Abstract
Aloe-emodin (AE) is a promising anti-tumor candidate for its significant activity against various tumors such as lung cancer, hepatic cancer, breast cancer and so on. Nevertheless, AE is clinically limited due to its poor water solubility and low bioavailability. This study was designed to prepare AE-loaded solid lipid nanoparticles (AE-SLNs) in an attempt to improve the anti-cancer efficacy of AE. The AE-SLNs were prepared with optimized prescription using high pressure homogenization (HPH) technique. Ultimately, the AE-SLNs showed stable particle size at 88.9 ± 5.2 nm, ideal drug entrapment efficiency (EE) of 97.71 ± 0.5% and good stability with regard to zeta-potential as high as −42.8 mV. The in vitro release profiles revealed that AE achieved sustained release by loading into SLNs. Moreover, AE-SLNs showed significantly higher in vitro cytotoxicity against human breast cancer MCF-7 cells and human hepatoma HepG2 cells as compared to the AE solution, while they showed no significant toxicity on human mammary epithelial MCF-10A cells. Hoechst 33342 staining and Annexin V/PI double staining indicated that AE-SLNs induced higher apoptotic rates in MCF-7 cells. Further study elucidated that the improved anti-cancer efficacy may be attributed to the increased cellular uptake of AE. Based on these findings, we believe that the development of AE-SLNs is an effective way for improving the anti-cancer efficacy of AE.
Introduction
Cancer has become the biggest threat to the public health due to the high fatality rate and death cases occurred every year all over the world (Siegel et al., Citation2012). However, since the pathogenesis of cancers is quite complicated, there is still a lack of effective treatment options for cancer patients. Aloe-emodin (AE), a naturally occurring bioactive anthraquinone, is mainly isolated from either Rheum palmatum L. or Aloe barbadensis Miller. Recent studies have demonstrated that AE is a significant antineoplastic agent for its remarkable anti-cancer activity on multiple tumor cells with multi-channel mechanisms, including disruption of cell cycle, induction of apoptosis, inhibition of metastasis and angiogenesisas, as well as strengthening the immune cell function (Chen et al., Citation2004, Citation2007, Citation2010; Harlev et al., Citation2012). In spite of its remarkable effect, AE suffers from limitations which severely restricted its clinical application, including poor aqueous solubility, bad absorption and low bioavailability. As conventional drug formulations could not satisfy the demand for clinical therapy, it is desirable to develop more efficient formulations. Even though there are β-cyclodextrin inclusion compounds and liposomes developed for further evaluation, the limited inclusion capability of β-cyclodextrin and easy oxidation of phosphatidylcholin are still awkward (Rajewski & Stella, Citation1996; Szulc et al., Citation2002; Chou & Liang, Citation2009; Guo et al., Citation2009). Therefore, looking for effective methods to ameliorate these limitations is necessary for achieving better efficacy of AE.
During the recent decades, novel drug delivery systems have presented superior advantages in improving drugs’ efficacy and patients’ compliance. In particular, the mention and application of solid lipid nanoparticles (SLNs) have absorbed intense concern. Taking the various advantages into consideration, SLN is regarded as a promising drug carrier system (Almeida & Souto, Citation2007). For instance, it could improve drugs’ bioavailability through increasing the specific surface area and adhesion of drugs, gradually resulting in better solubility in gastrointestinal tract (Wang et al., Citation2009). Besides, stronger targeting ability could also be obtained as a result of the better cytophagy and accumulation in tissues and organs (Wang et al., Citation2012a). In addition, SLN has sustained-release property which guarantees continued treatment and better efficacy (Zhang et al., Citation2011). Taking the above advantages into account, we decide to prepare AE loaded SLNs, in order to improve its water solubility and enhance its anti-cancer effect.
Materials and methods
Materials
AE was obtained from Nanjing Zelang Medical Technology Co., Ltd. (>98%, Jiangsu, China). Glycerol monostearate (GMS) was supplied by Tianjin Bodi Chemical Co., Ltd. (Tianjin, China). Glyceryl behenate, glyceryl tristearate and glyceryl trimyristate were purchased from Guangzhou Chemical Reagent Factory (Guangdong, China). Soybean lecithin was purchased from Shenyang Tianfeng Biological Pharmaceutical Co., Ltd. (Liaoning, China). Poloxamer 188 (F68) and Poloxamer 407 (F127) were supplied by BASF (German). Tween-20 was purchased from Sigma (USA). Tween-80 was supplied by Nanjing Well Chemical Corp., Ltd. (Jiangsu, China). Dulbecco’s modified eagle medium (DMEM), Fetal Bovine Serum (FBS), Phosphate-buffered saline (PBS), Penicillin-streptomycin (PS) and 0.25% (w/v) trypsin/1 mM EDTA were purchased from Gibco. MilliQ water was obtained from a water purification system (Millipore, USA).
