelles and associating with hydrophilic whey protein portions. In this study, SKM was selected as carrier for formulation of PM and IC of PXM to improve solubility, release profile and reduce ulcerogenicity of PXM.
Drug content, solubility and dissolution release studies
Drug content data for the PM and IC formulations are depicted in . From the results it has shown that the drug content in the formulated IC and PM was within the range of ±3% theoretical amount, indicating the method adopted for formulation was suitable and reproducible. Free PXM was found to have a solubility of 0.064 mg/ml. The solubility was enhanced (0.16 mg/ml) 2.5-fold using the IC technique (IC4, ). Likewise, the solubility of PXM was increased (0.087 mg/ml) 1.4-fold in PM4. The enhanced aqueous solubility of PXM observed with IC was possibly due to a reduction in particle size and formation of an amorphous state, while in the case of PM, the surface active agents and amino acid content of the milk may be responsible for better solubility. The hydrophilic casein micelles entrap hydrophobic PXM, thus improving the aqueous solubility (Vijaya Kumar & Mishra, Citation2006; Ankush et al., Citation2012). The results suggest that the SKM was able to enhance the solubility of PXM in enzyme free SGF. The dissolution profiles of the PM and IC formulations as compared to free PXM are depicted in , respectively. Free drug showed a percentage cumulative drug release (% CDR) of only 9.59 ± 0.8 within15 min. The % CDR of PM formulations ranged from 20.91 ± 1.38 to 43.50 ± 1.59, whereas in case of IC it varied from 24.34 ± 3.19 to 99.21 ± 2.82. The results showed that the % CDR from IC and PM was 2–9 times higher than free PXM within 15 min. The best % CDR was shown by formulations IC4 and PM4, 96.74 ± 2.30 and 41.0 ± 5.12, respectively, with in 15 min. It is evident that the % CDR continues to enhance with the increasing amounts of SKM in the formulations. However, beyond drug SKM ratio of 1:4, the % CDR was not considerably improved. This may be because of higher quantities of carrier involved which may take more time to dissolve (Gaucheron, Citation2005; Ahire et al., Citation2010). The IC showed higher % CDR in comparison to PM because the presence of carrier also prevents aggregation of fine drug particles, increasing dissolution of the drug. Furthermore, the presence of carrier inhibits the crystal growth of the drug assisting in faster dissolution (Patel et al., Citation2008). Accordingly DE was also significantly higher for IC formulations compared to free PXM in enzyme free SGF medium (p < 0.01) (). The RDR greater than one indicates dissolution enhancement and in this work noticed more than one for IC formulations (). Interestingly, the IC formulation (IC4) increased dissolution of PXM compared to other formulations. The in-vitro studies revealed that IC4 and PM4 were best of all the formulations. The solubility and dissolution rates exhibited by these formulations were maximum and better than other formulations. Therefore, IC4 and PM4 were selected for further studies.
Figure 1. In-vitro dissolution release profiles of (A) free PXM versus PM with skimmed milk (B) free PXM versus IC with skimmed milk.
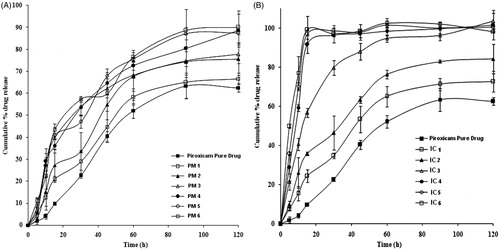
Table 1. Formulation chart of PXM-loaded IC and PM.
Solid state characterization
The mechanism of improved dissolution rate of PXM from IC, the solid-state properties of this system was investigated by means of DSC, XRD and SEM.
Differential scanning calorimetry
In order to determine the solid state of the PXM in PM and IC with SKM, DSC studies were performed on the PXM, PM and IC separately. As shown in , the DSC curve of PXM showed one endothermic peak at 204.7 °C, corresponding to its melting point, whereas SKM’s peaks has been shown to be at 148.6 and 208.3 °C. The DSC plots of the PM and IC showed almost all similar two broad peaks at about 144.0 and 200.0 °C. As the DSC peak of the IC is broad, it could be concluded that the complete conversion of crystalline state of PXM to amorphous state in IC.
