Abstract
Lung cancer is a dreadful disease which claims to be more life threatening as compared to total sum up of colon, prostate and breast cancers. Thus, there is an urgent need to develop an effective delivery approach for its management. Paclitaxel (PTX) is one of the well-known choice as antineoplasitic agent used for the treatment of different types of human cancers such as non-small-cell lung, head and neck cancers, leukemia, breast, ovarian and melanoma. Lactoferrin (Lf), a “multifunctional protein” is crucial for natural immunity which is secreted by exocrine glands. Lf receptors are expressed on the apical surface on bronchial epithelial cells. These over-expressed LF receptors can be utilized for the transportation of Lf-conjugated drug or nanocarrier devices. The present study was aimed to develop PTX-loaded Lf-coupled solid lipid nanoparticles (SLNs) for the treatment of lung cancer. PTX-loaded SLNs were prepared, characterized and then coupled with Lf using carbodiimide chemistry. The formulations were characterized by transmission electron microscopy, particle size, polydispersity index and zeta potential, whereas Lf conjugation was confirmed by FT-IR and 1H NMR and efficiency of prepared system was evaluated by in vitro, ex vivo and in vivo evaluations. The ex vivo cytotoxicity studies on human bronchial epithelial cell lines, BEAS-2B, revealed superior anticancer activity of Lf-coupled SLNs than plain SLNs and free PTX. In vivo biodistribution studies showed higher concentrations of PTX accumulated in lungs via Lf-coupled SLNs than plain SLNs and free PTX. These studies suggested that Lf-coupled PTX-loaded SLNs could be used as potential targeting carrier for delivering anticancer drug to the lungs with the minimal side effects.
Introduction
Lung cancer is a dreadful disease which claims more lives each year than colon, prostate, lymph and breast cancers combined. Tobacco smoking is considered to be the main cause which kills more than 1.4 million lung cancer deaths that occur annually worldwide as reported by World Health Organization, 2012. The main types of lung cancer are non-small-cell lung cancer (NSCLC), which accounts for about 80% of cases, and small-cell lung cancer (SCLC), which accounts for the other 20%. Nearly 70% of NSCLC patients present with advanced disease stage i.e. III/IV (Provencio et al., Citation2011; Gregory, Citation2012). Lung cancer cell has the property of metastasis and thus they can travel to another area or organ in the body leading to cancer (Akunuru et al., Citation2012). The occurrence of lung cancer in non-smokers, who accounts for as many as 15% of cases (Salim et al., Citation2011) is often attributed to a combination of different factors such as genetic factors, asbestos, air pollution, etc. includes secondhand smoke.
In the midst of the various antineoplastic agent used in cancer chemotherapy, Paclitaxel (PTX) is one of the drug/agent, a complex diterpene taxane, obtained from the bark of the Western yew tree, Taxus brevifolia (Zhao et al., Citation2011). It is a well-known choice as antineoplasitc agent is used for the treatment of different types of human cancers such as non-small-cell lung, head and neck cancers, leukemia, breast, ovarian and melanoma (Surapaneni et al., Citation2012; Xu & Veenstra, Citation2012), and in present research work, PTX is a choice of drug.
Lactoferrin (Lf), a 80-kDa iron-binding glycoprotein, is present in several biological fluids and in the secretory granules of neutrophils (Liao et al., Citation2010; Rana et al., Citation2012), as well as it is associated with a wide variety of biologically important processes, including host defense, regulation of cell growth, and cell differentiation (Fujita et al., Citation2004; Liao et al., Citation2011). Lf, a “multifunctional protein” is crucial for natural immunity which is secreted by exocrine glands. It is a form of iron-binding glycoprotein that has known to show antioxidant, antimicrobial and antineoplastic activity. It has the inherent capability to provoke apoptosis and hinder with cancer cell proliferation. Recent literature shows that Lf stimulate apoptosis and hinders with proliferation of cancer cells. It also promotes restoration of leucocytes and erythrocytes after chemotherapy (Gibbons et al., Citation2011). The biological properties of Lf are mediated by specific receptors on the surface of target cells. Lf receptors are expressed on the apical surface on bronchial epithelial cells. These expressed Lf receptors can be utilized for the transportation of Lf-conjugated drug or nanocarrier devices. By this way, Lf conjugation potentiate the anticancerous activity of PTX bearing solid lipid nanoparticles (SLNs)’s system via specific delivery to lungs.
