Abstract
Effective oral insulin delivery has remained a challenge to the pharmaceutical industry. This study was designed to evaluate the effect of magnesium stearate on the properties of insulin-loaded Eudragit® RL 100 entrapped mucoadhesive microspheres. Microspheres containing Eudragit® RL 100, insulin, and varying concentrations of magnesium stearate (agglomeration-preventing agent) were prepared by emulsification-coacervation method and characterized with respect to differential scanning calorimetry (DSC), morphology, particle size, loading efficiency, mucoadhesive and micromeritics properties. The in vitro release of insulin from the microspheres was performed in simulated intestinal fluid (SIF, pH 7.2) while the in vivo hypoglycemic effect was investigated by monitoring the plasma glucose level of the alloxan-induced diabetic rats after oral administration. Stable, spherical, brownish, mucoadhesive, discrete and free flowing insulin-loaded microspheres were formed. While the average particle size and mucoadhesiveness of the microspheres increased with an increase in the proportion of magnesium stearate, loading efficiency generally decreased. After 12 h, microspheres prepared with Eudragit® RL 100: magnesium stearate ratios of 15:1, 15:2, 15:3 and 15:4 released 68.20 ± 1.57, 79.40 ± 1.52, 76.60 ± 1.93 and 70.00 ± 1.00 (%) of insulin, respectively. Reduction in the blood glucose level for the subcutaneously (sc) administered insulin was significantly (p ≤ 0.05) higher than for most of the formulations. However, the blood glucose reduction effect produced by the orally administered insulin-loaded microspheres prepared with four parts of magnesium stearate and fifteen parts of Eudragit® RL 100 after 12 h was equal to that produced by subcutaneously administered insulin solution. The results of this study can suggest that this carrier system could be an alternative for the delivery of insulin.
Introduction
Diabetes mellitus, elevated blood glucose associated with absence of insulin (type 1) or inadequate pancreatic insulin secretion (type 2), is a chronic disease characterized by disturbances in carbohydrate, protein and fat metabolism (Ahmed & Goldstein, Citation2006). In this disease condition, hyperglycaemia occurs because of uncontrolled hepatic glucose production by skeletal muscle with reduced glycogen synthesis. Clinical manifestations of diabetes mellitus include glycosuria, polyuria, polyphagia and polydipsia (Owens, Citation2002; Evans et al., Citation2011; Akhter & Nijhu, Citation2012). Diet and exercise plays important role in the control of blood sugar and overall management of diabetes. Pharmacotherapy of type 2 diabetes is initially dietary, although oral hypoglycaemic drugs usually become necessary, and about one-third of patients ultimately require insulin, a 51 amino acid peptide which is essential for the treatment of type 1 diabetes and in some type 2 diabetes (Giriraj & Giriraj, Citation2003; Gowthamarajan & Kulkarni, Citation2003; Morishita & Peppas, Citation2006; Pamnani, Citation2008). Insulin is administered parenterally by subcutaneous injection (Beals & Kovach, Citation1997), which results in low level of compliance due to pain as well as complications due to multiple jabs, hence the need for an alternative less invasive but effective method of insulin administration (Gowthamarajan & Kulkarni, Citation2003). The oral route is considered to be the most convenient, acceptable and desired route of drug delivery which will help eliminate the pain caused by injection, psychological barrier associated with multiple daily injection and possible infections (Kisel et al., Citation2001; Kim & Peppas, Citation2003; Whitehead et al., Citation2004; Cui et al., Citation2006; Sarmento et al., Citation2007). Oral delivery of insulin as a non-invasive therapy for diabetes mellitus is still a challenge to the drug delivery technology, due to low oral bioavailability, lack of lipophility, poor permeability across intestinal epithelium because of insulin high molecular weight as well as digestion by proteolytic enzymes in the luminal cavity (Tozaki, Citation2001; Gowthamarajan & Kulkarni, Citation2003; Tiyaboonchai, Citation2003; Krauland et al., Citation2004; Li & Deng, Citation2004; Nakamura et al., Citation2004; Toorisaka et al., Citation2005; Sajeesh & Sharma, Citation2006; Sarmento, Citation2006; Tuesca & Lowman, Citation2006; Lin, Citation2007; Simon, Citation2007).
