Abstract
Context: Targeted delivery of drugs is still a therapeutic challenge and numerous methods have been reported for the same.
Objective: In this study, emphasis was placed on developing nanoparticles loaded with 5-fluorouracil (FU) and modifying the surface of the nanoparticles by conjugation with amino acid, to improve the distribution of 5-FU in the lungs.
Methods: An emulsion solvent evaporation technique was used to formulate nanoparticles of FU using Poly l-lactide and Pluronic F-68. The nanoparticles were conjugated with l-Cysteine using EDC as the activator of COOH group and were evaluated for product yield, particle size, surface morphology, amount of conjugation by Ellman’s method and in vitro drug release study.
Results and conclusion: The results indicated 60–65% yield with an average particle size of 242.7 ± 37.11 nm for the cysteine conjugated nanoparticle (CNP) formulation and more than 70% conjugation of cysteine. The cumulative percentage of drug released over a period of 24 h was found to be 58%. An increase in distribution of the delivery system in lungs (11.4% ID after 1 h) in mice was found indicating the role of l-Cysteine in the transport mechanism to the lungs. In vivo kinetic studies in rats revealed higher circulation time of CNP as compared to pure FU solution. The study helps in designing a colloidal delivery system for increased distribution of drugs to the lungs and may be helpful in delivery of drugs in conditions like non-small cell lung carcinomas.
Introduction
Formulation of drug delivery systems by the use of colloidal carriers offers dual benefits of controlled release and possibilities of targeting the drug to specific sites (Hagan et al., Citation1996). Poly(α-hydroxyacid)s, like poly l-lactic acid (PLA) have received high recognition as colloidal carriers over years due to (1) biodegradability of PLA under physiologic conditions leading to non-toxic, readily eliminated degradation products, (2) control over manufacturing to nano-sized particles which facilitates in evasing the phagocytic system (Moghimi et al., Citation2001; Eniola & Hammer, Citation2003).
The colloidal nanoparticles remain in circulation in high concentrations and offer potential targeting systems for site specific delivery (Davis, Citation1997). The distribution of nanoparticles within the body is mainly due to its relatively small size resulting in longer circulation times and its ability to penetrate through the microcapillaries (Kim, Citation2007).
A number of techniques for preparation of nanoparticles have been reported. Among the different methods, the solvent-evaporation technique has received particular attention due to its ease and good properties of formulated particles (El-Bagory et al., Citation2007).
Lung cancer, of all the cancers, stands to be leading cause of deaths worldwide, contributing to around 22% deaths in males and 14% in females (Silverberg et al., Citation1990). Tc99m labeled l-Cysteine (Tc99m-l-Cys) has been demonstrated to be useful in the evaluation of primary lung cancers (Tutus et al., Citation1996). The mechanism by which the Tc99m- l-Cys complex enters the tumor cells may be the interaction of the complex with serum mercaptalbumins that serve as carrier for the transport of the Tc99m-homocysteine to the tumor tissue and also the enlarged capillary permeability observed in the tumors (Takeda et al., Citation1998). Though, taken up in larger amounts in the kidney and bladder, the use of Tc99m- l-Cys, as a tumor imaging agent in the primary lesions of lung cancer was significant.
5-Fluorouracil (FU) is highly potent chemotherapeutic agent indicated for use in treatment of breast, colon and a little intrinsic activity against non-small cell lung cancer (NSCLC) (Lynch et al., Citation1993). It is a highly water-soluble drug, with a very short half-life (10–20 min). The short half-life and the lower circulation time of FU leads to higher doses (1000 mg/m2/d) of the drug in the synergistic combination therapy with the primary agents Cisplatin and etoposide.
In the present work, we focused on developing PLA nanoparticles containing FU, with an aim to improve the circulation time and thereby the half-life of FU and increasing its distribution and also modifying the surface of the nanoparticles with amino acid l-Cysteine (l-Cys) as a ligand capable of increasing the uptake of the drug in the lungs. The covalent attachment of l-Cys to the polymeric system, utilizing the amino group as the reaction center for linkage, may significantly contribute in increasing the affinity of l-Cys for the mercaptalbumin carriers thereby increasing the distribution of the drug in the lungs, eventually helping in decreasing the dose of FU in NSCLC. The nanoparticles were prepared by solvent-evaporation process and characterized in terms of size and zeta potential. Also, the tissue distribution (heart, liver, lung, kidney, spleen and brain) of the cysteine conjugated nanoparticles (CNP) was evaluated.