Quantification analysis of AE-SLNs by high performance liquid chromatograph (HPLC)
A reversed phase HPLC method was established for determining AE content in SLNs. Samples were detected by an Agilent 1200 series system equipped with DAD detector, autosampler and workstation, besides, an Agilent Eclipse XDB-C18 column (4.60 mm × 250 mm, 5 μm) was employed for separation. The mobile phase, which consisted of acetonitrile and water (50:50, v/v) was filtered through 0.22 μm filter and degassed for 10 min before analysis. All samples were eluted at a flow rate of 1 ml/min and detected at 254 nm. Aliquots of 10 μl samples were injected into the system and analyzed at 25 °C. The retention time of AE was about 8 min. A sensitive quantitative assay of AE was ultimately built with a calibration linear curve of A = 39.71C + 6.5136 within the concentration of 2.0–150.0 μg/ml (R2 = 0.9999). The average recovery of three concentrations was 98.51%, and the RSD for precision of intra- and inter-day were 0.34% and 0.93%, respectively.
Preparation of AE-SLNs
High pressure homogenization (HPH) was utilized for preparation of AE-SLNs (Li et al., Citation2006). Pharmaceutical lipid material and drug were precisely weighed and dissolved in a water bath at 70–80 °C. Meanwhile, a certain amount of surfactant was dissolved in water at the same temperature and slowly added to the melted lipid mixture with mechanical stirring at a dropping speed of 1 ml/min. Subsequently, it was continuously stirred for 20 min and then the pre-emulsion was passed through a high pressure homogenizer. The AE-SLNs suspensions were thus obtained and stored in a refrigerator at 4 °C. Besides, lyophilized AE-SLNs were also obtained for further physical characterizations.
Characterization of AE-SLNs
Measurement of average diameter and zeta potential
In order to access the optimal preparation condition, the mean particle size and zeta potential of prepared AE-SLNs dispersion were determined by a ZSP Zetasizer (Malvern, UK). All the samples were diluted with MilliQ water to reach a suitable concentration before measurement.
Differential scanning calorimetry (DSC)
DSC analysis was conducted on TA-60WS Thermal Analyzer (Shimadzu, Japan) to evaluate the physical status of AE in SLNs. Samples were accurately weighed (approximately 2 mg) and sealed in aluminum pans. Nitrogen was used as flush gas. The scan was recorded from 40 to 300 °C at a heating rate of 10 °C/min, using an Al2O3 pan as reference.
Transmission election microscope (TEM) image
The morphological characterization of AE-SLNs was observed by TEM (JEM-1400, Jeol Ltd., Japan). One drop of AE-SLNs dispersion was placed over a copper TEM grid, then the grid was stained with 1% uranyl acetate for 10 min. Finally, the morphology of AE-SLNs was imaged under the TEM.
Determination of drug entrapment efficiency
Ultrafiltration method (Wang et al., Citation2012b) was used for entrapment efficiency (EE) determination of AE-SLNs dispersion. Briefly, 2 ml of AE-SLNs dispersion was precisely transferred into the inner chamber of a centrifugal filter tube (10 000 Da molecular weight cut-off, Millipore, USA). Then the tube was centrifuged at 4500 rpm for about 15 min at room temperature. Subsequently, the particles and encapsulated drug were cut off by the filter and remained in the inner chamber, however, the aqueous dispersion medium could pass through the filter membrane thus concentrate on the outer chamber. After isolation, aqueous dispersion medium was detected by HPLC to measure the amount of free drug. Besides, initial drug amount was confirmed by measuring the concentration of AE-SLNs dispersion.
Ultimately, the EE of AE-SLNs was calculated with the following equation:
Among which, Wfree is the amount of free drug after isolation, while Winitial represents for the amount of drug used for assay.
Evaluation of in vitro drug release
The in vitro drug release of AE-SLNs was investigated by dialysis bag method at 37 °C. The dialysis bag (Biotopped, USA) with a molecular weight cut-off size of 8000–14 000 Da was used, and PBS (pH 7.4) containing 0.2% (w/v) sodium dodecyl sulfate (SDS) was employed as dissolution medium. The dialysis bag could retain SLNs while allowing the diffusion of free drug into the dissolution medium. In brief, 1 ml of AE-SLNs dispersion was added into the dialysis bag with the two ends fixed by strings. The dialysis bag was soaked in 50 ml dissolution medium and shaken at 100 rpm under 37 °C. At fixed time intervals, 1 ml of dissolution medium was withdrawn and replaced with 1 ml of fresh medium. The drug contents in each sample were analyzed by HPLC method and the cumulative percentage of AE released was calculated accordingly.
