Abstract
In the present study, the stability of thymopentin (TP5) in bronchoalveolar lavage fluid (BALF) in presence of potential excipients in inhalation formulation was investigated. The content of TP5 was determined using HPLC method. Commonly used bulking agent, dispersibility enhancers and absorption enhancers in inhalation were investigated with respect to the stability of TP5 in BALF. Finally, the stability of TP5 in two inhalation formulations based on the screening experiments was tested in BALF. The results showed that TP5 alone degraded very rapidly in BALF and zero-order enzymatic reaction with a half-life of t0.5 = 49.20 min was observed using 10 times diluted BALF. Among the amino acids examined, leucine and phenylalanine effectively inhibited the enzymolysis of TP5 with prolonged half-life of 112.7 min and 136.2 min, respectively. Nevertheless, slight but insignificant inhibition effect was witnessed for tyrosine, aspartic acid, and lysine; and negligible prevention on the degradation process of TP5 were found for lactose and mannitol. Regarding chitosan, irrespective with molecular weight, the formation of chitosan-TP5 complex improved the stability of TP5 with prolonged t0.5 by 1.8 times. However, along with the improved stability of TP5 in spray-dried chitosan microspheres, the content of TP5 in formulations was reduced to about 75% during preparation process. Thus, leucine was proved to be a prior candidate for inhalation formulation of TP5. Consequently, the results indicate the potential of leucine as carrier for pulmonary delivery of TP5 serving as both stabilizer and dispersibility enhancer.
Introduction
Peptides and therapeutic proteins have been the target of intense research and development in recent years by the pharmaceutical and biotechnology industry (Andrade et al., Citation2011). Due to the labile structure and high molecular weight, they are primarily administered through the parenteral route. Significant efforts have been dedicated to explore new routes, alternative to injection. Among them, inhalation appears as a promising alternative for the administration of such products (Shoyele & Slowey, Citation2006). The large surface area, good vascularization, immense capacity for solute exchange and ultra-thinness of the alveolar epithelium are unique features of the lungs that can facilitate systemic delivery via pulmonary administration of peptides and proteins (Agu et al., Citation2001). So far, several protein-based drugs, such as insulin, human growth hormone, calcitonin and deslorelin, have been reported to reach the systemic circulation following aerosol administration (Jin et al., Citation2011).
Thymopentin (TP5), a synthetic pentapeptide (Arg-Lys-Asp-Val-Tyr), presents a similar biological activity to thymopoietin and many studies have shown the efficacy of TP5 treatment for the therapy of a variety of diseases, including primary and secondary immune deficiencies, autoimmunity, infections, cancer and AIDS (Wang et al., Citation2009). Due to the extensive metabolism in the gastrointestinal tract and extremely short half-life of 30 s in plasma, only intramuscular injections or i.v. infusions of TP5 are available on the market so far (Gao et al., Citation2009). To overcome the poor patient compliance due to the long-term treatment course and repeated injections, a considerable effort has been made to investigate the alternative administration routes of TP5. Various non-injection routes have been tried, such as nasal (Wang et al., Citation2006), buccal, transdermal, ocular, and oral routes (Jin et al., Citation2011), implants, and lung delivery (Gao et al., Citation2009; Wang et al., Citation2009; Li et al., Citation2010). The results confirmed the improved bioavailability and therapeutic efficacy of TP5 through the pulmonary administration. It is well known that dry powder inhalers (DPIs) present longer stability over nebulizers and metered-dose inhalers for the delivery of peptide and protein therapeutics to the lungs. The studies also proved that TP5 DPIs was feasible for the future clinical application.