SLNs function as an alternative drug carrier system to other novel delivery approaches such as emulsions, liposomes, and polymeric nanoparticles. SLNs offer several advantages conferred by their colloidal dimensions including: easy incorporation of both lipophilic and hydrophilic drugs; improved biocompatibility; potential for site-specific drug delivery; improved drug and formulation stability; ability to freeze dry and reconstitute; high drug payload with controllable particle size; large surface area with small particle size, effective drug loading and enhanced surface interaction with cell membrane; avoidance of carrier toxicity; low production cost; etc. (Cavalli et al., Citation1993; Mukherjee et al., Citation2009).
The drug delivery meadow has witnessed the emerging potential of SLNs toward targeted delivery of anticancer agents by reducing their RES uptake. An anchoring legend to the surface of SLNs further specify the delivery of bioactives. Therefore, the current work was aimed to design and evaluate the efficacy of Lf-anchored PTX SLNs for effective management of lung cancer. The present study reveals the targeting potential of Lf-coupled SLNs for site-specific delivery of PTX to the lungs.
Material and methods
Material
PTX was obtained as a gift sample from Dabur India Limited, Ghaziabad, India. Lf was kindly provided by Prof. T.P. Singh, AIIMS, New Delhi, India. Hydrogenated soya phosphatidylcholine (HSPC) was purchased from Pall Germany Sciences (Washington, NY, USA). All other reagents and solvents were of analytical grade. Deionised water was used throughout the study.
Animals
The in vivo study was conducted on albino rats of Sprague-Dawley strain. The animal studies were conducted with the permission of the Institutional Animal Ethical Committee of Dr. Hari Singh Gour University, Sagar, Madhya Pradesh, India.
Sample preparation for biodistribution study
One gram of each organ was homogenized with 2.0 ml of phosphate-buffered saline (PBS) (pH 7.4) by glass homogenizer. In the organs weighing <1 g, whole organ was used. To tissue homogenate, 2 ml of acetonitrile was added and kept for 30 min. The resulting suspension was centrifuged for 20 min at 5000 rpm and was filtered through 0.22-µm membrane filter. Tissue homogenate was analyzed for drug content. Similarly, blood was collected from cardiac puncture in a centrifuge tube containing heparin sodium (anticoagulant) then centrifuged at 5000 rpm for 10 min. Supernatant was collected and acetonitrile was added to precipitate the proteins and filter by centrifugation at 5000 rpm for 10 min and supernatant was collected. One ml of collected supernatant was filtered through 0.22-µm membrane filter and drug concentration in serum at different time interval was determined using high-performance liquid chromatography (HPLC) method.
Preparation of plain SLN
The SLNs were prepared by the solvent injection method as reported by Hu et al. (Citation2008) with necessary modifications. Briefly, tristearin and HSPC in the ratio 60:40, SA (1% w/w) and PTX (10 mg) were taken, separately. All the components were dissolved in a minimum quantity of absolute alcohol and heated ∼70 °C in a beaker. Tween-80 (0.5% w/v) was dissolved in double-distilled water (DDW) and heated at ∼70 °C. Then the organic phase i.e. alcoholic solution containing lipid mixture, SA and drug was added to preheated aqueous solution at the same temperature (∼70 °C) with constant mechanical stirring (Remi Instruments, Mumbai, India) at 3000 rpm for 1 h. The preformed lipid suspension was then sonicated for 2 min by using a probe sonicator (Trans-O-Sonic (Mumbai, Maharashtra, India) to form SLNs. The obtained dispersion was filtered through cellulose acetate filter (0.45 μm) to remove any excess lipid (Hu et al., Citation2008).