Cell lines and cell culture
Human breast adenocarcinoma cell line MCF-7, human hepatocellular carcinoma cell line HepG2 and human breast epithelial cell line MCF-10A were purchased from the American Type Culture Collection (ATCC). Cells were cultured in DMEM medium supplemented with 10% (v/v) heat-inactivated FBS and antibiotics (100 U/ml penicillin and 100 μg/ml streptomycin) at 37 °C under 5% CO2.
MTT assay
Cell viability was estimated by MTT assay as described previously. MTT assay is a quantitative method used for cell viability determination (Collier & Pritsos, Citation2003). MCF-7, HepG2 and MCF-10A cells were seeded in 96-well plate at a density of 5 × 103 in 100 μl of medium per well. After growing adherence for 24 h, cells were incubated with different concentrations of free AE, AE-SLNs and blank nanoparticles (B-SLNs) for 24 and 48 h, repectively. After incubation, the medium was replaced with serum-free medium containing 1 mg/ml of 3-(4, 5-dimethylthiazol-2-yl)-2, 5-diphenyltetrazolium bromide (MTT) for 4 h. Then the supernatant was aspirated and formazan crystals were dissolved in 100 μl of DMSO. The absorbance of the resulting solution was determined by a microplate reader (SpectraMax M5, Molecular Devices, USA) at 570 nm. Cell viability was calculated by comparing the OD value of treated with control cells.
Hoechst 33342 staining
Apoptotic morphology of cell nucleus was evaluated by Hoechst 33342 fluorescent staining (Wang et al., Citation2013). MCF-7 cells were seeded in a 96-well plate at a density of 5 × 103 in 100 μl of medium per well. After incubation for 24 h, cells were well adherent and then treated with free AE (2.5 μM), AE-SLNs (2.5 μM) and B-SLNs for 48 h. After treatment, cells were washed with PBS thrice and fixed with 4% PFA (m/v) for 15 min. Afterwards, cells were washed by PBS twice and stained with Hoechst 33342 (5 μg/ml) at room temperature for another 15 min. Apoptotic morphology in MCF-7 cell nucleus was observed by Incell Analyzer 2000 (GE Healthcare Life Sciences, USA).
Annexin V/PI staining assay
Annexin V/PI staining assay is a sensitive method used for detecting apoptotic cells (Schutte et al., Citation1998). MCF-7 cells were plated in 6-well plate at a density of 2 × 105 cells in 2 ml of medium per well. After 24 h incubation allowing to adhere, cells were treated with free AE (2.5 μM), AE-SLNs (2.5 μM) and B-SLNs for 48 h. Afterwards cells were trypsinized and collected by centrifuging at 300 g for 5 min. The collected cells were washed twice by PBS and gently resuspended in 100 μl binding buffer containing 3 μl of Annexin-FITC and 5 μl of PI solution. Then the cells were incubated at room temperature for 15 min. Flow Cytometry Analysis (BD FACS Canto TM, BD Biosciences, USA) was carried out to indentify and quantitate apoptotic cells.
Cellular uptake of AE-SLNs
The cellular uptake of AE-SLNs was carried out according to a fluorescence method (Pecere et al., Citation2003). MCF-7 cells (1 × 105/well) were seeded in a 6-well plate the day before and then incubated with complete drug-free and AE loaded SLNs medium at a concentration of 5 μM. After fixed time intervals, the cells were washed twice in PBS to remove most of the drug residues. Then, cells were incubated with 1% Triton for 15 min at 4 °C; scraped and centrifuged for 20 min at 13 000g. The resulting supernatants were the cell lysates. Afterwards, 50 μl of supernatant was diluted in 200 μl methanol and vortexed for 5 min. The diluents were then supplied to fluorescence assay by using fluorometer (excitation: 410 nm; emission: 510 nm) to measure the concentrations of AE in lysates. Meanwhile, protein concentrations of the supernatants were determined by Bradford colorimetric assay using reagents from Bio-Rad (USA) and bovine serum albumin. Results are expressed as microgram per milligram of protein.
Results and discussion
Preparation of AE-SLNs
Optimization of HPH condition
HPH is extensively used for preparation of SLNs on account of the small particle size and narrow distribution. Since it is convenient and able to expand for industrial production, HPH is promising on SLNs preparation (Li et al., Citation2006). In order to confirm the optimal conditions of HPH, we investigated three pressures (600, 800 and 1000 bar) and monitored the variation of particles within 10 cycles. As shown in , we could find that SLNs homogenized at 600 bar showed a relatively large particle size, while the pressure of 1000 bar resulted in the lowest particle size. Hence 1000 bar was determined as the optimal pressure. In addition, the result revealed that the mean diameter significantly decreased with the increase of cycle times. It was obvious that the particle size reduced rapidly at the beginning and got gentle afterwards, which indicated that variation trend of mean diameter was not constant for it hardly reduced once reached an extreme. This might associate with enhancement of particle coalescence, which occurs as a result of the high kinetic energy of the particles. As for the pressure of 1000 bar, the particle size got smaller with the increase of homogeneous cycles, and became constant after 8 cycles. Therefore, 1000 bar and 8 cycles were confirmed as the optimal homogenization condition.