Although systemic delivery of TP5 by inhalation has gained considerable attention, there is little information available regarding the extent of TP5 metabolism encountered following inhalation to the lung. Even with the relative low activity of enzymes in the lungs, the bioactive macromolecules are prone to rapid degradation and proteolytic digestion with relative longer retention time required before absorption (Stark et al., Citation2008). Consequently, the potential excipients used in DPIs formulation are also expected to protect TP5 against enzymatic cleavage. The commonly used excipients in DPIs include bulking agents, dispersibility enhancers, adhesives and permeation enhancers. Mannitol and lactose, as GRAS (generally recognized as safe) excipients, are primarily used as bulking agents and carrier particles in DPIs delivery. As polyhydric alcohol, similar to lactose, mannitol provides protection against protein destabilization with reduced reducibility. Amino acids and nonionic surfactants are used to enhance the dispersability of powder, and related surface active property provides protection of bioactive molecules, enhances the morphology, fluidity, and dispersibility. Chitosan is often used in DPIs as bioadhesive agent and absorption enhancer. To serve duel role of carrier and enzymatic prohibitor of TP5 in DPIs, it is necessary to screen the commonly used potential carrier for TP5 in DPIs with the highlight of stability of TP5. So far, fundamental information concerning this has not yet been fully elucidated.
Taking these previous results into account, this research mainly focused on the stability of TP5 in lung to screen the potential excipients for TP5 in DPIs. The proteolytic conditions in the lungs were mimicked by bronchoalveolar lavage fluid (BALF), a lung surfactant solution, obtained by fiberoptic bronchoscopy. The stabilities of TP5 with or without excipients were assessed by its resistance to enzymatic degradation in BALF using high pressure liquid chromatography (HPLC). The spray-dried inhalable powder formulations using optimized excipients were prepared and evaluated with regard to the stability of TP5 in BALF.
Materials and methods
Materials
TP5 (purity: 99.11%) was purchased from Soho-Yiming Pharmaceutical Co., Ltd. (Shanghai, China); mannitol, lactose, leucine, valine, phenylalanine, tyrosine, aspartic acid, arginine and lysine were obtained from Bodi Chemicals (Tianjin, China); Chitosan (degree of deacetylation: 91.7%, Mw = 50 kDa, 200 kDa) was purchased from Zhejiang Jinke (Golden Shell) Bio. Chem. Company. Poloxamer 188 (Lutrol® F68) was kindly donated by BASF (Shanghai, China). Normal saline (sodium chloride injection 0.9%) was purchased from Zhiying Pharmaceutical Company (Shenyang, China). Other reagents were analytical or chromatographic grade. Double-distilled water was used throughout the study.
Preparation of BALF
As reported in the literatures, Sprague–Dawley rats (180–240 g) were anesthetized with ether, then exsanguinated with cardiac puncture. A cannula with a diameter of 1.8 mm was inserted into the trachea. Lung and the trachea were lavaged with 2.5 ml warm (37 °C) normal saline and the lavage proceeded 10 times by slowly instilling and withdrawing the same solution. BALF was collected four times (2.5 ml per lavage). For each lavage, the recovery of the lavage fluid was >80%. BALF was maintained in water bath at 37 °C for the following study.
Stability of TP5 in BALF
The stability of TP5 in BALF was studied alone, in the presence of potential inhalation carriers, or in the inhalation formulation. Unless otherwise stated, stability experiments were performed with the following standard procedure. Briefly, 2 mg TP5 and 1 ml of BALF were incubated and normal saline was added to a total volume of 10 ml at 37 °C, in the presence (18 mg) or absence of potential carriers. At pre-determined times up to 12 h, 150 μl aliquots were withdrawn from the incubated mixture, to which 150 μl of cold methanol was added immediately to terminate the reaction. The resulting mixture was centrifuged at 7000 × g for 10 min at 4 °C to remove the precipitated protein. The supernatants were analyzed by HPLC.
HPLC analysis of TP5
TP5 was assayed by a Hitachi D-7000 HPLC system. Samples were injected on a reversed-phase ODS-C18 HPLC column (250 mm × 4.6 mm, 5μm) at room temperature. The mobile phase was methanol (10%, v/v) and 0.02 mol/l phosphate buffer (pH 7.0, 90%, v/v) at a flow rate of 1 ml/min and the detection was performed at 204 nm.
