Abstract
Gemcitabine-loaded solid lipid nanoparticles (SLNs) were produced by double emulsification technique using stearic acid as lipid, soy lecithin as surfactant and sodium taurocholate as cosurfactant. Prepared nanoparticles are characterized for particle size and surface morphology using scanning electron microscopy (SEM). Particle yield, entrapment efficiency and zeta potential were also determined. In-vitro release studies were performed in phosphate-buffered saline (PBS) pH 7.4 using metabolic shaker. The formulation F6 with maximum entrapment efficiency 72.42% and satisfactory in-vitro release was selected. In-vivo tissue distribution to liver, spleen, lung, heart and kidneys of optimized formulation followed by stability study under specific conditions were also determined. This investigation has shown preferential drug targeting to liver followed by spleen, lungs, kidneys and heart. Stability studies showed no significant change in the particle size followed with very slight decrease in entrapment efficiency at 25 ± 2 °C/60 ± 5% RH over a period of three months.
Introduction
Among various novel colloidal carriers present today, solid lipid nanoparticles (SLNs) have versatile potential for safe and nontoxic formulation of cytotoxic drugs, and achieves site-specific drug release. SLNs are made from solid lipids, which combines the advantages of other innovative carrier systems while at the same time minimizes the associated problems. These possible benefits include physical stability, protection of incorporated labile drugs from degradation, controlled release and excellent tolerability (Wissing et al., Citation2004). SLNs are particulate systems for parenteral drug administration with mean particle diameters ranging from 50 to 1000 nm and they are composed of wide variety of lipids including lipid acids, mono-, di-, or triglycerides, glyceride mixtures or waxes, and stabilized by the biocompatible surfactant(s) of choice (non-ionic or ionic). At room temperature, the particles are in the solid state, therefore the mobility of incorporated drugs is reduced, which is prerequisite for controlled drug release. They have potential to incorporate lipophilic or hydrophilic drugs or diagnostics (Muhlen et al., Citation1998; Utreja & Jain, Citation2002; Reddy et al., Citation2007). Intravenous studies show that liver is the major organ depot for nanoparticles followed by spleen for particles in the size range of 200–300 nm (Garnett & Kallinteri, Citation2006; De Jong et al., Citation2008; Sarlo et al., Citation2009).
Gemcitabine is a nucleoside analog used as a form of chemotherapy for non-small cell lung, pancreatic, bladder and breast cancer. It is being investigated for use in esophageal cancer and is used experimentally in lymphomas and various other tumor types. Gemcitabine represents an advance in pancreatic cancer care. The major limitation of gemcitabine is short half life and strong side effects when administered intravenously (Li et al., Citation2009; Jia et al., Citation2010). Short half lives are commonly due to fast renal clearance, decreased protein binding and enzymatic degradation (Werle & Bemkop Schnurch, Citation2006). Inherent drawbacks of parenteral gemcitabine administration warrant an alternative drug delivery system. SLNs offer great promise to improve therapeutic effectiveness and safety profile of the conventional form of cancer chemotherapy. Hence in the present work an attempt is being made to provide an alternative colloidal drug delivery system for gemcitabine in the form of SLNs.
Materials
Gemcitabine hydrochloride was purchased from Aarti industries limited (Mumbai, India). Stearic acid (biochemistry grade) and sodium taurocholate were purchased from Loba Chemie Pvt, Ltd. (Mumbai, India). Soy lecithin was purchased from Sigma Aldrich Chemicals Pvt. Ltd (Bangalore, India). All other chemicals used were of analytical reagent grade.
Methods
Preparation of gemcitabine-loaded SLNs
SLNs were prepared from a warm w/o/w emulsion containing stearic acid as lipid, soy lecithin as surfactant and sodium taurocholate as co-surfactant. Warm w/o/w multiple emulsion can be prepared in two steps. Firstly, w/o emulsion is prepared by adding an aqueous solution containing drug, to a mixture of melted lipid, surfactant and co-surfactant with mechanical stirring using a propeller stirrer (RQ 121 D, Remi Equipments Ltd., Mumbai, India) at 80 °C for 10–15 min to obtain a colloidal system. In the second step, the formed w/o emulsion is added to a mixture of water, surfactant and co-surfactant to obtain a colloidal w/o/w system. SLNs can be obtained by dispersing the warm multiple emulsion in cold aqueous medium in a fixed ratio, under mechanical stirring with propeller stirrer (RQ 121 D, Remi Equipments Ltd.). Factors such as rate of addition, distance of needle from the surface of liquid and rate of stirring were standardized to reduce particle size. In order to obtain optimum nanoemulsion, the needle was placed 4 cm from the surface of the water and mixture stirred at 3000 rpm. After the complete addition of multiple emulsion, the SLN dispersion was further stirred for 3 h. After stirring, the SLN dispersion was subjected to ultra sonication for a period of 10 min (Sarmento et al., Citation2007; Doijad et al., Citation2008; Gallarate et al., Citation2009; Jelvehgari et al., Citation2010).