Methods
FU was obtained as a gift sample from Khandelwal Laboratiores, India; l-lactide was acquired from Alfa-aesar, GmBH. Pluronic F-68, Sodium hydrogen carbonate, Dichloromethane, Ellman’s reagent and mannitol were purchased from HiMedia Laboratories, Mumbai, India, EDC was procured from CDH laboratories, Mumbai, India.
Preparation of nanoparticles
Nanoparticles were prepared by modification of method previously reported elsewhere (Leo et al., Citation1998). PLA (50 mg) was dissolved in 5 mL of dichloromethane (DCM) and 1 mg of FU was dissolved separately in deionized water. The solution of FU was added under sonication to the PLA solution and the mixture was sonicated further for 3 min. The resulting primary water-in-oil emulsion was added slowly through fine syringe to a continuously sonicated 0.5% w/v aqueous solution of Pluronic F-68 over a period of 25 min. The water-in-oil-in-water emulsion obtained was stirred on a magnetic stirrer for 6 h at room temperature to remove DCM and complete the nanoparticle formation. The nanoparticles were washed with deionized water to remove any non-encapsulated drug, separated by ultracentrifugation (15 000 rpm, 4 °C), lyophilized using 0.1% w/v mannitol and stored in desiccator.
Conjugation of ligand to nanoparticles
Lyophilized nanoparticles (10 mg) were washed in 10 mL of activation buffer (mixed phosphate buffer, pH 5.5). The pellet was re-suspended with sonication in 10 mL of activation buffer and stirred on a magnetic stirrer for 15 min. To the resultant suspension, 20 mg EDC was added while mixing continuously at room temperature and was allowed to react for 20 min. The mixture was washed with two 10 mL portions of coupling buffer (pH 8.2) and re-suspended with sonication in 5 mL of the same.
A solution of l-Cys was freshly prepared in coupling buffer and a quantity equivalent to 300 µg was added to the activated nanoparticle suspension with continuous stirring for 5 h at room temperature.
The resulting suspension containing CNP was washed with storage buffer (PBS, pH 7.4), lyophilized and stored in desiccators ().
Characterization of nanoparticles
Yield
The yield of nanoparticles was calculated using the mass of final product after lyophilization with respect to the initial total mass of drug and polymer used to prepare the nanoparticles.
Encapsulation efficiency (Parikh et al., Citation2003)
Accurately weighed 25 mg nanoparticles were dissolved in 10 mL DCM. FU was extracted from the solution thrice using 25 mL normal saline by shaking in a separating funnel for 15 min and allowing equilibrating for 10 min before separating the aqueous portion. Absorbance of each aqueous extract was measured on UV spectrophotometer at 266 nm against normal saline as blank. Concentration of drug was calculated using the calibration curve. Encapsulation efficiency was calculated by the following formula:
where a – theoretical drug loading in mg
b – actual drug loading in mg
c – amount of drug in 25 mg of nanoparticles
d – total weight of drug and polymer in mg taken for nanoparticle formation
Surface morphology
Shape and surface morphology of the nanoparticles were examined by scanning electron microscopy (SEM). Dried nanoparticles were coated with thin gold–palladium layer using gold sputter module. The coated sample was scanned at an acceleration voltage of 15 kV.
Particle size, size distribution and zeta potential
The average particle size, poly dispersity index (PDI) and Zeta potential of the nanoparticles was determined using the dynamic light scattering technique on a Malvern Zeta sizer. The nanoparticles were suspended in ultrapure water in a disposable sizing cuvette and analysis was performed at an angle of detection of 90°.
Differential scanning calorimetry (DSC)
DSC was performed to study any possible interaction between the polymer and FU. Thermograms were obtained at a scanning rate of 10 °C min−1 over a temperature range of 25–330 °C.