Preparation of spray dried powders
Two inhalation formulations were prepared using spray drying method. The first formulation was based on TP5, mannitol and leucine at the ratio of 10:18:72. Aqueous solutions containing drug and excipients were made with a total powder mass of 1% (w/v). In another formulation, chitosan and leucine were used at the ratio of TP5:chitosan:leucine = 20:40:40. Briefly, TP5 and leucine were dissolved in water and the pH was adjusted to 12 with 1 mol/l NaOH solution. With constant stirring, this solution was mixed with chitosan solution (in 1% acetic acid) and diluted with water to give a final concentration of 0.5% (w/v) for total powder mass. For both formulations, Poloxamer 188 was added at a concentration of 2% (w/w) of the dry powder mass. The final solutions were subsequently spray-dried using an Eyela® SD-1000 spray-dryer (Tokyo Rikakikai Co. Ltd., Japan), with the optimized process parameters: inlet temperature, 110 °C; atomization pressure, 170 kPa; feed rate, 7 ml/min; aspirator setting, 0.6 m3/min. The resultant powders were stored in a desiccator at ambient temperature until analysis.
Statistical analysis
Significance between groups was evaluated by one-way analysis of variance (ANOVA) followed by a Newman-eul post hoc test and the tests were carried out using SPSS 12.11 software (SPSS Inc., Chicago, IL). Differences were considered significant at a level of p < 0.05.
Results and discussion
Selection of enzymatic reaction terminator
To investigate the stability of TP5 in BALF, the drug content in the solution should be monitored. For this, it is necessary to find a terminator for enzymatic degradation process. Considering the nature of enzymes, high temperature, acid, alkali, and organic solvents can denature the activity of enzymes and thereby destroy catalytic activity thereof. Among them, the one with minimal adverse effect on TP5 will be selected.
To choose an appropriate terminator of enzymatic reaction, the stability of TP5 in 0.2 mol/l NaOH solution (pH = 12.76), 36% acetic acid (pH = 1.49), methanol, acetonitrile, and ethanol were investigated. Upon dilution with equal volume of normal saline, TP5 was dissolved at a 2 mg/ml concentration with pure normal saline as the reference. The solutions were maintained in 37 °C water bath and the drug content was monitored at the different intervals as 0, 2, 4, 6, 8, 10, and 12 h.
The results were depicted in . In normal saline, the RSD of peak area of TP5 was 1.16% during 12 h, revealing little effect on the stability of TP5. Negligible decomposition was also observed in methanol and acetonitrile solutions with lower RSD 3.24% and 2.46%, respectively. Obvious and similar degradation behaviors of TP5 in acid and alkali were found and the drug content was reduced to about 87.76% and 87.58% at 12 h, separately. Comparatively, the fastest degradation process was noticed in ethanol solution with the reduction of 74% at 12 h.
From stability standpoint of TP5, together with the consideration of mobile phase of HPLC, methanol was selected as the terminator of enzymatic reaction for stability study of TP5 in BALF.
Stability of TP5 in BALF
To assess the stability of TP5 in BALF, TP5 was incubated with different amounts of BALF, then normal saline was added to 10 ml with a final drug concentration of 0.2 mg/ml. This solution was incubated in 37 °C water bath. All experiments were performed in triplicate.
At the very beginning, based on the same experiment conditions, different amounts of BALF were added as 10 ml, 5 ml and 2 ml. In these solutions, enzymatic decomposition process was too fast to collect enough data of drug concentration for analysis. These results demonstrate the high enzymatic activity of BALF. Thus, keeping other factors unaltered, the stability test of TP5 was carried out using 1 ml BALF. The enzymatic decomposition curve of TP5 was presented in . Under this specific condition, kinetic analysis suggests the linear relationship between percentage of drug remaining (y) and time (x) with high correlation coefficient (R2 = 0.9992), indicating a zero-order enzymatic reaction with a half-life of t0.5 = 49.20 min.
To further investigate the influence of enzymes in BALF on the degradation of TP5, keeping all variables constant, the stability of TP5 was examined in BALF with varied volume as 500, 333, 250, 200, 100, 50, 33 and 25 μl. After linear regression of drug remaining percentage (y) and time (x), the linear regression equations, correlation coefficient and half-life were summarized in . Under the various BALF concentrations, linear relationships between TP5 remaining and time verify the zero-order kinetics of the enzymatic reaction. With increase of BALF concentration, the enzymatic reaction rate increased with a decreased half-life, revealing an enzymatic dependent reaction process. Even for such a low volume of BALF as 33 μl used in this study, the half-life of TP5 was 1125.9 min reflecting a relatively strong enzymatic activity. With further lowered volume of BALF, changes in TP5 remaining within 12 h were negligible with RSD 5.97%.