Figure 1. (A) Chemical structure of AE. (B) Effect of HPH pressure and cycle times on particle size. (C) Effect of lipid concentration on particle size and zeta-potential of AE-SLNs at 10, 20 and 30 mg/ml. (D) Effect of different proportion of surfactant to lipid on particle size and zeta-potential.
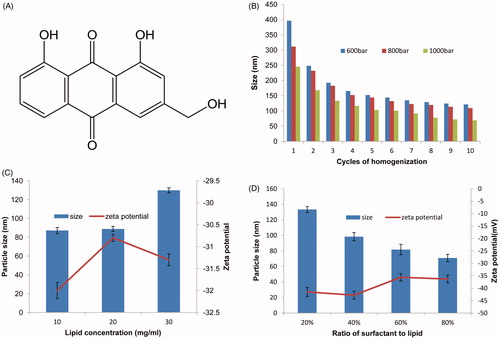
Effect of lipid material and concentration
In order to select a suitable lipid for AE-SLNs preparation, lipid materials such as glyceryl monostearate, glyceryl behenate, glyceryl tristearate, glyceryl trimyristate, soybean lecithin and stearic acid were employed for preparation. Except for Soybean lecithin, the above lipid materials could result in translucent dispersion. However, SLNs dispersion prepared by glyceryl tristearate, glyceryl trimyristate and stearic acid were found to flocculate after stored at 4 °C overnight. Therefore, there were only glyceryl monostearate and glyceryl behenate could form clear and translucent dispersion after long time storage. Ultimately, we chose glyceryl monostearate as the optimal lipid on account of its higher solubility and smaller particle size when compared with glyceryl behenate ().
Table 1. Effect of lipid materials on particle size and zeta potential of AE-SLNs.
In addition, lipid concentration also played an important role in the physicochemical property of SLNs. Hence, lipid concentration of 10, 20 and 30 mg/ml were investigated. Taking the particle size and zeta-potential into consideration, the lipid concentration was of great influence. In the tested concentration range, the average diameter of SLNs rose with the increase of lipid concentration. Besides, lipid concentration also displayed significant effect on the zeta-potential, the important indicator of the stability. This may be determined by the properties of lipid materials. It was noteworthy that the increasing lipid content in most cases would result in larger particles due to the decrease of the homogenization efficiency and increase in particle agglomeration, which may also influence the stability of particles (Mehnert & Mader, Citation2012). Therefore, synthesizing with the two factors, we chose 10 mg/ml as the lipid content in order to obtain smaller and more stable particles.
Effect of surfactant and concentration
A surfactant is another important factor influencing the quality of SLNs. In aqueous SLNs colloidal dispersion, the surfactant is an essential asset for stabilizing the lipid particles in water dispersion and preventing agglomeration. Hence, we chose F68, F127, Tween 20, Tween 80 as emulsions to investigate the influence of surfactants. Through , we could conclude that smaller particles could be obtained with the use of F68 and Tween 80. Besides, taking account of zeta-potential whose absolute value was better to be greater than 30 in order to ensure a good stability, Tween 80 was selected as the suitable emulsion.
Table 2. Effect of different surfactants on particle size and zeta potential of AE-SLNs.
Afterwards, the effect of surfactant concentration was carried out with the content of 20–80% to lipid (w/w). As expected, an increase in surfactant content resulted in a smaller particle diameter, as well as a slight decrease in zeta-potential. As shown in , the particle size was 133.3 ± 3.8 nm with 20% surfactant to lipid, whereas it reduced to 70.9 ± 7.6 nm at the content of 80%. This may be related to the reason that the high content of the emulsifier could either reduce the surface tension of particles or facilitate the particle partition during the process of homogenization. Generally, the excessive emulsifiers might rapidly cover the new surfaces of particles and compete with the agglomeration during the homogenization process (Mehnert & Mader, Citation2012). This was conducive for particle partition, and smaller particle sizes could be achieved. As a nonionic surfactant, Tween 80 is capable of steric stabilization and prevention of particle aggregation. Consequently, our finding revealed that it has an optimal content of 40% to lipid concentration.