Preparation of Lf-coupled SLN
Conjugation of Lf with SLNs was done via carbodiimide chemistry, i.e. coupling of carboxylic group of Lf with the amine group of the stearylamine present on the surface of previously formed drug-loaded SLNs in the presence of 1-ethyl-3-(3-dimethylaminopropyl)carbodiimide (EDC). For the preparation of coupled SLNs, Lf was dissolved in a minimum quantity of PBS (pH 7.4) and dispersed in 1.0 ml of drug-loaded SLNs. EDC (10 mg) was then added to the above mixture and incubate for 6 h with stirring, to ensure the completion of the reaction at room temperature (Figure 1). Excess unbound Lf was removed by passing the formulation through Sephadex G-50 mini-column. The preparation was subjected to lyophillization (Heto Drywinner, DW3, Heto-Halter, Allerod, Denmark) (Jain et al., Citation2010).
Characterization of nanoparticles
FT-IR and 1H NMR spectroscopy of Lf-conjugated nanoparticles
The Lf-conjugated nanoparticles were characterized by FT-IR (Shimadzu 8201 PC, Kyoto, Japan), and 1H NMR spectroscopy for the confirmation of conjugation. 1H NMR spectroscopy was carried out using D2O and DMSO as a solvent (Bruker Advanced DRX, 400 MHz, Bruker Optics, Tokyo, Japan).
Morphology
Transmission electron microscopy (TEM; Philips Morgagni 268, Eindhoven, The Netherlands) was used as a visualizing aid for particle morphology. The sample (10 µL) was placed on the grids and allowed to stand at room temperature for 90 s. Excess fluid was removed by touching the edge with filter paper. All samples were examined under a TEM at an acceleration voltage of 100 KV, and photomicrographs were taken at a suitable magnification (40 000×).
Particle size and distribution
The average particle size and size distribution of the SLNs were determined by photon correlation spectroscopy using a Malvern Zetasizer DTS ver. 4.10 (Malvern Instruments, Malvern, UK). The samples of SLNs dispersions were diluted in 1:9 v/v with deionized water. The particles size and size distribution were represented by an average (diameter) of the Gaussian distribution function in the logarithmic axis mode.
Zeta potential
The zeta potential of SLNs was measured by laser-based multiple angle particle electrophoresis analyzer, Malvern Zetasizer DTS ver. 4.10 (Malvern Instruments, Malvern, UK). Both plain SLNs and Lf-coupled SLNs were dispersed in deionized water, placed in the electrophoretic cell with an electric field of 15.24 V/cm and the zeta potential was determined.
Entrapment efficiency
Drug entrapment of the PTX in SLNs was determined by dispersing the 10-mg PTX-loaded SLNs in DDW in cellulose dialysis bag (MWCO 1000 Da; Sigma-Aldrich, Buchs, Switzerland), then dialyzed with help of magnetic stirring (50 rpm; Remi Instruments, Mumbai, India) against PBS (pH 7.4) having 0.1% Tween-80 (Yong et al., Citation2012) under sink condition for 10 min to remove any unentrapped drug from the formulation. One milliliter aliquot was withdrawn after 10 min and diluted 10 times in a volumetric flask with 30:70 methanol:PBS (pH 7.4). Absorbance was measured spectrophotometrically (Schimadzu, 1601, Kyoto, Japan) at 230 nm to indirectly estimate the amount of drug entrapped within the system.
In vitro drug releases
The in vitro drug release studies of SLNs and Lf-conjugated SLNs were performed in PBS (pH 7.4; 0.1% w/v Tween-80) (Yong et al., Citation2012) using presoaked dialysis bag diffusion techniques with 0.4% albumin (pH 7.4) containing 0.1% w/v Tween-80. The dialysis bag retains SLNs and allows the free drug into the dissolution media. One milliliter of pure SLNs suspension free of any unentrapped drug was taken in a dialysis bag (MWCO 12 kDa, HiMedia Laboratories Pvt Ltd, Maharashtra, India) tied at both ends and placed in a beaker containing 50 ml of PBS (pH 7.4).