In developing oral insulin delivery systems with high bioavailability, several attempts have been explored to optimize insulin stability and absorption within the GIT (Chandler et al., Citation1994; Damge et al., Citation1995; Sarciaux et al., Citation1995; Iwanaga et al., Citation1997). Various approaches that could be adopted include modification of physicochemical properties such as lipophilicity and enzyme susceptibility, addition of novel function to macromolecules as well as the use of improved carrier system. In other words, methods like protecting insulin from enzymatic degradation, use of penetration enhancers, chemical modification, bioadhesive delivery system, as well as use of carrier systems could help to improve bioavailability of insulin (Heinemann et al., Citation2001; Rosenstock, Citation2001; Dorkoosh, Citation2002; Eaimtrakarn et al., Citation2002; Heinemann, Citation2002; Cefalu, Citation2004; Chung, Citation2004; Attivi, Citation2005; DeFronzo et al., Citation2005; Jain et al., Citation2005; Kavimandan, Citation2006; Chalasani, Citation2007; Cui, Citation2007; Damge et al., Citation2007; Ichikawa & Fukumori, Citation2007). The successful production of an effective oral insulin formulation will depend on the development of an appropriate drug delivery (carrier) system that will protect insulin from the degradative effect of enzymes and pH of the GIT (Caliceti & Veronese, Citation1999). The use of carrier systems, such as liposomes, mixed micelles, multiple emulsions, microemulsions, microspheres, hydrogels, azopolymer coating, site specific drug delivery systems, absorption enhancers, enzyme inhibitors and modifications of chemical structure have been shown to improve the gastrointestinal absorption of peptide drugs (Yamamoto et al., Citation1994; Tozaki et al., Citation1997; Chetty & Chien, Citation1998; Caliceti & Veronese, Citation1999; Kisel et al., Citation2001).
A microparticulate drug delivery system is a colloidal carrier system. In terms of formulation strategies, microspheres provide benefits such as rapid emptying from the stomach as well as more reproducible transit through the small intestine and colon. Their increased surface area facilitates rapid drug release and more reproducible absorption than conventional dosage forms (Nilkumhang & Basit, Citation2009). Further advantages of microspheres include effective protection of encapsulated drugs against degradation, increased drug solubility, reduced adverse or toxic effects, site-specific drug delivery and controlled drug release (Singh et al., Citation2010). Microspheres based on Eudragits® have been explored for various drug delivery applications (Agyilirah & Banker, Citation2000; Haznedar & Dortunc, Citation2004; Sahoo et al., Citation2009; Apurba et al., Citation2009; Kishori et al., Citation2013), including insulin delivery (Kinesh et al., Citation2010; Singh et al., Citation2010b; Zhang et al., Citation2011; Cárdenas-Bailón et al., Citation2013). Eudragit® polymers are more favored in the formulation of pH-sensitive drug molecules. The obvious advantages of these polymers include pH-dependent release profiles, encapsulation of high amount of drug, release of the incorporated drug in controlled manner and high level of stability (Kishori et al., Citation2013). Generally, Eudragits® are copolymers of acrylic and methacrylic acid esters with quaternary ammonium groups. The ammonium groups are present as salts and make the polymers permeable (Haznedar & Dortunc, Citation2004). Eudragit® RL is a low water permeable, cationic, non-biodegradable polymer commonly used for the preparation of controlled-release dosage forms, and represents a good material for the dispersion of drugs (Sahoo et al., Citation2009). It is composed of poly (ethylacrylate-methylmethacrylate-trimethylammonioethyl methacrylate chloride) copolymers with ratios 1:2:0.1 containing a low level of quaternary ammonium groups in 4.5-6.6%. Eudragit® RL exhibits a very low permeability, enabling sustained release formulation manufacture; has well-established mucoadhesive characteristics (Zhang et al., Citation2012b; Ofokansi et al., Citation2014); is insoluble in aqueous media but swells in water. It is inert and permeable and exhibits pH-independent release profiles. Controlled-release formulations have many advantages over immediate release formulations. With these formulations, less frequent drug administration is possible, lower plasma peak concentrations can be obtained to avoid adverse effects, and patient compliance can correspondingly be improved (Agyilirah & Banker, Citation2000; Apurba et al., Citation2009).