X-ray diffraction
shows the X-RPD of the free PXM, SKM, PM and IC. Considerably different X-RDPs are to be predicted if an IC is formed, because crystal structure will be altered (Green et al., Citation1991; Khan et al., Citation2001). In our study, it was noticed that most of the diffraction patterns of the PM were simply superimposition or summation of the PXM and SKM, with similar sharp peaks, similar d values and other characteristics as that of pure components. However, some alterations like peak positions, diminutions in peak intensities, and little disparity in d values were noticed diffractograms of the PM (), demonstrating the possibility of interactions between SKM and PXM. On the other hand, the study of changes in peak positions, appearances, disappearances and relative intensity of the peaks of the ICs established somewhat soft diffraction patterns with considerable differences than those of PM and individual constituents. Additionally the d values of the IC were completely different from that of PXM and SKM (), which further supports the evidence that complexation did takes place between PXM and SKM. The X-RDPs further showed that the crystalline peaks in the diffractograms of the IC were wider and decreased intensities, indicating the amorphous nature of the PXM in the final IC. These results are accordance with those, previously explained by the DSC study. This could be recognized to the molecular inclusion of the PXM molecules in the center hydrophobic core of the SKM casein micelles leading to the inhibition of crystallization of the PXM.
Figure 3. XRD patterns of (A) free PXM, (B) skimmed milk powder, (C) physical mixture and (D) inclusion complex.
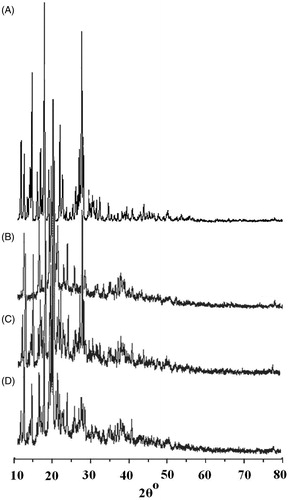
Table 2. X-ray powder diffraction of piroxicam, skimmed milk, PM, IC expressed as 2θ, peak intensity, width, inter planar distance and relative diffraction intensity.
Scanning electron microscopy
The SEM results in show that in the case of PM, the particle size of PXM is almost the same, and on few SKM particles crystals of PXM can be seen. In IC, PXM particles are in amorphous form, which concludes that decreasing in particle size was accomplished.
Fourier transform infra-red spectroscopy
The FTIR spectra of samples are shown in . FTIR spectra of PXM exhibits characteristic peaks of C–H stretching, aromatic CH bending, C–S stretching, C=C, C=N ring stretching, asymmetric S(=O)2 stretching, symmetric S(=O)2 stretching, secondary amine N-H stretching at 2930, 830, 773, 618, 1630, 1434, 1529, 1351, 1149 and 3338 wave numbers respectively (). In the spectra of IC optimized formulation (), the peaks characteristic to the SKM were present at almost same positions, where as PXM peaks were also present, but at a reduced rate of absorption, indicating the trapping of the drug inside the carrier matrix. None of the spectra showed any peaks other than those assigned to PRX drug and SKM, which indicates the absence of chemical interactions.
Figure 5. FTIR spectra of (A) free PXM, (B) skimmed milk powder, (C) physical mixture and (D) inclusion complex.
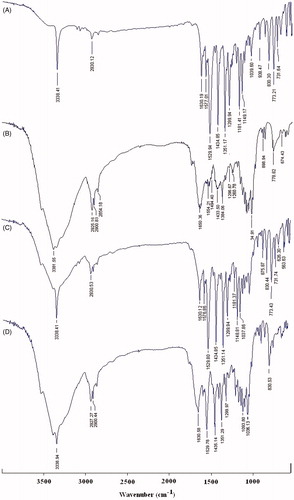
Table 3. Characteristic peaks of free PXM, skimmed milk, PM and IC with skimmed milk in FTIR spectra.