The beaker was placed over a magnetic stirrer (Remi Instruments, Mumbai, India) and temperature were maintained at 37 ± 1 °C throughout the study. The samples were withdrawn at definite time intervals and replaced with the same volume of PBS (pH 7.4). The withdrawn samples were analyzed for drug content by HPLC as reported by Wang et al. (Citation2003). Amount of PTX was assessed using ODS C18 column (National Analytical Corporation, Mumbai, Maharashtra, India) and mobile phase consisting of acetonitrile, methanol and ammonium acetate buffer (10 mM, pH 5.0) (48.5:16.5:35). Flow rate of instruments was 0.8 ml/min. The effluents peaks were measured at 230 nm with retention times of 8.5 min for PTX (Schimadzu, C18, Japan).
Cell cytotoxicity study
Human bronchial epithelial cell lines, BEAS-2B, were procured from the National Center for Cell Science (NCCS), Pune, India. The in vitro cytotoxicity was determined by sulforhodamine blue (SRB) colorimetric assay method (Dalai et al., Citation2012). The cell lines were grown in RPMI 1640 medium containing 10% fetal bovine serum and 2 mM l-glutamine. For the present screening experiment, cells were inoculated into 96-well microtiter plates in 100 µL per plate, depending on the doubling time of individual cell lines. After cell inoculation, the microtiter plates were incubated at 37 °C, 5% CO2, 95% air and 100% relative humidity for 24 h prior to addition of experimental drugs.
After 24 h, one 96-well plate containing 5 × 103 cells/well was fixed in situ with trichloro acetic acid. Experimental drugs were initially solubilized in dimethyl sulfoxide at 100 mg/ml and diluted to 1 mg/ml using water and stored frozen prior to use. At the time of drug addition, an aliquot of frozen concentrate (1 mg/ml) was thawed and diluted to prepare different sub-aliquots having drug concentration 100, 200, 400 and 800 μg/ml. Ten microliter aliquots of different drug dilutions having a final concentration of 10, 20, 40 and 80 μg/ml as mentioned above were added to the appropriate microtiter wells already containing 90 µl of the medium. The formulations were incubated with the cells for 24 h and the viability of cells was checked after an incubation period by counting the live cells using Neubauer chamber (Dalai et al., Citation2012). The results of % control growth and formulation concentration required for the GI50 on BEAS-2B are shown in and .
In vivo blood level and biodistribution studies
Albino rats were taken for the present study, weighing between 100 and 125 g, 6–8 weeks old and divided into three groups with 12 rats in each group. All 48 animals were fasted overnight and allowed access to water. The dose administered to rat was 25 mg/kg body weight. The surgical procedures were followed as described elsewhere (Kurmi et al., Citation2011). Plain PTX, PTX-loaded SLNs and PTX-loaded Lf-coupled SLNs formulations were administered to the each rat of first, second and third group, respectively through the tail vein.
One rat from each group was sequentially sacrificed and blood was collected by cardiac puncture. Different organs i.e. liver, spleen, lungs and kidney were excised, isolated and dried with tissue paper and weighed. Further, samples were prepared following the method mention under section material and method. The amount of drug present in each organ was quantified by HPLC method as reported by Wang et al. (Citation2003). Experiments were performed in triplicate.
Statistical analysis
In order to evaluate the extent of a relationship between two data sets, Pearson’s correlation coefficients were used. To analyze statistical differences among groups one-way analysis of variance was used. A p value of 0.05 was considered statistically significant.