Table 1. The results of linear regression at various BALF dilution levels.
The stability of TP5 in BALF here examined shows the strong activity of enzymes and fast degradation of TP5. Consequently, it is necessary to select appropriate excipients in DPIs with the intention to protect TP5 in lung.
Influence of lactose and mannitol
As commonly used carriers of inhalation formulation, lactose and mannitol were firstly used to study the potential influence on the stability of TP5 in BALF. The TP5 and the carriers were added to the incubation mixtures at the ratio of 1:9 (w/w). The experiments were performed as described and the results were shown in the . Compared with the control group (pure TP5), with the addition of lactose and mannitol, no significant differences in concentration changes of TP5 were obtained. Likewise, similar half-lives were recorded as TP5:48.6 min; TP5/Lactose:53.2 min; and TP5/Mannitol: 66.8 min. For both mannitol and lactose, little effect on the stability on TP5 in BALF was observed. Upon stability data collected, the commonly used bulking agent as mannitol and lactose could be used in DPIs of TP5 without adverse effect on the stability of TP5.
Influence of amino acids
It has been reported that the amino acids were employed as dispersibility enhancers in spray-dried powders for inhalation, and among them leucine can be used to enhance the dispersibility, aerosolization properties and deposition in the lower regions of the respiratory tract (Li et al., Citation2005; Rabbani & Seville, Citation2005; Seville et al., 2007b). Since TP5 is a pentapeptide (Arg-Lys-Asp-Val-Tyr), the presence of the possible enzymatic product may retard the enzymatic degradation process. Bearing this information in mind, the commonly used amino acids Leu, phenylalanine (Phe), valine (Val), Tyrosine (Tyr), aspartic acid (Asp), arginine (Arg), and lysine (Lys) were investigated with respect to the stability of TP5 in BALF. Among them, Arg, Lys, Asp, Val, and Tyr, as a part of the structure of TP5, were investigated as the possible decomposed products.
Nonpolar amino acids (Leu, Phe, Val, etc.) were firstly examined and the results were presented in . It can be seen the stability of TP5 was greatly improved with addition of Leu or Phe with prolonged half-life 112.7 min, and 136.2 min, respectively. The half-life was more than two-fold increased relative to the pure TP5 (48.6 min). Obviously, both Leu and Phe can inhibit the enzymatic reaction and thereby improve the stability of TP5 in BALF. However, the influence of Val on the stability of TP5 was of minor concern due to insignificant differences in concentration of TP5 at each point compared with the control group.
In the neutral solution, negatively charged Leu (PI = 5.98) and Phe (PI = 5.48) could form complexs with positively charged TP5 through electrostatic interactions. With the similar property of nonpolar amino acids, long hydrophobic alkyl side chain of Leu and Phe gave rise to greater steric resistance drug molecules, thereafter prevented the combination of enzyme with TP5 molecules. However, Val, with short hydrophobic side chain, generates negligible steric resistance effect and plays a minor role in inhibiting enzyme effect.
Additionally, Tyr, Asp, Arg and Lys were also examined, and the results were illustrated in . With an exception of Asp, all experiments were performed as described before. To preclude the influence of pH on the catalytic capacity of enzyme, the pH of Asp solution was adjusted to 6.5–7.0 with 0.1 mol/l NaOH from original pH value of 2.78. The average t0.5 of degradation of TP5 was 63.2 min for TP5/Tyr, 44.3 min for TP5/Asp, 70.4 min for TP5/Arg, and 56.5 min for TP5/Lys, respectively. Compared with the t0.5 of degradation of TP5 (48.6 min), only Arg showed visible protection effect on the stability of TP5 (p < 0.05). However, no obvious difference in degradation profiles of TP5 was observed in the presence of Tyr, Asp, and Lys, separately.