Freeze-drying of nanoparticles
Usually, nanoparticles may subject to a series of stability problems such as aggregation, fusion and leakage of the encapsulated drug into the storage medium. One of the approaches to resolve this problem is to freeze dry the nanoparticles. The prepared nanoparticle formulations were freeze-dried using Heto freeze drier (UK) at −40 °C for 24 h. The temperature and vacuum conditions were optimized to recover completely dried SLNs. The vacuum was maintained at 0.06 mB. The obtained freeze-dried SLNs were stored at −4 °C (Cavalli et al., Citation1997; Konan et al., Citation2002; Abdelwahed et al., Citation2006).
Evaluation of gemcitabine loaded SLNs
Percentage yield
Percentage practical yield is calculated to know about percent yield or efficiency of any method, thus it helps in selection of appropriate method of production. Practical yield was calculated as the weight of dried SLNs recovered from each batch in relation to the sum of the starting material.
Particle size and surface morphology
Particle size analysis was done by scanning electron microscopy (SEM) (JEOL JSM-T330A, Tokyo, Japan). It has been used to determine particle size and surface morphology of nanoparticles. Approximately 2 mg of SLN was dispersed in de-ionized water. A few drops of this suspension were placed on a metal stub and allowed to air dry to form a thin uniform layer of particles on the metal stub. The stub was then coated with gold–palladium alloy (∼200 nm) under reduced pressure (0.133 Pa) for 5 min using an ion sputtering device (JFC-1100E, JEOL, Tokyo, Japan). Then the gold-coated SLN are observed under SEM and photographs were taken. Each sample was measured in triplicate.
Drug entrapment efficiency
The percentage of incorporated gemcitabine (loading efficiency) was determined with a UV-Visible spectrophotometer (UV-1201 Shimadzu, Japan) at 268 nm. The drug loaded in SLNs was calculated with the total amount of drug subtracting with that of free drug. The drug entrapment efficiency (EE%) and the drug loading (DL%) was determined in triplicate, calculated by the following equation,
Where Wlp = amount of drug loaded in lipid nanoparticles, Wtotal = total amount of gemcitabine, Wlipid = weight of lipid in SLNs.
Zeta potential
Zeta potential was measured using a zeta potentiometer (Malvern Nano ZS90, Malvern, UK). To determine zeta potential, SLN samples were diluted with double distilled water and placed in the electrophoretic cell in a cuvette. Each sample was analyzed in triplicate (Luo et al., Citation2006).
In-vitro drug release studies
SLNs equivalent to 10 mg of gemcitabine were weighed and transferred into a conical flask containing 50 ml of PBS pH 7.4. Then the flask was kept in a metabolic shaker and the shaker was adjusted to 50 horizontal shakes per minute at 37 ± 0.5 °C. One milliliter aliquot of release medium was withdrawn at time intervals of 1, 2, 3, 4, 6, 8, 10, 12 and 24 h and replaced by the same volume of PBS. These samples were filtered through 0.45 µm membrane filter. The filtrate was diluted suitably and estimated by UV-Visible spectrophotometer (UV-1201 Shimadzu, Japan) at 268 nm (Doijad et al., Citation2008).
In-vivo drug targeting studies
This study was carried out after obtaining the due permission for conduction of experiments from relevant ethics committee (KLEU College of Pharmacy, Belgaum) which is registered for “Teaching and Research on Animals” by committee for the purpose of control and supervision of experiments on animal, Chennai (Registration number 221/CPCSEA).
This study was carried out to compare the targeting efficiency of gemcitabine-loaded SLNs with that of free drug in terms of percentage increase in targeting various organs of reticuloendothelial system (RES) like pancreas, liver, lungs, spleen and kidneys. Experiments were performed on Wistar rats of 200–250 g weight. All the experiments were carried out in accordance with the protocols approved by the institutional animal ethics committee (KLEU College, Belgaum, India).