Results and discussion
Formulation and ligand coupling of SLNs
SLNs were prepared by the solvent injection method using tristearin, HSPC, PTX and Tween-80. Staerylamine was added to provide the amine group on the surface of the SLNs. The conjugation of Lf with SLNs was performed via carbodiimide chemistry wherein carboxylate group of Lf was conjugated with amino group present on SLNs, with the help of EDC as coupling agent (Figure 1). Structure of the prepared conjugated system was confirmed by FT-IR and NMR. Result of FT-IR showed a strong peak at 3392.1 cm−1 suggested the free terminal amine groups of stearylamine present on the surface of plain SLNs. The FT-IR analysis of Lf-conjugated SLNs showed a characteristic peak of a C=O stretch of the amide linkage (–CONH) at 1698.0 cm−1 indicates the formation of conjugate via amide linkage. Further configuration was confirmed by 1H NMR spectroscopy, wherein shift at 2.3 ppm depicted the presence of NH2 groups present on the surface of plain SLNs. 1H NMR spectroscopy of the conjugated system showed a shift at 7.6 ppm which was due to the presence of –CONH linkage in conjugated SLNs.
Zeta potential
The zeta potential of PTX-loaded SLNs was found to be approximately two times more (6.8 ± 0.2 mV) as compared to PTX-loaded Lf-coupled SLNs (3.7 ± 0.3 mV). This may be due to the presence of positively charged amine groups over the surface of SLNs ().
Table 1. Comparison of particle size, polydispersity index, zeta potential and % drug entrapped.
Particle size and polydispersity index
The average particle size was studied by photon correlation spectroscopy. It was found that Lf-coupled SLNs having the larger particle size (249.4 ± 2.6 nm) than plain SLNs (201.1 ± 3.7 nm). This change in size may be due to Lf coupling over preformed SLNs. Polydispersity index of SLNs was found to be 0.234 and 0.283 for plain SLNs and Lf-coupled SLNs, respectively ().
Surface morphology
The shape of the both PTX-loaded and PTX-loaded Lf-coupled SLNs was found to be spherical as evidenced by the TEM photomicrographs. The photomicrographs depicted the nanometric size of both formulations. Increase in size in case of PTX-loaded Lf-coupled formulations was observed which may be due to the conjugation of Lf with SLNs ().
Drug entrapment efficiency
The drug entrapment efficiency of PTX-loaded SLNs was found to be 79.3 ± 0.5% whereas in case of PTX-loaded Lf-coupled SLNs, it was found to be 71.7 ± 0.5%. The decrease in % entrapment efficiency may be due to leaching of the drug during the incubation process at the time of Lf coupling with SLNs ().
In vitro drug release
The in vitro drug release studies depicted a biphasic drug release pattern which is an initial fast release followed by a slower sustained release. About, 34.32 ± 1.51 and 30.43 ± 2.37% drug was released in 8 h from PTX-loaded and PTX-loaded Lf-coupled SLNs, respectively. After 48 h release rate found to be of 79.94 ± 1.88 and 68.78 ± 2.68% for the PTX-loaded and PTX-loaded Lf-coupled SLNs, respectively (). The initial fast release may be due to release of surface absorbed drug on SLNs and the slower and sustained release may be due to the passing of the drug through the lipid matrix.
Cytotoxicity studies
The cell cytotoxicity screening of the free drug solution, PTX-loaded SLNs and PTX-loaded Lf-coupled SLNs were performed by SRB colorimetric assay. It was observed that PTX-loaded Lf-coupled SLNs exhibited significantly higher cytotoxicity in comparison to PTX-loaded SLNs. A further PTX solution was found to be less cytotoxic. PTX-loaded Lf-coupled SLNs were most cytotoxic and exhibited greater cellular uptake which may be triggered by ligand–receptor interaction. Higher uptake of PTX-loaded SLNs (plain and conjugated both) than the free drug within the cells might be responsible for the enhanced cytotoxic effect. The order of their cytotoxicity effect on BEAS-2B cells was found to be: PTX-loaded Lf-coupled SLNs > PTX-loaded SLNs > free PTX.