Figure 5. Degradation profile of TP5 in presence of tyrosine, aspartic acid, arginine, and lysine in BALF, respectively.
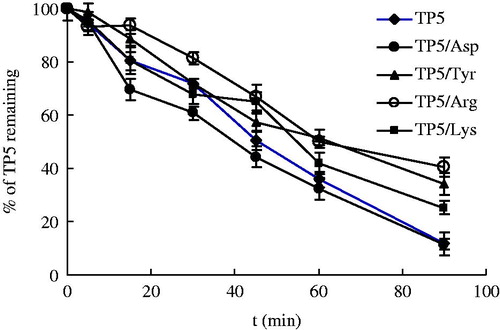
The results indicate that Leu and Phe are effective to inhibit enzymolysis effect, thereby improving the stability of TP5 in BALF. During the experiments, it was found that the enzyme activity in lung was sensitive to pH value greatly and lower activity was observed at lower pH value. In the experiment of Asp, we also did the experiment without pH adjustment with 1 mol/l NaOH, and the concentration of TP5 was not altered at the pH 2.78, indicating a total loss of activity of the enzyme. Structural changes of enzyme may occur at excessively low pH or high pH, thereafter result in a loss of biological activity. Even within the vicinity of optimum pH of the activity of enzyme, the apparent activity of enzymes was different. Because the pH not only influences the dissociation state of substrate molecule, also affects the dissociation state of enzyme molecule. Generally speaking, there is specific molecular state and conformation for the combination of substrate with enzyme. Hence, pH may also possibly affect the dissociation of different groups of enzyme molecules, which are related to the specificity of enzyme and the conformation of active center of enzyme molecules.
Influence of Poloxamer 188 (F68)
It has been reported that the use of non-ionic surfactant in the formulation of aerosol power could be selectively absorbed on the surface of the particles and therefore protect the activity of the protein (Elversson & Millqvist-Fureby, Citation2006). Also, use of surfactant resulted in improved flowability of the spray-dried particles for aerosol (Adler et al., Citation2000; Padhi et al., Citation2006). Triblock co-polymer (Poloxamer 188) was investigated regarding the protect effect on TP5 in BALF. While the amount of surfactant in formulation usually is quite low, based on the same experiment procedure, the ratio between F68 and TP5 is 1:1. As shown in , negligible influence of F68 on the stability of TP5 was obtained with mean t0.5 of 58.0 min. This is possibly because this study was performed in solution, and the effect of F68 in formulation on the stability TP5 could not be fully demonstrated.
Influence of chitosan
Chitosan and its derivatives have been extensively investigated in different formulations due to the mucoadhesive and enhanced permeation properties. Specifically, in lung delivery system, chitosan has been used as carrier for pulmonary drug delivery (Learoyd et al., Citation2008). It is also reported that the formation of complex between chitosan and drugs based on electrostatic interaction could improve the stability of drug. Therefore, as a multifunctional material in formulation, the effect of chitosan on the stability of TP5 in BALF was investigated.
In this experiment, the chitosan was dissolved in 1% acetate acid at a concentration of 5 mg/ml, and pH of solution was adjusted to 6.0–6.3 with 1 mol/l NaOH. For chitosan is positively charged in the solution, it is necessary for TP5 to bear negative charge to form a complex. TP5 (2 mg) was dissolved in 0.2 ml 0.01 mol/l NaOH to make a solution with pH 12 which is higher than isoelectric point of TP5 8.59. Then, the TP5 solution was mixed with chitosan solution to form complex. Afterwards, 1 ml BALF was added and the stability of TP5 was monitored as before. The results were shown in . Irrespective with molecular weight, the formation of chitosan-TP5 complex improved the stability of TP5 with prolonged t0.5 by 1.8 times than pure TP5. Considering the multi-functioning of chitosan in DPIs and the stability effect on TP5, it is worth to investigate the chitosan carrier for TP5 lung delivery.