Characterization of AE-SLNs
Particle size, zeta-potential and morphology of AE-SLNs
The particle size and zeta-potential of AE-SLNs were measured by Malvern Zetasizer. As shown in , the mean particle size of AE-SLNs in three batches was 88.9 nm, and the mean zeta-potential was −42.8 mV. The particles seemed spherical shaped with monodispersed size distribution under TEM image (). Moreover, the formulation displayed stable quality within one month.
Figure 2. Physical characterization result of AE-SLNs. (A) The particle size distribution of AE-SLNs. (B) Transmission electron microscopy of AE-SLNs.
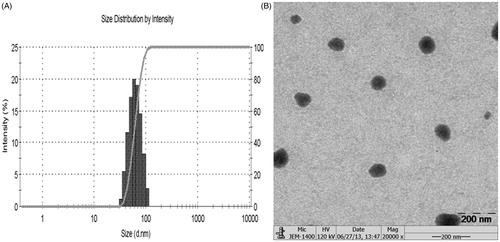
Table 3. The results of formulation assay.
Determination of drug entrapment efficiency
It was revealed that drug dissolved in SLN dispersion mainly comprised two forms, one was either encapsulated in lipid nucleus or absorbed by the surface of nanoparticles, the other existed as a free drug in aqueous dispersion. Actually, different existence forms of drug notably influenced its in vivo fate including absorption, distribution, metablism and excretion, which ultimately determined the drug’s curative effect. Therefore, entrapment efficiency is important for estimating the therapeutic potential of drug loaded SLNs. Our study concluded that ultrafiltration was the most convenient, sensitive and accurate method for entrapment efficiency (EE) evaluation of AE-SLNs. The average EE of AE-SLNs in three batches was 97.71%, with RSD of 0.50% through ultrafiltration. However, though mini-column centrifugation could be used for determination, it could also separate the drug absorbed on the surface of nanoparticles as free drug, which may lead to lower EE value comparing with actual value. Besides, with consideration that dialysis may result in drug leak-out from the nanoparticles, the result of dialysis was also relatively lower than the actual value. Therefore, ultrafiltration was the most suitable method for entrapment efficiency determination of AE. Meanwhile, the high accuracy, good reproducibility and recovery have verified that ultrafiltration was a convenient, sensitive and accurate method for estimating the quality of AE-SLNs.
DSC analysis
DSC is frequently used for investigating the melting and recrystallization behavior of materials and nanoparticles. The thermogram of AE, lipid material, mixture and AE-SLNs were shown in . It was obvious that the characteristic peaks of AE crystals and lipid material appeared at 224 °C and 62 °C, respectively. Meanwhile, the characteristic peaks of AE and lipid material could also be found in physical mixture. However, the thermogram of AE-SLNs presented only one characteristic peak at 51 °C. The disappearance of characteristic peak of AE in the thermogram of SLNs revealed that AE had been encapsulated in the lipid nanoparticles, indicating that AE existed in amorphous rather than crystal form in nanoparticles (Noack et al., Citation2012).
Figure 3. DSC thermogram of free AE, lipid material, physical mixture and AE-SLNs. The characteristic peaks of AE crystals and lipid material appeared at 224 °C and 62 °C respectively, but the thermogram of AE-SLNs presented only one characteristic peak at 51 °C, revealing that AE-SLNs have been successfully prepared.
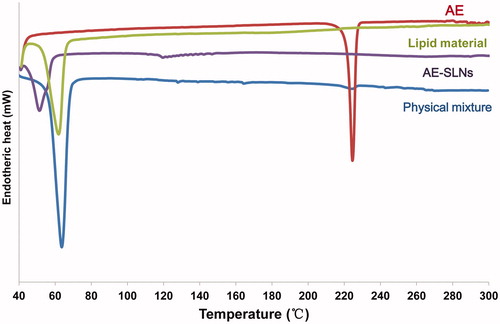
Drug release profiles of AE-SLNs
SLNs are demonstrated to have a shell-core nanometer structure comprising a lipid core surrounded by surfactants. In AE-SLNs, AE was both encapsulated in the lipid core and adhered to the surface of the particles. Therefore, the drug needs to be released from the nanocapsules rather than being directly exposed to the dissolution medium. It took more time to release, thus resulting in a sustained release. In our study, the release profiles of AE-SLNs exhibited sustained drug release over a period of 48 h while AE possessed a biphasic pattern, including burst drug release and sustained drug release. The sustained release effect of AE-SLNs was essential to prolong the retention time of AE and ensure good efficacy (Muller et al., Citation2000) ().