Amount of coupling
The amount of l-Cysteine coupled to the nanoparticles was determined by Ellman’s test (Ellman, Citation1959). Briefly, 10 µL of cysteine-conjugated nanoparticle suspension was added to 990 µL of bis-dithionitrobenzoic acid (DTNB) working reagent (50 µL DTNB, 840 µL deionized water and 100 µL TRIS buffer), mixed properly and incubated for 5 min at room temperature. Optical density of the solution was measured at 412 nm and the amount of cysteine (measured as SH group) was calculated using ɛ = 13 600 by the following formula:
In vitro drug release
In vitro release of FU from the nanoparticles was performed by dialysis method adapted from Kostanksi & Deluca (Citation2000). FU-loaded nanoparticles (1 mg FU) were dispersed in 2 mL of phosphate buffer solution (PBS, pH 7.4) and filled in a pre-swollen dialysis membrane. The membrane was immersed in 30 mL of PBS pH 7.4 and mounted on a biological shaker at 37 °C. At determined time intervals, fixed volume of aliquots of sample was withdrawn and replaced with equal amount of fresh buffer. The aliquots were analyzed by HPLC using UV detector at 260 nm. Similar testing was done for release of pure FU directly from the dialysis membrane and release from CNP.
In vitro cytotoxicity
The cell growth inhibition activity of the CNP was evaluated by 3-(4,5-dimethylthiazol-2-yl)-2,5-diphenyl tetrazolium bromide (MTT) colorimetric assay method. Normal human lung fibroblast cells IMR-90 were re-suspended to a concentration of 1 × 104 cells/mL in fresh DMEM culture medium. The cells were seeded evenly into a 96-well plate at 1000 cells/well concentration and incubated for 24 h in a humidified atmosphere of 5% CO2 at 37 °C. Test solutions of 100 µg FU and CNP equivalent to 100 µg FU were added to each well and the plate was incubated for 72 h. After incubation, 10 mg/mL solution of MTT in PBS was added to each well and the plate was incubated for 4 h at 37 °C, allowing the viable cells to reduce the MTT into formazan crystals bearing purple color. The formazan crystals were dissolved by addition of 200 µL of DMSO. The absorbance of each well was measured at 560 nm using an ELISA microplate reader (Gajbhiye et al., Citation2013).
In vivo release and pharmacokinetic study
All the experiments were approved by the Institutional Animal Ethical Committee and were performed in accordance to the guidelines of the ethical committee. Healthy male albino rats (200–250 g) were used in the study. Animals were maintained on standard pellet diet with free access to water in a 12-h light and dark cycle. All the animals were fasted overnight with access to water ad libitum before dosing. Aqueous nanoparticle dispersion equivalent to 30 mg/kg of FU and pure drug solution of FU in saline were administered to rats by intravenous route in tail vein. Three rats per group were used for the study (Ceruti et al., Citation2000).
Blood samples (0.5 mL) were collected from retro orbital plexus of rat eye at 0, 1, 2, 4, 6, 8 and 24 h post administration of test compounds. The estimation of FU in plasma was performed by HPLC (Jin et al., Citation2005) using of C-18 column with PDA detector; wavelength of estimation was 205 nm and mobile phase consisting of water and acetonitrile (99:1) at a flow rate of 1.0 mL/min.
Blood samples were centrifuged at 1400 × g for 10 min to obtain serum. To a 200 µL of serum sample obtained from animals, 200 µL of distilled water and 4 mL ethyl acetate were added and the mixture was vortexed for 1 min and centrifuged at 13 000rpm for 20 min at 37 °C. The supernatant was evaporated to dryness under nitrogen atmosphere and the residue was reconstituted in 200 µL of ethanol. Twenty microliters of this solution was injected onto HPLC.
The pharmacokinetic parameters were determined by analyzing the data by Wagner–Nelson method. Clearance, half-life, area under curve (AUC0–24), volume of distributions and elimination rate constant were determined in blood samples.
Radiochemical synthesis of technetium-99m (99mTc) nanoparticles
Nanoparticles (NP & CNP) were labeled separately with 99mTc pertechnetate by direct labeling method as reported (Richardson et al., Citation1977). Briefly, freshly eluted 0.1 mL of 99mTc (185 MBq/mL) was mixed with 0.1 mL stannous chloride solution in 10% acetic acid and the pH of the solution was adjusted to 7.0 using sodium bicarbonate solution. To this solution, 0.1 mL of nanoparticle solution was added and incubated for 15 min at room temperature. The labeled formulations were stored in sealed vials for subsequent study.