A relationship between % control growth and drug concentration in the concentration range of 10–80 μg/ml was determined. The % control growth at 10 μg/ml was found to be 28.1 ± 1.6% for free PTX, 22.9 ± 1.3% for PTX-loaded plain SLNs and 10.2 ± 1.1% for PTX-loaded Lf-conjugated SLNs, which decreases as the concentration increases and found to be 9.7 ± 0.7% for free PTX, 7.4 ± 0.5% for PTX-loaded plain SLNs and 0.7 ± 0.1% for PTX-loaded Lf-conjugated SLNs at 80 μg/ml (). The results showed that the formulations were less toxic at 10 μg/mL and had more cytotoxicity at 80 μg/mL. The IC50 value was found to be less for Lf-conjugated SLNs than free PTX and plain SLNs () which might be due the higher cellular uptake of the Lf-conjugated SLNs compare to free PTX and plain SLNs.
Table 2. Percentage control growth on BEAS-2B.
Table 3. Different formulation concentration required for the IC50 on BEAS-2B.
Biodistribution studies
The blood level studies of PTX-loaded Lf-coupled SLNs, PTX-loaded SLNs and PTX (free drug) were performed and the concentration of drug in blood samples was determined at various time intervals (). Maximum concentration of PTX in plasma was found to be 52.5 ± 0.6% after 1 h and then rapidly decreased to 5.8 ± 0.5% after 24 h. This may be due to the simultaneous tissue distribution of drug in various organs and rapid excretion of drug from kidney. However, the concentration of drug in plasma after administration of different type of SLNs (PTX loaded and PTX-loaded Lf coupled) was considerably low. This may possibly be due the PTX-loaded SLNs as well as PTX-loaded Lf-coupled SLNs can reach the blood with its intact form and then release PTX. In case of uncoupled formulation, the maximum percent dose recovered in serum was found to be 10.4 ± 0.8% after 1 h, which decreased to 5.4 ± 0.8% after 24 h, while in case of coupled formulation the maximum percent dose recovered in serum was found to be 8.4 ± 0.7% after 1 h, which was decreased to 4.1 ± 0.2% after 24 h (). The significant reduction in drug concentration in plasma may be accounted due to the fact that most of the drug present in blood was entrapped in SLNs which lead to reduced toxicity of PTX.
The biodistribution studies in liver, kidney, spleen and lung after intravenous administration of free PTX at different time interval shows that the maximum accumulation of the drug in these organs was achieved within a few hour. The maximum amount of drug accumulated in different organs following intravenous administration of free drug were found to be 25.8 ± 0.6% in the liver, 13.9 ± 0.4% in kidney, 9.9 ± 0.9% in lung and 6.2 ± 0.7% in spleen after 6 h whereas, after 24 h, the amount of drug recovered was 10.4 ± 0.2% in the liver, 3.1 ± 0.6% in kidney, 2.4 ± 0.1% in lung and 1.1 ± 0.2% in the spleen, which might be due to clearance of the drug from the body ().
The tissue i.e. liver, lung, kidney and spleen distribution studies of uncoupled and Lf-coupled SLNs were shown in . In case of uncoupled formulation, the liver uptake was found to be 17.4 ± 0.6% after 6 h, while it was 4.7 ± 0.8% after 24 h. In case of the spleen, the percent drug recovered was found to be 5.7 ± 0.8% in 6 h and not detected in 24 h. However, in the case of Lf-coupled formulation, the percent drug recovered by the liver and spleen was found to be 3.2 ± 0.2% and not detected, respectively, in 24 h. The decrease in percent drug recovered may be due to the less RES uptake of the drug from the liver and spleen.
Figure 5. Distribution profile of free PTX, PTX-loaded SLNs and PTX-loaded Lf-coupled SLNs after IV administration in (a) liver, (b) kidney, (c) lungs and (d) spleen. n = 5; *p < 0.05; **p < 0.01.