Stability of TP5 in inhalation formulations in BALF
In view of similar effect of enzymatic reaction inhibition of Phe and Leu, leucine with the superior property as dispersibility enhancer of dry powder was selected to formulate DPIs for TP5 using spray-drying method together with mannitol and F68 (Seville et al., 2007a). For this formulation of TP5: mannitol: leucine at 10:18:72, the dry powder for inhalation prepared here were characterized by size of 3.11 ± 0.25 µm, drug content of 93.3 ± 3.17%, and tapped density of 0.32 ± 0.04 g/m3.
The stability of TP5 in BALF was examined in the same manner as described above, and amount in total DPIs tested is equivalent to 0.2 mg TP5. As depicted in , the degradation half-life of TP5 in DPIs formulation was 95.6 min which is two-fold of pure TP5 (48.6 min). This result was in agreement with the effect of leucine on the stability of TP5 above mentioned. Furthermore, with application of leucine in the formulation, the aerosolization properties were improved greatly (Wang et al., Citation2009). Thus, leucine was proved to be a prior candidate for inhalation formulation of TP5.
Figure 8. Degradation profiles of pure TP5, TP5 loaded DPIs (TP5: mannitol: leucine = 10:18:72), and TP5-loaded chitosan microspheres (CS-MP) (TP5:chitosan:leucine = 20:40:40).
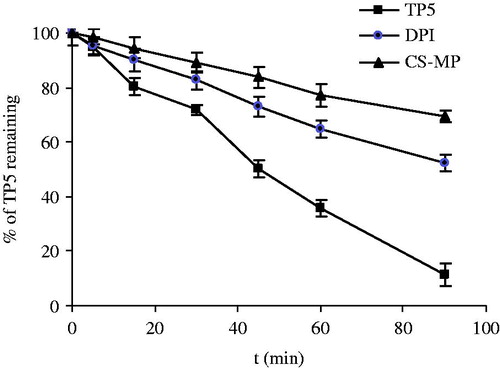
Chitosan has been extensively employed by virtue of the adhesive properties and absorption enhancement especially for protein and peptide (Yamamoto et al., Citation2005). Chitosan-based carriers in micro-dimension have been prepared for pulmonary delivery (Panchal et al., 2012; Yang et al., 2012; Learoyd et al., Citation2008). Based on the results described, formation of complex of chitosan and TP5 reduced the enzymatic degradation rate independent of the molecule weight of chitosan. Here, chitosan and leucine were used at the ratio of TP5:chitosan:leucine = 20:40:40. Similar size of the dry powder as 3.10 ± 0.39 µm was obtained, and the drug content was 75.20 ± 0.22 %, which is much lower than the first formulation. Meanwhile, the tapped density of the dry powder was 0.50 ± 0.05 g/m3.
Here, the stability of TP5 in chitosan microspheres was investigated and the result confirmed the inhibition effect of chitosan (50 kDa) on enzymatic degradation of TP5 with degradation half-life of 142.2 min. After dissolution of chitosan microspheres in BALF, complex of TP5 and chitosan interfere with the combination of TP5 and enzyme and thereafter hinder the enzymatic degradation of TP5. However, along with the improved stability of TP5, the content of TP5 after preparation was reduced to about 75% of the original input. Similarly, it has been reported previously by Yang et al (Yang et al., Citation2007) that after spray-drying with chitosan, the content of calcitonin was significantly reduced. Therefore, from stability of TP5 point of view, the application of leucine together with mannitol is more practical with consideration of content reduction of TP5 loaded chitosan microspheres.
Conclusions
The stability of TP5 in BALF was investigated in presence of commonly used excipients in inhalation formulation including dispersibility enhancers, bulking agents and absorption enhancers. The present findings indicate Phe, Leu and chitosan were effective stabilizers for TP5 in BALF and significantly reduced the enzymatic degradation rate under neutral or close to neutral condition. The stability test in BALF of two inhalation formulations also confirmed this finding. This effective screening of excipients supply a possible selection of Leu as excipient in TP5 containing DPIs serving as dual role of enzymatic inhibitor and inhalation carrier with improved stability of TP5 in BALF.
Declaration of interest
The authors report no conflicts of interest. The authors alone are responsible for the content and writing of this article.