Radiochemical purity of 99mTc nanoparticles
Radiolabeling efficiency was determined by ascending thin layer chromatography (ITLC) – silica gel-coated fiber plates (ITLC-SG) using 100% acetone as the developing solvent. A measured quantity (2–3 µL) of radiolabeled compound was applied at the bottom of the plate and was developed in acetone. The ITLC-SG strip was cut into small pieces of 0.5 cm and the gamma count of each piece was recorded by Caprac-R scintillation well counter to calculate the labeling efficiency in NP and CNP. The percent labeling efficiency was calculated by using the formula
Biodistribution studies
All the studies were performed in accordance with the institutional animal ethical committee guidelines and the protocol was approved by the IAEB. Balb-c mice of either sex weighing 20–25 g were divided in two groups of 3 animals each. Group I received NP as a single dose injection 30 mg/kg and group II received CNP at dose of 30 mg/kg body weight of animals; injected through the tail vein of animals. Three animals from each group were sacrificed after 15 min, 30 min and 1 h for collecting blood, liver, heart, kidney, spleen, lungs, stomach, intestine and brain. The amount of NP distributed in the tissues was estimated by determining the amount of radioactivity by gamma counter (Dubey et al., Citation2012). Briefly, each tissue obtained from the animals was weighed and subjected to gamma count by scintillation well counter and the percent injected dose of drug in tissue was established. The scintigraphic imaging of CNP in Ehlrich ascites tumor (EAT) bearing mice was also performed using GE Triumph system. The tumor was induced in the thigh of the mice in order to study the possible uptake of the delivery system in tumor.
Statistical analysis
All in vivo results are presented as mean ± SD (n = 3). Pearson correlation coefficients were used to evaluate the extent of relationship between two data sets. Statistical differences among groups were analyzed using t-test. In all cases, p < 0.05 was considered statistically significant.
Results and discussions
Conjugation of l-cysteine
Conjugation of the amino acid l-cys to nanoparticles was done by activating the carboxyl group of the polyester by EDC. An activated carboxyl group interacts more readily with amine group of amino acid leading to the formation of strong amide bonds. The conjugation was confirmed by the IR and NMR spectral analysis of model nanoparticles and CNP. The occurrence of peaks at 1643 (C = O, secondary amide), 1514 (NH deformation) and 3298 (NH stretching) in the CNP confirmed the coupling of the amino acid to the nanoparticle surface (). The NMR spectra of the CNP revealed peak at δ 5.1–5.4 confirming the formation of amide linkage (). The absence of the above peak in model nanoparticles further confirms the conjugation by formation of amide bonds.
Characterization of nanoparticles
The yield of the nanoparticles was found to be in the range of 60–65%. Average encapsulation efficiency, as calculated from the formula, was found to be 24.6 ± 0.42%. The low encapsulation efficiency can attributed due to the hydrophilic nature of the drug which limits its incorporation into the polyester core.
PLA nanoparticles were analyzed for particle size, size distribution and Zeta potential using Zetasizer. The average particle size obtained was 124.2 ± 23.11 nm with a PDI of 0.430 for model nanoparticles, 168.5 ± 29.97 nm with a PDI of 0.422 for FU-loaded nanoparticles. The nanoparticles displayed strong negative Zeta potential of −25.9 ± 4.71 mV for model nanoparticles and −17.3 ± 3.59 mV for FU-loaded particles. The negative surface charge of PLA nanoparticles at physiologic pH contributes to the formation of stable spherical particles. The PDI as obtained by DLS is shown ().
Figure 4. (a) Size distribution of the nanoparticles developed. (b) Zeta potential of the prepared nanoparticles. (c). Size distribution of the CNP.
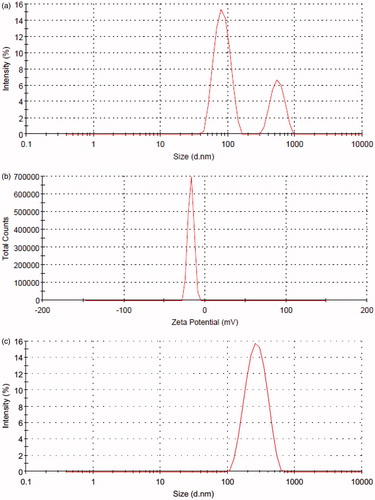
The surface morphology of formed nanoparticles was studied by SEM (). SEM photomicrographs revealed that the particles were spherical with a smooth surface without pores.