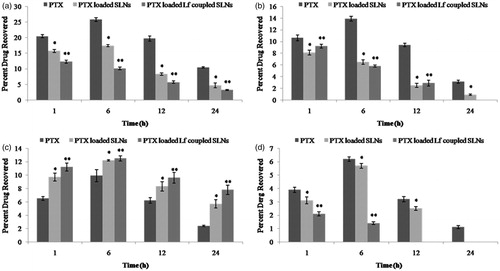
The percent drug recovered from the lung was found to be 12.2 ± 0.1% in 6 h and 5.7 ± 0.6% in 24 h in case of uncoupled formulation, while with Lf-coupled formulation the percent drug recovered was found to be 20.5 ± 0.4 and 10.8 ± 0.7% after 6 and 24 h, respectively. However, 9.9 ± 0.9 and 2.4 ± 0.1% drug was found in lungs in the case of free PTX at 6 and 24 h, respectively. The results show that there was an increase in lung uptake in the case of Lf-coupled formulation as compared to free and uncoupled formulations.
The treatment and management of diseases associated with the lung is difficult with presently available therapeutic systems, as insufficient drug reaches to the lung due to mucocilliary clearance and lung deposition of the medicament. Therefore, practical strategies are required to develop such systems, which can facilitate the drug transport to the lung in sufficient concentration. There are certain specialized carrier transport systems as well as specific receptors present in the lung, which can mediate the uptake of therapeutic agents. Lf-coupled carrier systems seem to be a promising strategy for effective management of lung cancer. Lf is an important class of iron containing glycoprotein, which is abundantly found in bronchial epithelium. It is confined to “serous acini”. These serous acini are found in globular form (Bowes et al., 1981). Lf act as a “non-specific antimicrobial agent” that plays a key role in protecting systemic and secretory surface infections (Alkawash et al., Citation1999). Overexpression of Lf receptors observed during lung cancer could be an ultimate strategy for targeting nanocarriers to the lungs. Previous reports on Lf have also supported this fact (Elfinger et al., Citation2007).
The increased uptake of Lf-coupled SLNs by lung as compared to PTX (free drug) and uncoupled SLNs formulation may be due to the Lf recognition by the receptors present on the cell membrane of bronchial epithelial cells of the lung tissue. Hence, nearly 1.6 times increase in uptake was achieved by Lf-coupled SLNs formulation than uncoupled SLNs formulation whereas 0.5 times from free drug solution. This is due to the presence of Lf receptors on the surface of bronchial epithelial cell of lung which may facilitate the drug transportation by receptor-mediated endocytosis.
Conclusion
The aim of the study was to design SLN loaded with the anticancer drug PTX. The success of drug delivery system lies on one of the principle factors like higher concentration of drug at the site of action with decreased side effects to the surrounding normal tissues. Prepared SLNs coupled with Lf to achieve target-specific delivery of PTX to the lungs. Cytotoxicity and biodistribution studies of PTX-loaded Lf coupled and uncoupled showed that the Lf-coupled formulation is more cytotoxic and deliver the drug at a higher concentration to the target. Based on these results, it can be concluded that the formulations developed in this work may be considered as an effective anticancer drug delivery for cancer chemotherapy. Although this work requires further experimental study.
Declaration of interest
The authors report no conflicts of interest. The authors alone are responsible for the content and writing of this article.