Ex-vivo intestinal permeation studies
The cumulative amount of drug permeated (Q) was plotted against time ( and ). The steady state flux (Jss) calculated for the PXM, PM and IC were 0.00084, 0.00108 and 0.00152 µg/cm2 h−1, respectively. The permeability coefficient (Kp) of the drug through intestine was found to be 0.84, 1.08, 1.52 cmh−1 for PXM, PM and IC, respectively. The ER values for PM and IC were found to be 1.29 and 1.80, respectively, which indicates that the IC permeated more than PM than PXM.
Figure 6. Ex-vivo permeation of PXM across rat intestine from physical mixture (PM4) inclusion complex (IC4) with skimmed milk (mean ± SD, n = 3).
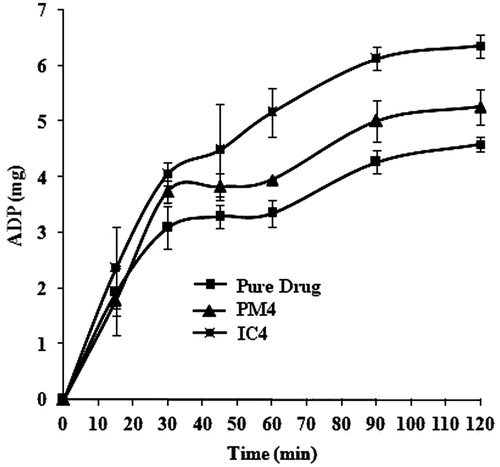
Table 4. Permeation parameters of PXM, PM and IC with skimmed milk.
Stability studies
To determine the change in dissolution release profile and drug content on storage, a temperature sensitivity study of IC4 and PM4 was performed at 40 °C and 75% ± 5% RH for three months. Sample tested after 3 months showed no significant change in drug content and dissolution release profile. The similarity factor (f2) for the IC4 and PM4 was 85.34 and 83.47, respectively (), indicating close proximity of dissolution release profiles before and after temperature sensitivity studies. Drug content data of after storage compared with before storage using paired t test as shown in , indicating an insignificant (p > 0.05) difference.
Table 5. Results of stability of piroxicam in inclusion complex (IC4) and PM4.
Assessment of ulcerogenecity
Macroscopic scoring
As shown in the average lesion index is higher for free PXM (40.33 ± 10.68) than for the PM (30.33 ± 7.94) and for the IC with SKM (25.00 ± 3.94). However, the difference between the GLI of normal control and free PXM is significant (p < 0.001), free PXM and IC is significant (p < 0.01), whereas the difference between PM and IC is not significant. The macroscopic images of individual stomach from each group were represented in .
Histopathology
The histological pattern of the mucosal specimens was studied by examining the histology of the treated and control samples. Stomach tissue with normal histological pattern observed in normal rats (), Stomach tissue with mucosal ulceration was observed in PXM-treated rats () with a complete loss of the mucosa and neutrophil infiltration and fibrin deposition. At the periphery of the ulcer, the adjacent squamous epithelium shows regenerative changes. On the other hand, the mucosal ulceration in groups treated with PM4 () was with a partial loss of mucosa with fibrin deposition and mild neutrophil infiltration. The specimens from rats treated with IC4 () revealed no significant ulcerations and the tissues were almost intact.
Conclusion
In conclusion, the present investigations suggest that the IC of PXM with SKM is useful carrier for oral administration of PXM with improved solubility, dissolution release rate and reduced gastro-intestinal complications.
Declaration of interest
The authors report no conflicts of interest. The authors alone are responsible for the content and writing of the paper.
Acknowledgements
Authors acknowledge Apex Health Care Pvt Ltd Ankleshwar, India, for gift sample of Piroxicam. Authors also thankful to Dr. Rajeshwar Reddy, Chairman, School of Pharmacy (Formerly Lalitha college of Pharmacy), Anurag Group of Institutions for providing research facilities. Appreciation is also extended to Dr. A. Madhu Babu and A. Padmanabha Rao for their invaluable assistance.