The average particle size for the CNP was found to be 242.7 ± 37.11 nm which was in accordance to our expectations (Nobs et al., Citation2003). The CNP retained the spherical shape and surface morphology of the FU nanoparticles.
Drug–polymer interactions were studied by DSC (). The thermograms of FU, physical mixture of FU and PLA and FU nanoparticles were studied. The occurrence of single sharp endothermic peak at around 280 °C corresponds to the melting transition temperature of FU. A slightly broadened peak was obtained for the physical mixture which could be explained as depression in melting point of FU due to the presence of PLA which exhibited melting transition temperature peak at around 150 °C. The absence of peak of FU in the nanoparticle confirms the uniform distribution of the drug throughout the core of the nanoparticles.
Amount of conjugation
The CNP were treated with DTNB working mixture to form a salt of polymer bound SH groups. The concentration of SH groups in this solution is determined colorimetrically. The results reflected a 72.1 ± 1.7% coupling of cysteine residues to the nanoparticles.
In vitro release profile and kinetics
The release of FU from nanoparticles was found to be prolonged up to a period of 24 h as compared to direct release of FU which was at peak with in 5 h and significantly dropped within 8 h. The initial burst release of FU from nanoparticles could be attributed to the presence of loosely entrapped drug on to the surface of the nanoparticles followed by a steady release due to slow diffusion of FU from the core of the nanoparticles. The burst release of drug was even less in the CNP (). The amount of FU released from CNP during the first 4 h was equivalent to 8.0% of the drug load of nanoparticles. After 24 h the cumulative amount of drug released was 57.8%.
In vitro cytotoxicity
The cytotoxicity of free FU and FU-loaded nanoparticles evaluated by the MTT assay method is represented in . It was observed that the CNP exhibited lower cytotoxicity as compared to plain FU solution. This is due to the presence of surface amino acid groups and thiol groups in the CNP which inhibit the direct interaction of the cells with CNP (Thasneem et al., Citation2011).
In vivo release and pharmacokinetic studies
CNP and pure drug solution of FU were subjected to pharmacokinetic analysis in rats. The serum drug concentration of FU solution and the l-cys conjugated nanoparticles is presented in . The pharmacokinetic parameters are summarized in . The values obtained for area under curve and the elimination half-life of FU nanoparticles were found to be twice than the pure drug solution. The clearance rate of the nanoparticles was half as compared to the same for pure drug. The CNP released FU at a steady state over the duration of 8 to 24 h whereas the FU from pure solution could not be detected in serum over the same period representing complete clearance of FU from circulation.
Table 1. Pharmacokinetic parameters of FU-loaded nanoparticles compared to pure drug.
Radiolabeling of NP and CNP
The labeling efficiency of NP and CNP with 99mTc was found to be more than 98% consistently. Technetium-99 m was used to label the nanoparticles directly using stannous chloride as the reducing agent. The reduced form of 99mTc makes it possible for labeling the surface of the nanoparticles efficiently.
Biodistribution studies
The normal tissue distribution of NP exhibits that the delivery system was recovered in major amounts in the reticuloendothelial (RES) organs (liver and spleen). A large amount of CNP was found to be distributed to lungs, heart and kidney. The presence of drug in the immune organs indicates rapid opsonization of the colloidal system, NP. The opsonization was found to be decreased in the CNP as evident from the lesser distribution of FU from CNP in liver and spleen, thus incorporating stealth properties in the system on conjugation with l-Cys.