The successful formulation of stable and effective solid dosage forms depends on the careful selection of the excipients which are added to facilitate administration, promote the consistent release and bioavailability of the drug and protect it from degradation (Zhao & Augsburger, Citation2005). Magnesium stearate has become one of the most widely used lubricants owing to its superior lubrication properties and is also a droplet stabilizer (Yüksel & Baykara, Citation1997; Rosa et al., Citation2000; Hogan, Citation2008). The novelty of the work lies on the use of Eudragit® RL 100 and magnesium stearate (a hydrophobic droplet stabilizer) in combination to improve the controlled release effect of insulin-loaded Eudragit® entrapped microspheres prepared by the emulsification-coacervation technique. Emulsion-based formulations of insulin (Morishita et al., Citation1998; Shima et al., 2006), including microspheres prepared by novel emulsification technique (Liu et al., Citation2006) have been used to control the release of insulin. Emulsification enhances the fineness and droplet size of microspheres (Liu et al., Citation2006) while magnesium stearate stabilizes the droplet sizes of microspheres (Cárdenas-Bailón et al., Citation2013). In this study, liquid paraffin and acetone were used for the preparation of microspheres. Magnesium stearate was used as a droplet stabilizer to prevent droplet coalescence in the oil medium and n-hexane was added as a non-solvent to the processing medium to solidify the microspheres. The rationale for magnesium stearate ratio is that, as magnesium stearate is a droplet stabilizer, this stabilizing effect was assessed by increasing the concentration of the stabilizer so as to find out the effect it will have on the controlled release and mucoadhesion of insulin-loaded Eudragit® entrapped microspheres. The prepared microspheres were evaluated for production yield, loading efficiency, morphology, particle size, thermal, mucoadhesive and micromeritic properties, and in vitro drug release. The anti-diabetic property of the formulations was also evaluated in alloxan-induced rat model.
The objective of this study was to evaluate insulin-loaded mucoadhesive Eudragit® RL100 microspheres prepared by emulsification-coacervation method for possible oral delivery of insulin, in order to achieve a controlled release and reduced enzymatic degradation by proteolytic enzymes and low absorption rates of the drug.
Experimental
Materials
The materials used include methacrylic acid copolymer (Eudragit® RL 100) (Evonik Degussa, India), sorbitan monostearate (Span 60, Merck, Germany), Liquid paraffin (Moko Pharm. Ltd., Nigeria), magnesium stearate, n-hexane, acetonitrile and perchloric acid (BDH, England), potassium dihydrogen phosphate (monobasic potassium phosphate), sodium hydroxide, concentrated hydrochloric acid and acetone (Sigma-Aldrich, New York, USA), distilled water (freshly prepared in Biochemistry lab, UNN), insulin (Humulin 70/30, Lilly, Egypt). These materials were used as procured from the manufacturers without further purification. All other reagents were analytical grade and used as such. The animal experiments complied with the regulations of the Committee on Ethics on the Use of Laboratory Animals of the University of Nigeria in accordance with the Federation of European Laboratory Animal Science Association and the European Community Council Directive of November 24, 1986 (86/609/EEC).
Formulation of insulin-loaded microspheres
Insulin microspheres were prepared based on oil-in-oil emulsification-coacervation method using Eudragit® RL 100 polymer. The polymer was dissolved in 12.5 ml of acetone contained in a 250 ml beaker with stirring at room temperature. Thereafter, 0.4 ml of 100 International Unit (IU) per ml insulin and magnesium stearate (0.1 g) were dispersed in the polymer solution. The resulting milky white dispersion was added drop wise into a beaker containing a mixture of liquid paraffin (50 ml) and Span 60 (0.5 g) and homogenized using a paddle stirrer (Remi Instruments, Mumbai, India) at 500 rpm for 2 h. The resulting microspheres were filtered using filter paper (Whatman NO.1) and washed severally with n-hexane until they were completely free of oil. The microspheres were dried at room temperature and stored at 4 °C until used. Four batches of drug-loaded microspheres and a control (unloaded microspheres) were prepared as shown below in .
Table 1. Formulation compositions of the microspheres.
Determination of percentage yield
The formed microspheres were recovered and weighed accurately. The yield of microspheres was determined by comparing the whole weight of formed microspheres against the combined weight of the copolymer and drug using the equation below:
(1)
Thermal analysis
Briefly, the thermal properties of insulin, Eudragit® RL 100 and drug-loaded microspheres were studied using a differential scanning calorimeter (DSC 204 F1, Netzch, Germany) to evaluate any possible drug-polymer interaction. The analysis was performed at a heating rate of 10 °C/min from 10 °C to 400 °C temperature range under an inert nitrogen atmosphere with a flow rate of 20 ml/min.
Quantitative determination of insulin
The insulin content of the microspheres was determined using a high performance liquid chromatography (HPLC). The machine consisted of an Agilent 1100 series programmable separating module, quaternary pump G 1311 A (Agilent technology, Geneva, Switzerland), an auto degasser G1322A, and a variable wavelength detector G1314A (Marinfield, Germany). The column was a reverse phase ODS (C-18, 5 µm 4.6 × 250 mm, Supelcosol, Mumbai, India) equipped with a guard. The mobile phase consisted of acetonitrile and water (10:90), perchloric acid was used to adjust the pH to 3. The flow was set at 0.8 ml/min and the chromatograms recorded at 280 nm.