In vitro anti-cancer study
In vitro cytotoxicity of free AE and AE-SLNs
MTT assay is a conventional method used for evaluating cytotoxicity. The succinate dehydrogenase (SDH) in metabolically active cells could reduce MTT to formazan crystals, which is directly associated with the quantity of living cells. In our study, the cytotoxicity of free AE, AE-SLNs and B-SLNs in different cancer cell lines were carried out. In test concentration, free AE (0.1–2.5 μM) showed inconspicuous effect on cell viability, while treatment of AE-SLNs at the same concentration in both MCF-7 and HepG2 cells produced significant concentration dependent inhibition on cell proliferation. Thus the result revealed that AE-SLNs could enhance the cytotoxicity effect of AE, and similar enhanced result could also conclude from higher concentration comparing to free AE. Besides, though B-SLNs showed mild cytotoxicity effect on the two cancer cells, it was in stark contrast to the effect of AE-SLNs, where indicated the potential therapy of AE-SLNs. Accumulating experimental data have revealed SLNs to be a promising carrier with expected low toxicity due to the biodegradability of lipid materials, that is much better than other nanoparticulate delivery systems such as polymeric particles (Patel et al., Citation2013). Besides, the toxicity of free AE, AE-SLNs and B-SLNs to normal cells was also investigated on MCF-10 A cells, where almost no toxicity was found among the three groups ().
Figure 5. Cytotoxicity analysis of free AE, B-SLNs and AE-SLNson MCF-7, HepG2 and MCF-10 A cells at the concentration of 0.1–2.5 μM. Data are shown as mean ± S.D. (n = 3). *p < 0.05, **p < 0.01, AE-SLNs versus Free AE.
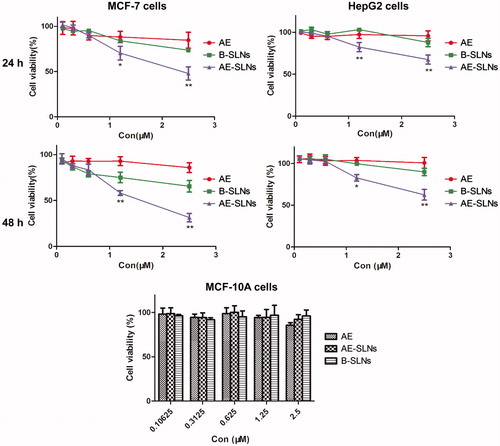
Previous studies have demonstrated that nanoparticulate drug delivery system could enhance the anti-cancer effect of many chemotherapeutic drugs. Our study also revealed that SLNs could enhance the anti-cancer activity, which is a promising system for carrying an anti-cancer drug. The enhancement mechanism is complicated and may include the following aspects. Firstly, because of the small particle size, nanoparticles could penetrate through the cell membrane through endocytosis and phagocytosis which contributed to the accumulated drug uptake. Secondly, owing to the lipid bilayer of cell membrane, the adhesion of nanoparticles on cell membranes may influence the structure of lipid bilayer and functions of biomacromolecule adhered to the membranes, thus the cell permeability is altered and more nanoparticles are internalized into the cells. Thirdly, as the stability of drugs increases after being encapsulated into SLNs, it is possible to retard drug degradation and prolong the retention time in cells, thereby improving the bioavailability of drugs.
Apoptosis assay on MCF-7 cells
Hoechst 33342 and Annexin V/PI staining assay were further used for evaluating the anti-cancer effect of AE-SLNs. Hoechst 33342 was widely used for detecting apoptotic cell nucleus as it was a fluorochrome that could stain DNA (Kiechle & Zhang, Citation2002). The nucleus morphology of apoptotic cells turn out to be irregular shaped compared with normal cells. As shown in , the nucleus morphology of MCF-7 cells in the control group was round, however, it became blebbing after treatment with free AE, and it was obvious that the quantity of apoptotic cells in AE-SLNs was much larger than the free AE. The above results indicated that AE-SLNs could enhance the anti-cancer activity of free AE, which may be associated with the apoptosis induction pathway.
Figure 6. Hoechst 33342 fluorescent staining to detect apoptotic morphology in breast cancer cells, MCF-7 cells were treat with B-SLNs, Free AE and AE-SLNs at 2.5 μM for 48 h. The cell morphology was observed by Incell Analyzer 2000 (GE Healthcare Life Sciences, USA).