References
- Abuznaid M, Sallam AS, Hamdan I, et al. (2008). Diclofenac-bismuth complex: synthesis, physicochemical, and biological evaluation. Drug Dev Ind Pharm 34:434–44
- Ahire BR, Rane BR, Bakliwal SR, Pawar SP. (2010). Solubility enhancement of poorly water soluble drug by solid dispersion techniques. Int J Pharm Tech Res 2:2007–15
- Alekhya G, Raju J, Sharan RB, et al. (2012). Improved oral delivery of valsartan from maltodextrin based proniosome powders. Adv Powder Technol 23:583–90
- Ankush C, Avtar CR, Geeta A, et al. (2012). Development and characterization of an atorvastatin solid dispersion formulation using skimmed milk for improved oral bioavailability. Acta Pharm Sin B 2:421–8
- Atherton HV, Newlander JA. (1977). Chemistry and testing dairy products. 4th ed. Westport: AVI Publications
- Avila JR, de la Lastra CA, Martin MJ, et al. (1996). Role of endogenous sulphydryls and neutrophil infiltration in the pathogenesis of gastric mucosal injury induced by piroxicam in rats. Inflamm Res. 45:83–8
- Bulbena O, Escolar G, Navarro C, et al. (1993). Gastroprotective effect of zinc acexamate against damage induced by nonsteroidal anti-inflammatory drugs: a morphological study. Dig Dis Sci 38:730–9
- Chime SA, Attama AA, Builders PF, Onunkwo GC. (2013). Sustained-release diclofenac potassium-loaded solid lipid microparticle based on solidified reverse micellar solution: in vitro and in vivo evaluation. J Microencapsul 30:335–45
- Costa P, Sousa Lobo JM. (2001). Modeling and comparison of dissolution profiles. Eur J Pharm Sci 13:123–33
- Dave BS, Amin AF, Patel MM. (2004). Gastroretentive drug delivery system of ranitidine hydrochloride: formulation and in vitro evaluation. AAPS Pharm Sci Tech 5:Article 34:1--6
- Dickinson E, Stainsby G. (1988). Advances in food emulsions and foams. London: Elsevier Applied Science
- Fox PF, Mc Sweeny PLH. (1998). Dairy chemistry and biochemistry. 1st ed. New York: Springer
- Gaucheron F. (2005). The minerals of milk. Reprod Nutr Dev 45:473–83
- Green AR, Mullar ES, Guillory JK. (1991). Physical properties of the complex formed between heptakis-(2, 6-di-o-methyl)-β-cyclodextrin, β-cyclodextrin and chlorambucil. J Pharm Sci 80:186–9
- Hongyu P, Akihiko H, Hideakira Y, et al. (2007). Reduction of gastric ulcerogenicity during multiple administration of diclofenac sodium by a novel solid-in-oil suspension. Pharm Dev Technol 12:321–5
- Ibrahim AA, Ahmed MO, Fars KA, et al. (2010). Influence of cyclodextrin complexation with NSAIDs on NSAID/cold stress-induced gastric ulceration in rats. Int J Med Sci 7:232–9
- Khan GM, Wazir F, Zhu JB. (2001). Ibuprofen-β-cyclodextrin inclusion complexes: evaluation of different complexation methods. The Sciences 1:193–9
- Livney YD. (2010). Milk proteins as vehicles for bioactives. Curr Opin Colloid Interface Sci 15:73–83
- Menegola J, Steppe M, Schapoval EE. (2007). Dissolution test for citalopram in tablets and comparison of in vitro dissolution profiles. Eur J Pharm Biopharm 67:524–530
- Muhammad AH, Zahid H, Muhammad TH, et al. (2011). Fabrication of potential macromolecular prodrugs of aspirin and diclofenac with dextran. Pak J Pharm Sci 24:575–81
- Patel M, Tekade A, Gattani S, Surana S. (2008). Solubilityenhancement of lovastatin by modified locust bean gum using solid dispersion techniques. AAPS Pharm Sci Tech 9:1262–9
- Pradip KG, Rita JM, Manish LU, Rayasa SRM. (2006). Design and development of microemulsion drug delivery system of acyclovir for improvement of oral bioavailability. AAPS Pharm Sci Tech 7:E1–E5