Insulin loading efficiency
A 10 mg quantity of microspheres was dispersed in 10 ml of simulated intestinal fluid (SIF, pH 7.2). The dispersion was allowed to stand for 2 h after which it was mixed with a vortex mixer for 5 min and then centrifuged at 4000 rpm for 10 min. The amount of insulin contained in each batch of the formulations was determined by the HPLC method (Builders et al., Citation2008b). The drug loading efficiency was then determined by evaluating with Equation Equation2(2) (Kim & Peppas, Citation2003; Cui et al., Citation2006; Sarmento et al., Citation2007).
(2)
where ILE is insulin loading efficiency, AD is actual amount of insulin in microspheres and TD is the theoretical amount of insulin in microspheres.
Morphology and particle size analysis
The size and morphology of the microspheres were analyzed by computerized image analysis using samples mounted on a glass slide (Marinfield, Germany). These samples were dispersed in little quantity of liquid paraffin and smeared on the slide using a glass rod. It was then covered with a cover slip and viewed with a photomicroscope (Hund®, Weltzlar, Germany) attached with a digital camera at a magnification of 1000×. With the aid of the software in the photomicroscope, the particle morphologies were observed and photomicrographs taken. The sizes of the particles were measured (n = 30) and average taken.
Mucoadhesiveness of the microspheres
The mucoadhesive properties of the microspheres were evaluated by the in vitro wash-off test as reported by Ofokansi & Adikwu (Citation2007). The apparatus used for this study was designed to give reproducible results. A 200 mg quantity of the microspheres was weighed accurately and placed on an 8.5 cm long bovine ileum and allowed to interact with and adhere to the surface of the ileum. A 50 ml portion of simulated intestinal fluid was poured into a separating funnel, clamped to a retort stand, and allowed to run over the microspheres on the bovine ileum. The microspheres that detached from the ileum were collected, dried and weighed. This was repeated for all the batches. The percentage mucoadhesion for each batch was calculated using the formula below:
(3)
Micromeritic properties of the microspheres
The flow properties of the microspheres were investigated by measuring the bulk density, tapped density, Carr’s index and Hausner’s ratio using standard methods (Haznedar & Dortunc, Citation2004; Jain et al., Citation2005; Kishori et al., Citation2013).
In vitro release of insulin from microspheres
Insulin release from the microspheres was evaluated in simulated intestinal fluid (SIF, pH 7.2) at 37 ± 1 °C using an established method based on magnetic stirrer plate (Ofokansi & Adikwu, Citation2007). The polycarbonate dialysis membrane (pore size 0.22 µm) used as release barrier was pre-treated by soaking it in the dissolution medium for 24 h prior to the commencement of each release experiment. In each case, approximately 100 mg microspheres were placed in dialysis membrane containing 5 ml release medium, suspended in a 250 ml beaker containing 150 ml of SIF and tied with paddle of dissolution apparatus. Agitation of the fluid system (100 rpm) was done with a magnetic stirrer (Remi Instruments, Mumbai, India). At predetermined time intervals, 5 ml samples were withdrawn and replaced with SIF. The insulin content of the withdrawn samples was determined using HPLC quantitative method and the release profiles plotted.
Kinetic analysis of in vitro release profiles
The dissolution data for the microspheres were analyzed to determine the in vitro release kinetic models and mechanisms. Four kinetic models including the zero-order, first-order, Higuchi square root and Hixson-Crowell cube root models were applied to process the release data to find out the equation with the best fit (Yüksel & Baykara, Citation1997; Rosa et al., Citation2000; Zhao & Augsburger, Citation2005; Hogan, Citation2008).
(4)
(5)
(6)
(7)
where Q is the release percentage at time, t. K1, K2, K3, and K4 are the rate constants of zero-order, first-order, Higuchi and Hixson-Crowell models, respectively.
Pharmacodynamic studies
Induction of diabetes
Rats weighing between 180–280 g were purchased from the Department of Biochemistry, University of Nigeria, Nsukka. The rats were all kept in standard and conditioned animal cages and left for one week to acclimatize to the new laboratory environment while being fed with standard laboratory diet. Diabetes was induced by intravenous injection of alloxan dissolved in normal saline through the marginal ear vein at a dose of 120 mg/kg (Whitehead et al., Citation2004; Sarmento et al., Citation2007). After 3–5 days of the alloxan treatment, rats with frequent urination, loss of weight, and blood glucose levels higher than 120 mg/dL were considered diabetic and selected for the study. The rats were monitored for persistent blood glucose elevation for 5 days (Tozaki, Citation2001; Tuesca & Lowman, Citation2006). Before testing, animals were fasted overnight with free access to water.