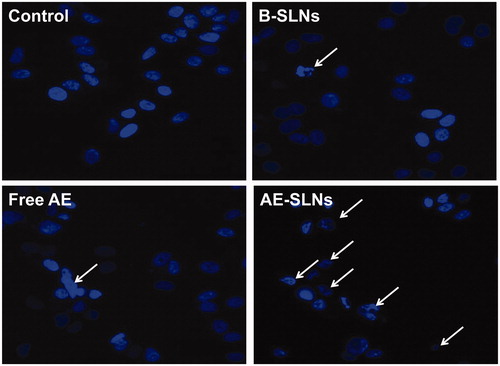
Besides, Annexin V/PI staining assay was also applied for detecting apoptotic cells. Annexin V/PI staining is sensitive for Annexin V could label the extrinsic phosphatidyl serine (PS) with fluorescence, this is an essential mark in distinguishing them from normal cells. Besides, another fluorescent marker PI is sensitive in detecting necrotic cells as it could not internalize into normal cells. As shown in , the percentages of apoptotic cells in control, B-SLNs and free AE was 1.6%, 2.6% and 3.9% respectively, after treatment at the concentration of 2.5 μM for 48 h. However, the percentage of apoptotic cells in AE-SLNs was up to 70%, which indicated that free AE slightly induced cell apoptosis, thus AE-SLNs significantly promoted the apoptosis effect in comparison with free AE. Meanwhile, as B-SLNs showed negligible apoptosis activity on MCF-7 cells, the mild cytotoxity B-SLNs might attribute to other mechanism of the excipients. Therefore, we could draw a conclusion that AE-SLNs could enhance the anti-cancer effect of AE, whose mechanism was mainly associated with the enhanced induction of apoptosis.
Figure 7. Quantitative apoptotic measurement in MCF-7 cells with treatment of Free AE, B-SLNs and AE-SLNs. (A). Results of dose dependent effect are expressed as dot plot of AnnexinV-FITC versus PI and representative values are shown. (B). The results are expressed as bar chart. Data as mean ± S.D. (n = 3). **p < 0.01, AE-SLNs versus Free AE.
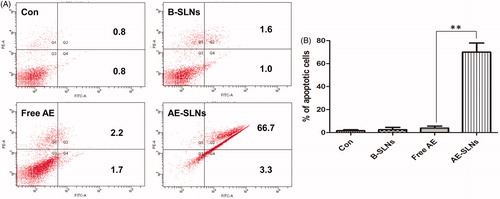
Cellular uptake study
The cellular uptake process of AE in MCF-7 cells was carried out to confirm the anti-cancer effect of AE-SLNs by detecting the uptake amount of AE in MCF-7 cells using spectrofluorimetry. The quantitative estimation of AE cellular uptake showed prominent difference between AE and AE-SLNs. It was obvious that the cellular uptake amount of AE in SLNs was higher than free AE after half an hour’s incubation, and the discrepancy became larger as time increased. Statistical analysis showed that the uptake amount was significantly different between free AE and AE-SLNs. Due to the small mean diameter, SLNs could be internalized into cells through multi-channel such as endocytosis, membrane fusion and adsorption on the surface of the cells, yet hydropholic drug could be only internalized into the cell via the dissolution of cytomembrane (Yuan et al., Citation2008; del Pozo-Rodriguez et al., Citation2009). Therefore, more drugs could be uptaken into cells in comparison with free drug. Besides, as the drug needs to release from the particles, the retention time prolonged so that higher concentration may remain in cells. The increasing drug uptake was an important factor which accounted for the efficacy enhancement of AE-SLNs ().
Conclusions
The present work provided an optimized method for preparing AE-SLNs, which included 10 mg/ml of glyceryl monostearate as lipid materials, 40% (Tween 80 to GMS) of surfactant as emulsion using HPH technology. AE-SLNs with small particle size, good stability and high entrapment efficiency were achieved under the optimal method. The in vitro drug release profile of AE-SLNs revealed that AE was sustainably released from SLNs, which was important for improving the bioavailability of AE. Moreover, in vitro anti-cancer study indicated that AE-SLNs could enhance the anti-cancer effect of AE through inducing apoptosis of tumor cells. Further cellular uptake investigation demonstrated that the enhanced anti-cancer effect of AE-SLNs may be attributed to the increased cellular uptake of AE. Based on the above findings, we could draw a conclusion that the preparation of AE-SLNs is an effective way for improving the anti-cancer efficacy of AE. However, this study is lacking in clear understanding of how AE-SLNs work at molecular levels and all of these studies were carried out just in in vitro models, further mechanistic and in vivo studies still deserve research.
Declaration of interest
The authors report no conflicts of interest in this work. This study was supported by the Macao Science and Technology Development Fund (077/2011/A3, 102/2012/A3) and the Research Fund of the University of Macau (SRG025-ICMS13-CMW, MYRG 208 (Y3-L4)-ICMS11-WYT, MRG012/WYT/2013/ICMS).