Nine healthy adult rats weighing 200–250 g were selected, a constant day and night cycle was maintained and they were fasted for 12 h. The animals were divided into three groups, each containing three rats. Group I received SLNs equivalent to 673.8 µg of gemcitabine intravenously in the tail vein after re-dispersing them in sterile PBS solution (Sacchi et al., Citation2006). SLNs from F6 batch were selected for the study. Group II rats received 673.8 µg of pure gemcitabine intravenously. Group III rats were treated as solvent control and were injected intravenously with sterile PBS solution.
After 24 h, the rats were sacrificed and their pancreas, liver, lungs, spleen and kidneys were isolated. The individual organs of each rat were homogenized separately by using a tissue homogenizer (Remi Motors Ltd, Mumbai, India) and the homogenate was centrifuged at 15000 rpm for 30 min. The supernatant was collected and filtered through 0.45 µm filter (Millipore Molsheim, France) and analyzed after dilution with PBS as per the previously reported high performance liquid chromatography (HPLC) method (Hirn et al., Citation2011).
Stability studies
The optimized formulation of gemcitabine-loaded SLNs was tested for their stability. Freshly prepared freeze-dried three samples of optimized SLNs are sealed in vials and were placed in a stability chamber maintained at 25 ± 2 °C/60 ± 5% RH. With a sampling frequency of one month, sealed SLNs in stability chamber were analyzed over three months for particle size and entrapment efficiency (The European Agency for the Evaluation of Medicinal Products, Citation2003).
Statistical analysis
Significance of differences was evaluated using the Student’s t-test at the probability level of 0.05. All the values are reported as mean ± standard error of mean (SEM) of three determinations.
Results
The drug–lipid interactions are assessed by running Fourier transform infrared spectroscopy (FTIR) and differential scanning calorimetry (DSC) for the pure gemcitabine, stearic acid, and for the physical mixture of gemcitabine and stearic acid, and for gemcitabine nanoparticles. All the characteristic FTIR peaks of drug and stearic acid were present in the combination spectra indicating compatibility of the drug with the polymer used. DSC thermogram of pure drug showed a sharp melting peak at 266.5 °C, whereas stearic acid exhibited melting peak at 56.4 °C. No significant change in the position of endothermic peaks was observed between physical mixture drug and stearic acid. Percentage yield of formulations F1 to F6 by varying drug to lipid ratio was determined and is presented in . During the study, other formulation variables such as amount of surfactant, volume of continuous phase and the processing variables such as stirring speed and stirring time were maintained constant. Particle size and surface morphology of all the formulations were determined under SEM (JEOL JSM-T330A). Average particle size of gemcitabine-loaded SLNs of formulations F1 to F6 was found to be 127, 145, 173, 199, 238 and 263 nm, respectively. Scanning electron photomicrograph of formulation F6 is shown in .
Table 1. Drug entrapment efficiency and zeta potential of gemcitabine-loaded SLNs.
The amount of drug bound per 10 mg of dried lipid nanoparticles was determined. shows the results of the drug entrapment efficiency and zeta potential in each of these formulations. The maximum entrapment efficiency was found to be 72.42 ± 1.13% in F6 and minimum entrapment efficiency was found to be 52.86 ± 0.90% in F1. Therefore, the formulation having the highest encapsulation parameters was selected for in-vivo tissue distribution and stability study. The stability of the formulation was evaluated by measuring the zeta potential of the particles by zeta potentiometer. Zeta potential of all formulated nanoparticles was in the range of −17.4 ± 0.85 to −21.9 ± 0.41 mV. The results are shown in .
All the six formulations of gemcitabine-loaded SLNs were subjected to in-vitro release studies. These studies were carried out using metabolic shaker in pH 7.4 PBS. The cumulative percent drug release of formulations from F1 to F6 after 24 h was 93.53%, 86.09%, 84.08%, 82.19%, 78.48% and 71.95%, respectively. The release data obtained for formulations F1, F2, F3, F4, F5 and F6 are showed in . Formulation F6 with highest entrapment efficiency and satisfactory in-vitro release was selected for in-vivo drug targeting studies. The amount of drug targeted from SLNs and free drug in various organs is presented in . The average targeting efficiency of drug-loaded SLN was found to be 23.23 ± 0.36% of the injected dose in liver, 12.62 ± 0.44% in spleen, 9.68 ± 0.12% in lungs, 3.58 ± 0.20% in kidneys and 7.14 ± 0.26% in heart, whereas accumulation of pure drug was 12.01 ± 0.27% in liver, 8.01 ± 0.14% in spleen, 6.31 ± 0.42% in lungs, 2.36 ± 0.32% in kidneys and 5.77 ± 0.19% in heart of the injected dose. Results of stability study of the selected formulation F6 for two parameters namely particle size and entrapment efficiency is shown in .