Distribution of CNP in lungs (11.4 ± 0.8% ID at 1 h) was found to be higher as compared to NP (9.1 ± 1.6% ID at 1 h) (), which is a result of the conjugation with l-Cys (Tutus et al., Citation1996). As discussed earlier, the presence of mercaptalbumin may be responsible for this increased uptake of CNP by the lungs. Mercaptalbumin is the most common form of albumin, the most prominent plasma protein, present in serum (Suzuki et al., Citation1989). Albumin readily combines with the thiol (SH) groups of various amino acids like l-Cys, d-HCys, threonine to form mercaptoalbumin complex and hence mark their presence in the systemic circulation. The conjugated delivery system, CNP, as it contains free SH group of l-Cys, may have combined with albumin to form CNP-mercaptalbumin complex, which helps in the transport of the CNP to the lungs and decreasing its biotransformation thereby further increasing the circulation time of the complex. The presence of high quantity of CNP in blood even after 1 h of administration provides an idea of the formation of CNP-mercaptalbumin complex.
Figure 10. Concentration (%ID) of delivery system in tissue samples collected at 15 min after single dose of CNP or NP. N = 3. Mean ± SD. p < 0.05 versus NP.
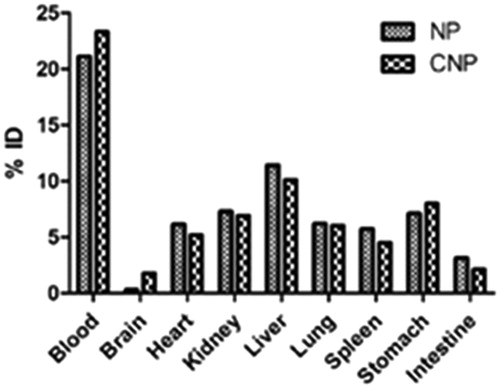
Figure 11. Concentration (%ID) of delivery system in tissue samples collected at 30 min after single dose of CNP or NP. N = 3. Mean ± SD. p < 0.05 versus NP.
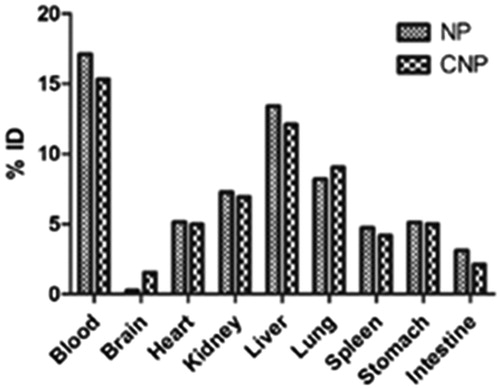
Figure 12. Concentration (%ID) of delivery system in tissue samples collected at 1 h after single dose of CNP or NP. N = 3. Mean ± SD. p < 0.05 versus NP.
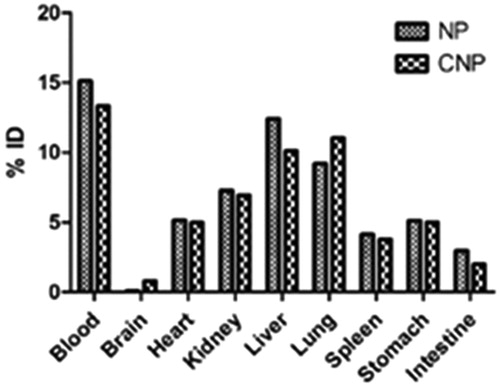
Scintigraphic images of 99mTc-labeled CNP in mice show rapid accumulation of radioactivity throughout the body with major accumulation in the thoracic region of animal at time interval of 1 h () post administration. The delivery system was also found to be accumulated in the tumor site of the animal.
Conclusion
A surface modification of polymeric nanoparticles with l-amino acid is utilized to improve the half-life and targeting efficiency of drugs. This approach proves to be effective in producing a significant sustained release of drug up to a period of 24 h with a lesser burst release effect. Tissue uptake of CNP was however time dependent. In mice, CNP showed noticeable increase in distribution to lungs as compared to NP. Based on these results, it can be concluded that the formulation developed in this work may be considered as an effective drug delivery for NSCLC.
Acknowledgements
The authors would like to thank Dr Anil Kumar Mishra, Scientist F, INMAS, New Delhi, India for providing the facilities for performing radiolabeling studies. One of the authors Mr Brijeshkunvar Mishra would also like thank Director, Technocrats Institute of Technology (Pharmacy), Bhopal, India for providing the facilities for in vitro cytotoxicity studies.
Declaration of interest
The authors report no declarations of interest.