References
- Almeida AJ, Souto E. (2007). Solid lipid nanoparticles as a drug delivery system for peptides and proteins. Adv Drug Deliv Rev 59:478–90
- Chen HC, Hsieh WT, Chang WC, et al. (2004). Aloe-emodin induced in vitro g2/m arrest of cell cycle in human promyelocytic leukemia hl-60 cells. Food Chem Toxicol 42:1251–7
- Chen SH, Lin KY, Chang CC, et al. (2007). Aloe-emodin-induced apoptosis in human gastric carcinoma cells. Food Chem Toxicol 45:2296–303
- Chen YY, Chiang SY, Lin JG, et al. (2010). Emodin, aloe-emodin and rhein inhibit migration and invasion in human tongue cancer scc-4 cells through the inhibition of gene expression of matrix metalloproteinase-9. Int J Oncol 36:1113–20
- Chou TH, Liang CH. (2009). The molecular effects of aloe-emodin (ae)/liposome-ae on human nonmelanoma skin cancer cells and skin permeation. Chem Res Toxicol 22:2017–28
- Collier AC, Pritsos CA. (2003). The mitochondrial uncoupler dicumarol disrupts the mtt assay. BiochemPharmacol 66:281–7
- Del Pozo-rodriguez A, Solinis MA, Gascon AR, et al. (2009). Short- and long-term stability study of lyophilized solid lipid nanoparticles for gene therapy. Eur J Pharm Biopharm 71:181–9
- Guo Y, Cui DS, Wang ZM. (2009). Determination of binding constant between β-cyclodextrin and aloe-emodin by capillary electrophoresis. Chin J App Chem 26:492–4
- Harlev E, Nevo E, Lansky EP, et al. (2012). Anticancer potential of aloes: antioxidant, antiproliferative, and immunostimulatory attributes. Planta Medica 78:843–52
- Kiechle FL, Zhang X. (2002). Apoptosis: biochemical aspects and clinical implications. Clin Chim Acta 326:27–45
- Li YC, Dong L, Jia A, et al. (2006). Preparation of solid lipid nanoparticles loaded with traditional chinese medicine by high-pressure homogenization. Nan Fang Yi Ke Da Xue Xue Bao 26:541–4
- Mehnert W, Mader K. (2012). Solid lipid nanoparticles production, characterization and applications. Adv Drug Deliv Rev 64:83–101
- Muller RH, Mader K, Gohla S. (2000). Solid lipid nanoparticles (sln) for controlled drug delivery – a review of the state of the art. Eur J Pharm Biopharm 50:161–77
- Noack A, Hause G, Mader K. (2012). Physicochemical characterization of curcuminoid-loaded solid lipid nanoparticles. Int J Pharm 423:440–51
- Patel M, Souto EB, Singh KK. (2013). Advances in brain drug targeting and delivery: limitations and challenges of solid lipid nanoparticles. Expert Opin Drug Deliv 10:889–905
- Pecere T, Sainella F, Saltata C, et al. (2003). Involvement of p53 in specific anti-neuroectodermal tumor activity of aloe-emodin. Int J Cancer 106:836–47
- Rajewski RA, Stella VJ. (1996). Pharmaceutical applications of cyclodextrins. 2. In vivo drug delivery. J Pharm Sci 85:1142–69
- Schutte B, Nuydens R, Geerts H, et al. (1998). Annexin V binding assay as a tool to measure apoptosis in differentiated neuronal cells. J Neurosci Methods 86:63–9
- Siegel R, Naishadham D, Jemal A. (2012). Cancer statistics, 2012. CA-Cancer J Clin 62:10–29
- Szulc J, Dudzik M, Woyczikowski B, et al. (2002). Liposomes – therapeutic progress and technological problems. Pol Merkur Lekarski 12:164–8
- Wang J, Chen J, Ye N, et al. (2012a). Absorption, pharmacokinetics and disposition properties of solid lipid nanoparticles (slns). Curr Drug Metab 13:447–56
- Wang Q, Wang Y, Wang LF. (2009). Phamacokinetic study of solid lipid nanoparticles as drug delivery systems. Gansu J Tradit Chin Med 22:79–81
- Wang SP, Chen TK, Chen RE, et al. (2012b). Emodin loaded solid lipid nanoparticles: Preparation, characterization and antitumor activity studies. Int J Pharm 430:238–46
- Wang SP, Zhong ZF, Wan JB, et al. (2013). Oridonin induces apoptosis, inhibits migration and invasion on highly-metastatic human breast cancer cells. Am J Chin Med 41:177–96
- Yuan H, Miao J, Du YZ, et al. (2008). Cellular uptake of solid lipid nanoparticles and cytotoxicity of encapsulated paclitaxel in a549 cancer cells. Int J Pharm 348:137–45
- Zhang HB, Zhu XY, Zhang TJ. (2011). Advances in study on nanotechnology used in chinese materia medica preparation. Drugs Clinic 26:208–11