Figure 2. In-vitro release profile for different formulations of gemcitabine-loaded SLNs formulations F1 to F6.
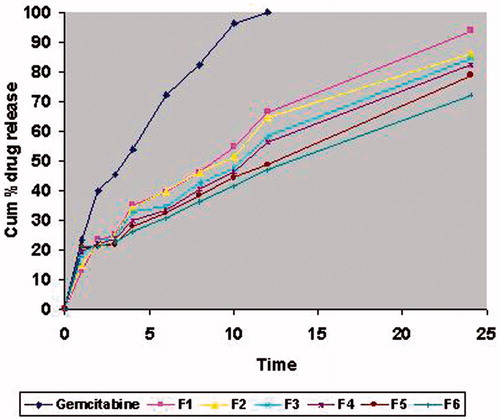
Table 2. In-vivo targeting studies of gemcitabine-loaded SLNs for formulation F6.
Table 3. Stability study of freeze-dried formulation F6 for particle size and entrapment efficiency assessment over three months.
Discussion
The aim of this study was to prepare gemcitabine-loaded SLNs and to investigate their physical characteristics and tissue distribution efficiency. From the results of FTIR and DSC, physical incompatibility between the components was discarded. The particle size analysis shows that increase in concentration of lipid resulted in the increase of particle size. SEM study revealed that the particles were of spherical shape with smooth and nonporous surface. The increase of particle size was followed by increase in drug pay load and entrapment efficiency of gemcitabine in lipid nanoparticles. This may be due to the increased lipid concentration in the lipid formulation. Negative charge of zeta potential values indicates that they are moderately stable, which may be attributed by the presence of surfactant soy lecithin.
The in-vitro release data show cumulative percent drug released as a function of time for different formulations of gemcitabine-loaded SLNs in . The in-vitro release of all the six batches of SLNs showed an interesting bi-phasic release with an initial burst effect. In the first hour, drug released was 12.76%, 14.72%, 17.8%, 19.42%, 20.79% and 21.18% for F1, F2, F3, F4, F5 and F6, respectively. Afterwards, the drug release followed a steady pattern. The burst release in the first hour can be attributed to the drug loaded on the surface of SLNs. The same pattern of release is reported for curcumin (Tsai et al., Citation2011). The values of in-vitro release were attempted to fit into various mathematical models like zero order, first order, Higuchi matrix, Peppas and Hixson Crowell. Models with the highest correlation coefficient were judged to be the most appropriate model for the dissolution data. For formulations F2, F3 and F5 the best fitting linear parameter was that of Higuchi matrix with the ‘n’ value of 0.4269, 0.4348 and 0.4521, respectively. This indicates that the drug release is controlled by diffusion of the drug through the pores. On the other hand, formulations F1, F4 and F6 best fitted into the Peppas model with the ‘n’ value of 0.5645, 0.5720 and 0.593, respectively.
Therefore, the formulation with high encapsulation efficiency and satisfactory in-vitro release was selected for further studies. The results of in-vivo tissue distribution revealed that higher concentration of drug was targeted to the RES organs after administering the dose in the form of SLNs when compared with pure drug. The average targeting efficiency of gemicitabine-loaded SLNs was significantly (p < 0.05) higher compared with the pure drug. The selected F6 formulation with mean particle size of 263 nm showed preferential drug targeting to liver followed by spleen, lungs, heart and kidneys. This is attributed by the higher phagocytotic rates for the uptake of nanoparticles in liver and spleen, than cells of other tissues. A similar phenomenon was observed in the selective targeting of albumin nanospheres (Li et al., Citation2009). The results of stability testing revealed that there was no significant change in the mean particle size and there was a very slight decrease in the entrapment efficiency (%) which may be due to the expulsion of drug from lipid matrix during storage.
Conclusion
Gemcitabine-loaded SLNs prepared by double emulsification technique showed controlled drug release when compared to free drug. In-vivo tissue distribution study of the optimized solid lipid formulation showed increase in cellular uptake by various organs over free drug. The poor entrapment efficiency may nevertheless be pointed as a major drawback in SLN formulation. Therefore, these lipid nanoparticles can be used as colloidal carrier for improving cellular uptake of drug molecules and controlled release.
Acknowledgements
The authors sincerely thank the Hindalco Ind. Ltd, R&D centre, Belgaum for providing the facility of SEM.