References
- Adler M, Unger M, Lee G. (2000). Surface composition of spray-dried particles of bovine serum albumin/trehalose/surfactant. Pharm Res 17:863–70
- Agu RU, Ugwoke MI, Armand M, et al. (2001). The lung as a route for systemic delivery of therapeutic proteins and peptides. Respir Res 2:198–209
- Andrade F, Videira M, Ferreira D, et al. (2011). Nanocarriers for pulmonary administration of peptides and therapeutic proteins. Nanomedicine (Lond) 6:123–41
- Elversson J, Millqvist-Fureby A. (2006). In situ coating–an approach for particle modification and encapsulation of proteins during spray-drying. Int J Pharm 323:52–63
- Gao J, Ding X, Chu C, et al. (2009). Dry powder inhalations containing thymopentin and its immunomodulating effects in Wistar rats. Eur J Pharm Sci 36:572–9
- Jin X, Huang A, Ping Q, et al. (2011). Box-Behnken optimization design and enhanced oral bioavailability of thymopentin-loaded poly (butyl cyanoacrylate) nanoparticles. Pharmazie 66:339–47
- Learoyd TP, Burrows JL, French E, et al. (2008). Chitosan-based spray-dried respirable powders for sustained delivery of terbutaline sulfate. Eur J Pharm Biopharm 68:224–34
- Li HY, Seville PC, Williamson IJ, et al. (2005). The use of amino acids to enhance the aerosolisation of spray-dried powders for pulmonary gene therapy. J Gene Med 7:343–53
- Li YZ, Sun X, Gong T, et al. (2010). Inhalable microparticles as carriers for pulmonary delivery of thymopentin-loaded solid lipid nanoparticles. Pharm Res 27:1977–86
- Padhi BK, Chougule MB, Misra A. (2006). Optimization of formulation components and characterization of large respirable powders containing high therapeutic payload. Pharm Dev Technol 11:465–75
- Panchal R, Patel H, Patel V, et al. (2012). Formulation and evalution of montelukast sodium – chitosan based spray dried microspheres for pulmonary drug delivery. J Pharm Bioallied Sci 4:S110–11
- Rabbani NR, Seville PC. (2005). The influence of formulation components on the aerosolisation properties of spray-dried powders. J Control Release 110:130–40
- Seville PC, Li HY, Learoyd TP. (2007a). Spray-dried powders for pulmonary drug delivery. Crit Rev Ther Drug Carrier Syst 24:307–60
- Seville PC, Learoyd TP, Li HY, et al. (2007b). Amino acid-modified spray-dried powders with enhanced aerosolisation properties for pulmonary drug delivery. Powder Technol 178:40–50
- Shoyele SA, Slowey A. (2006). Prospects of formulating proteins/peptides as aerosols for pulmonary drug delivery. Int J Pharm 314:1–8
- Stark B, Andreae F, Mosgoeller W, et al. (2008). Liposomal vasoactive intestinal peptide for lung application: protection from proteolytic degradation. Eur J Pharm Biopharm 70:153–64
- Wang J, Lu WL, Liang GW, et al. (2006). Pharmacokinetics, toxicity of nasal cilia and immunomodulating effects in Sprague–Dawley rats following intranasal delivery of thymopentin with or without absorption enhancers. Peptides 27:826–35
- Wang L, Zhang Y, Tang X. (2009). Characterization of a new inhalable thymopentin formulation. Int J Pharm 375:1–7
- Yamamoto H, Kuno Y, Sugimoto S, et al. (2005). Surface-modified PLGA nanosphere with chitosan improved pulmonary delivery of calcitonin by mucoadhesion and opening of the intercellular tight junctions. J Control Release 102:373–81
- Yang M, Yamamoto H, Kurashima H, et al. (2012). Design and evaluation of poly(dl-lactic-co-glycolic acid) nanocomposite particles containing salmon calcitonin for inhalation. Eur J Pharm Sci 46:374–80
- Yang M, Velaga S, Yamamoto H, et al. (2007). Characterisation of salmon calcitonin in spray-dried powder for inhalation. Effect of chitosan. Int J Pharm 331:176–81