- Ramprakash G, Mangal SN. (2005). Formulation studies and in vivo evaluation of a flurbiprofen-hydroxypropyl β-cyclodextrin system. Pharm Dev Technol 10:105–14
- Rasheed A, Ashok Kumar CK, Mishra A. (2011). Synthesis, hydrolysis studies and phamacodynamic profiles of amide prodrugs of dexibuprofen with amino acids. J Enzyme Inhib Med Chem 26:688–95
- Rasheed A, Ashok Kumar CK. (2010). Tyrosine and glycine derivatives as potential prodrugs: design, synthesis, and pharmacological evaluation of amide derivatives of mefenamic acid. J Enzyme Inhib Med Chem 25:804–11
- Reynolds JEF. (1993). Martindale the extra pharmacopeia. 13th ed. London: The Pharmaceutical press
- Sahin NO, Arslan H. (2007). Inclusion complex of prednisolone with skimmed milk Part I: physicochemical characterization. Yakugaku Zasshi 127:1255–61
- Sammour OA, Hammad MA, Megrab NA, Zidan AS. (2006). Formulation and optimization of mouth dissolving tablets containing rofecoxib solid dispersion. AAPS Pharm Sci Tech 7:1–8
- Savita V, Piyush T, Subhash CC. (2008). Ketorolac-dextran conjugates: synthesis, in vitro and in vivo evaluation. Acta Pharm 57:441–50
- Savita V, Piyush T, Subhash CC. (2009). Dextran-etodolac conjugates: synthesis, in vitro and in vivo evaluation. Acta Pol Pharm 66:201–6
- Shrivastava PK, Singh R, Shrivastava SK. (2010). Dextran successful carrier molecule for the delivery of NSAIDs with reduced gastrointestinal effect. J Drug Del Sci Tech 20:135–42
- Simon LS, Lanza FL, Lipsky PE, et al. (1998). Preliminary study of the safety and efficacy of SC-58635, a novel cyclooxygenase 2 inhibitor: efficacy and safety in two placebo-controlled trials in osteoarthritis and rheumatoid arthritis, and studies of gastrointestinal and platelet effects. Arthritis Rheum 41:1591–602
- Skiba M, Morvan C, Duchene D, et al. (1995). Evaluation of gastrointestinal behavior in the rat of amphifilic β-cyclodextrin nanocapsules, loaded with indomethacin. Int J Pharm 126:275–9
- Topaloglu Y, Yener G, Groning R. (1999). Solid dispersion tenaxicam with skimmed milk via freeze-drying. Acta Pharm Turc 41:19–27
- Topaloglu Y, Yener G. (1999). Inclusion of ketoprofen with skimmed milk by freeze-drying. II Farmaco 54:648–52
- Topaloglu Y. (1981). The preparation and identification of indomethacin arginine and lysine salts. Acta Pharm Turc 23:10–15
- Topaloglu, Y, Yener, G, Toprak, N. (1997). Modulation of anti inflammatory drugs ulcerogenicity via solid dispersion with skimmed milk on the example of indomethacin. Acta Pharm Turc 39:167–70
- Urushidani T, Okabe S, Takeuchi K, Takagi K. (1977). Effects of various amino acids on indomethacin-induced gastric ulcers in rats. Jpn J Pharmacol 27:316–19
- Valleri M, Mura P, Maeshrelli F, et al. (2004). Development and evaluation of glyburide fast dissolving tablets using solid dispersion technique. Drug Dev Ind Pharm 30:525–34
- Vane JR. (1971). Inhibition of prostaglandin synthesis as a mechanism of action for aspirin like drugs. Nature 231:232–5
- Vane JR. (1998). Mechanism of nonsteroidal anti-inflammatory drugs. Am J Med 104:25–85
- Vijaya Kumar SG, Mishra DN. (2006). Preparation and evaluation of solid dispersion of meloxicam with skimmed milk. Yakugaku Jasshi 126:93–7
- Walstra P, Geurts TJ, Noomen A, et al. (1999). Dairy technology, principles of milk properties and processes. New York: Marcel Dekker
- Walstra P, Wouters JTM, Geurts TJ. (2006). Dairy science and technology. New York: Taylor & Francis, 341
- Walstra P. (1990). On the stability of casein micelles. J Dairy Sci 73:1965–79