References
- Akunuru S, James Q, Zheng ZY. (2012). Non-small cell lung cancer stem/progenitor cells are enriched in multiple distinct phenotypic subpopulations and exhibit plasticity. Cell Death Dis 3:352–6
- Alkawash M, Head M, Alshami I, Soothill JS. (1999). The effect of human lactoferrin on the MICs of doxycycline and rifampicin for Burkholderia cepacia and Pseudomonas aeruginosa strains. J Antimicrob Chemother 44:385–7
- Bowes D, Clark AE, Corrin B. (1981). Ultrastructural localisation of lactoferrin and glycoprotein in human bronchial glands. Thorax 36:108–15
- Cavalli R, Caputo O, Gasco MR. (1993). Solid lipospheres of doxorubicin and idarubicin. Int J Pharm 89:R9–12
- Dalai S, Pakrashi S, Kumar RSS, et al. (2012). A comparative cytotoxicity study of TiO2 nanoparticles under light and dark conditions at low exposure concentrations. Toxicol Res 1:116–30
- Elfinger M, Maucksch C, Rudolph C. (2007). Characterization of lactoferrin as a targeting ligand for nonviral gene delivery to airway epithelial cells. Biomaterials 28:3448–55
- Fujita K, Matsuda E, Sekine K, et al. (2004). Lactoferrin enhances Fas expression and apoptosis in the colon mucosa of azoxymethane-treated rats. Carcinogenesis 25:1961–6
- Gibbons JA, Kanwar RK, Kanwar JR. (2011). Lactoferrin and cancer in different cancer models. Front Biosci (Schol Ed) 3:1080–8
- Gregory MM. (2012). Locally advanced non-small cell lung cancer: what is the optimal concurrent chemoradiation regimen. Cleve Clinic J Med 79:32–7
- Hu FQ, Zhang Y, Du YZ, Yuan H. (2008). Nimodipine loaded lipid nanospheres prepared by solvent diffusion method in a drug saturated aqueous system. Int J Pharm 348:146–52
- Jain A, Agrawal A, Majumdar S, et al. (2010). Mannosylated solid lipid nanoparticles as vectors for site-specific delivery of an anti-cancer drug. J Control Rel 148:359–67
- Kurmi BD, Gajbhiye V, Kayat J, Jain NK. (2011). Lactoferrin-conjugated dendritic nanoconstructs for lung targeting of methotrexate. J Pharm Sci 100:2311–20
- Liao Y, Du X, Lonnerdal B. (2010). miR-214 regulates lactoferrin expression and pro-apoptotic function in mammary epithelial cells. J Nutr 140:1552–6
- Liao Y, Du X, Lonnerdal B. (2011). Chimeric aptamers in cancer cell-targeted drug delivery. Crit Rev Biochem Mol Biol 46:459–77
- Mukherjee S, Ray S, Thakur RS. (2009). Solid lipid nanoparticles: a modern formulation approach in drug delivery system. Indian J Pharm Sci 71:349–58
- Provencio M, Isla D, Sánchez A, Cantos B. (2011). Inoperable stage III non-small cell lung cancer: current treatment and role of vinorelbine. J Thorac Dis 3:197–204
- Rana S, Sharma S, Singh S. (2012). Iron binding and receptor: lactoferrin and tonb receptors. Int J Biomed Res 3:306–16
- Salim EI, Jazieh AR, Moore MA. (2011). Lung cancer incidence in the Arab league countries: risk, factors and control. Asian Pac J Cancer Prev 12:117–34
- Surapaneni MS, Das SK, Das NG. (2012). Designing paclitaxel drug delivery systems aimed at improved patient outcomes: current status and challenges. ISRN Pharmacol 2012:1–15
- Wang LZ, Ho PC, Lee HS, et al. (2003). Quantitation of paclitaxel in micro-sample rat plasma by a sensitive reversed-phase HPLC assay. J Pharm Biomed Anal 31:283–9
- Xu X, Veenstra TD. (2012). Concentration of endogenous estrogens and estrogen metabolites in the NCI-60 human tumor cell lines. Genome Med 4:2–11
- Yong HY, Eunjoong K, Dai EP, et al. (2012). Cationic solid lipid nanoparticles for co-delivery of paclitaxel and siRNA. Eur J Pharm Biopharm 80:268–73
- Zhao D, Zhao X, Zu Y, et al. (2011). Preparation, characterization, and in vitro targeted delivery of folate-decorated paclitaxel-loaded bovine serum albumin nanoparticles. Int J Nanomed 10:1–8