References
- Ceruti M, Crosasso P, Brusa P, et al. (2000). Preparation, characterization, cytotoxicity and pharmacokinetics of liposomes containing water-soluble prodrugs of paclitaxel. J Control Release 63:141–53
- Davis SS. (1997). Biomedical applications of nanotechnology-implications for drug targeting and gene therapy. Trend Biotechnol 15:217–24
- Dubey N, Varshney R, Shukla J, et al. (2012). Synthesis and evaluation of biodegradable PCL/PEG nanoparticles for neuroendocrine tumor targeted delivery of somatostatin analog. Drug Del 19:132–42
- El-Bagory IM, Honsy EA, Suwayeh AA, et al. (2007). Effects of sphere size, polymer to drug ratio and plasticizer concentration on the release of theophylline from ethyl cellulose microspheres. Saudi Pharm J 15:213–7
- Ellman GL. (1959). Tissue sulfhydryl groups. Arch Biochem Biophys 82:70–7
- Eniola AO, Hammer DA. (2003). Artificial polymeric cells for targeted drug delivery. J Control Release 87:15–22
- Gajbhiye V, Ganesh N, Barve G, Jain NK. (2013). Synthesis, characterization and targeting potential of zodovudine loaded sialic acid conjugated-mannosylated poly(propyleneimine) dendrimers. E J Pharm Sci 48:668–79
- Hagan SA, Coombe AGA, Garnett MC, et al. (1996). S.S.Polylactide-poly(ethylene glycol) copolymers as drug delivery systems. 1. Characterization of water dispersible micelle-forming systems. Langmuir 12:2153–62
- Jin Y, Li J, Long-fu Rong, et al. (2005). Pharmacokinetics and tissue distribution of 5-fluorouracil encapsulated by galactosylceramide liposomes in mice. Acta Pharmacol Sin 26:250–6
- Kim KY. (2007). Nanotechnology platforms and physiological challenges for cancer therapeutics. Nanomed 3:103–10
- Kostanski JW, DeLuca P. (2000). A novel in vitro release technique for peptide containing biodegradable microspheres. AAPS PharmSciTech 1:30–40
- Leo E, Pecquet S, Rojas J, et al. (1998). Changing the pH of the external aqueous phase may modulate protein entrapment and delivery from poly(lactide-co-glycolide) microspheres prepared by w/o/w solvent evaporation method. J Microencaps 15:421–30
- Lynch TJ Jr, Kass F, Kalish LA, et al. (1993). Cisplatin, 5-Fluorouracil and etoposide for advanced non small cell lung cancer. Cancer 71:2953–7
- Moghimi SM, Hunter AC, Murray JC. (2001). Long-circulating and target-specific nanoparticles: theory to practice. Pharmacol Rev 53:283–318
- Nobs L, Buchegger F, Gurny R, Allemann E. (2003). Surface modification of poly (lactic acid) nanoparticles by covalent attachment of thiol groups by means of three methods. Int J Pharm 250:327–37
- Parikh RH, Parikh JR, Dubey RR, et al. (2003). Poly(D,L-lactide-co-glycolide) microspheres containing 5-fluorouracil: optimization of process parameters. AAPS PharmSciTech 4:1–8
- Richardson V, Jeyasingh K, Jewkes RF. (1977). Properties of 99mTc technetium- labeled liposomes in tumor bearing rat. Biochem Soc Trans 5:220–9
- Silverberg E, Boring CC, Squier TS. (1990). Cancer statistics. CA [A Cancer Journal for Clinicians] 40:9–26
- Suzuki KT, Karasawa A, Yamanaka K. (1989). Binding of copper to albumin and participation of cysteine in vivo and in vitro. Arch Biochem Biophys 273:572–7
- Takeda A, Goto R, Okada S. (1998). Mechanism of tumor transport of 99mTc-DL-Homocysteine, a possible tumor-imaging agent. Chem Pharm Bull 36:2570–5
- Thasneem YM, Sajeesh S, Sharma CP. (2011). Effect of thiol functionalization on hemo-compatibility of PLGA nanoparticles. J Biomed Mater Res A 99:607–17
- Tutus A, Nardali M, Gulmez I, et al. (1996). Evaluation of primary lung cancer with technetium-99m-l-Cysteine in comparison to thallium-201 uptake. Turkish J Nucl Med 5:33–9