Declaration of interest
The authors report no declaration of interest.
References
- Abdelwahed W, Degobert G, Stainmesse S, Fessi H. (2006). Freeze drying of nanoparticles: formulation, process and storage considerations. Adv Drug Deliv Rev 58:1688–713
- Cavalli R, Caputo O, Carlotti ME, et al. (1997). Sterilization and freeze drying of drug free and drug loaded solid lipid nanoparticles. Int J Pharm 148:47–54
- De Jong WH, Hagens WI, Krystek P, et al. (2008). Particle size-dependent organ distribution of gold nanoparticles after intravenous administration. Biomaterials 29:1912–9
- Doijad RC, Manvi FV, Godwani DM, et al. (2008). Formulation and targeting efficiency of cisplatin engineered solid lipid nanoparticles. Indian J Pharm Sci 70:203–7
- Gallarate M, Trotta M, Battaglia L, Chirio D. (2009). Preparation of solid lipid nanoparticles from W/O/W emulsions: preliminary studies on insulin encapsulation. J Microencapsul 26:394–402
- Garnett MC, Kallinteri P. (2006). Nanomedicines and nanotoxicology: some physiological principles. Occup Med 56:307–11
- Hirn S, Behnke MS, Schleh C, et al. (2011). Particle size dependent and surface charge dependent biodistribution of gold nanoparticles after intravenous administration. Eur J Pharm Biopharm 77:407–16
- Jia L, Zheng JJ, Jiang SM, Huang KH. (2010). Preparation, physicochemical characterization and cytotoxicity in vitro of gemcitabine loaded PEG-PDLLA nanovesicles. World J Gastroenterol 16:1008–13
- Jelvehgari M, Barar J, Valizadeh H, Heidari N. (2010). Preparation and evaluation of poly (ε-caprolactone) nanoparticles-in-microparticles by W/O/W emulsion method. Iran J Basic Med Sci 13:85–96
- Konan YN, Gurny R, Allemann E. (2002). Preparation and characterization of sterile and freeze-dried sub-200nm nanoparticles. Int J Pharm 233:239–52
- Li JM, Chen W, Wang H, et al. (2009). Preparation of albumin nanospheres loaded with gemcitabine and their cytotoxicity against BXPC-3 cells in vitro. Acta Pharmacol Sin 30:1337–43
- Luo YF, Chen DW, Ren LX, et al. (2006). Solid lipid nanoparticles for enhancing vinpocetine's oral bioavailability. J Control Release 114:53–9
- Muhlen AZ, Schwarz C, Mehnert W. (1998). Solid lipid nanoparticles (SLN) for controlled drug delivery – drug release and release mechanism. Eur J Biopharm 45:149–55
- Reddy LH, Dubernet C, Mouelhi SL, et al. (2007). A new nanomedicine of gemcitabine displays enhanced anticancer activity in sensitive and resistant leukemia types. J Control Release 124:20–7
- Sacchi A, Gasparri A, Gallo Stampino C, et al. (2006). Synergistic antitumor activity of cisplatin, paclitaxel and gemcitabine with tumor vasculature-targeted tumor necrosis factor-α. Clin Cancer Res 12:175–82
- Sarlo K, Blackburn KL, Clark ED, et al. (2009). Tissue distribution of 20 nm, 100 nm and 1000nm fluorescent polystyrene latex nanospheres following acute systemic or acute and repeat airway exposure in the rat. Toxicology 263:117–26
- Sarmento B, Martins S, Ferreira D, Souto EB. (2007). Oral insulin delivery by means of solid lipid nanoparticles. Int J Nanomed 2:743–9
- The European Agency for the Evaluation of Medicinal Products. (2003). Stability testing guidelines: stability testing of new drug substances and products. London: ICH-Technical Coordination, EMEA. Available from: http://www.emea.eu.int [last accessed 20 Feb 2003]
- Tsai YM, Chien CF, Lina LC, Tsai TH. (2011). Curcumin and its nano formulation: the kinetics of tissue distribution and blood brain barrier penetration. Int J Pharm 416:331–8
- Utreja S, Jain NK. (2002). Solid lipid nanoparticles: controlled and novel drug delivery. 1st ed. : CBS Published and Distributors, 408–25
- Werle M, Bemkop Schnurch A. (2006). Strategies to improve plasma half life time of peptide and protein drugs. Amino Acids 30:351–67
- Wissing SA, Kayser O, Muller RH. (2004). Solid lipid nanoparticles for parenteral drug delivery. Adv Drug Deliv Rev 56:1257–72