In vivo anti-diabetic study
The anti-diabetic study was based on real oral administration of the microspheres encapsulated in hard gelatin capsules (Builders et al., Citation2008b). Fourty Wistar rats (either sex) were used for the evaluation of the anti-diabetic effects of the formulations. In each case, the animals were fasted for 12 h prior to oral drug administration. Rats were divided into eight groups of five animals each and each group of animals was housed in a separate cage. The different formulations of the insulin-loaded microspheres were put into hard gelatin capsules (300 mg capacity), with each capsule containing microspheres equivalent to insulin dose of 50 IU/kg body weight for each animal. The capsules were administered orally to the animals in groups 1 to 4 (groups that received insulin-loaded microspheres batches C1 to C4) according to their weight. The fifth group received unloaded microspheres; the sixth group received insulin solution (50 IU/kg) orally; the seventh group received distilled water orally; while the eightieth group received insulin solution (50 IU/kg) subcutaneously (Builders et al., Citation2008b). In order to evaluate the effect of administration on hyperglycemia, distilled water, insulin solution and unloaded microspheres (encapsulated in hard gelatin capsule) were orally administered as negative controls, while subcutaneous injection of insulin was given as positive control. Blood samples were withdrawn from the tail vein at predetermined intervals of 0, 0.5, 1, 2, 4, 6, 8, 10 and 12 h, and blood glucose levels were measured using Accu-check glucometer. (Switzerland). Food and water intake as well as urine output of the animals were measured and monitored in the course of the study.
Statistical analysis
All experiments were performed in replicates for validity of statistical analysis. Results were expressed as mean ± SD. ANOVA and student’s t test were performed on the data sets generated using SPSS. Differences were considered significant for p values ≤0.05.
Results and discussion
Percentage yield of the microspheres
The percentage yield of the microspheres was high and ranged from 55.48 to 83.97% (), an indication that the formulation technique adopted was reliable. There was no evidence of correlation between the proportion of droplet stabilizer used in the formulation of the microspheres and the microspheres yield. The percentage loss recorded could be attributed to losses during the filtration, transferring or drying.
Table 2. Some physicochemical and physico-technical properties of the microspheres.
Thermal properties
shows the thermograms of insulin and the microspheres while presents the thermal properties of insulin and the formulations. DSC result of insulin showed a melting peak of 125 °C with an enthalpy of −132 mW/mg (). The unloaded microspheres (C0) showed two melting peaks (62.7 and 78.2 °C) with corresponding enthalpies of −4.216 and −4.131 mW/mg. The DSC thermograms of insulin-loaded microspheres showed different melting peaks and thermal properties, as depicted in . The results showed that all the drug-loaded microspheres showed two melting peaks [62.5 and 75.7 °C (batch C1); 62.3 and 79.0 °C (batch C2); 63.6 and 104.6 °C (batch C3); 63.8 and 78.1 °C (batch C4)] with corresponding enthalpies of −3.767 and −4.720 mW/mg (batch C1), −4.437 and −4.007 mW/mg (batch C2), −5.452 and 3.213 mW/mg (batch C3), and −1.785 and −2.637 mW/mg (batch C4). Since higher melting point values indicate more ordered crystal structures (Caliceti & Veronese, Citation1999; Kavimandan, Citation2006; Chalasani, Citation2007), it follows that the formulations are less crystalline than insulin and that the drug is solubilized in the microspheres. More so, the physicochemical compatibility of the drug and the polymer studied by differential scanning calorimetry suggested absence of any incompatibility. The results revealed the compatibility of insulin and the polymer (Eudragit® RL 100) as well as the stability of the drug in the microspheres. This was because the formulations (drug-loaded microspheres) gave lower melting point values than insulin, implying that insulin existed in amorphous state in the formulations and also was properly solubilized in the microspheres (Rosa et al., Citation2000; Jain et al., Citation2005).
Figure 1A. DSC thermograms of (a) insulin (b) unloaded Eudragit® RL 100 microspheres (batch C0) (c) drug-loaded microspheres batch C1 (d) batch C2 (e) batch C3 (f) batch C4. C1–C4 are insulin-loaded microspheres containing increasing quantities of magnesium stearate (0.1, 0.2, 0.3 and 0.4 g, respectively) while C0 is the unloaded microspheres.
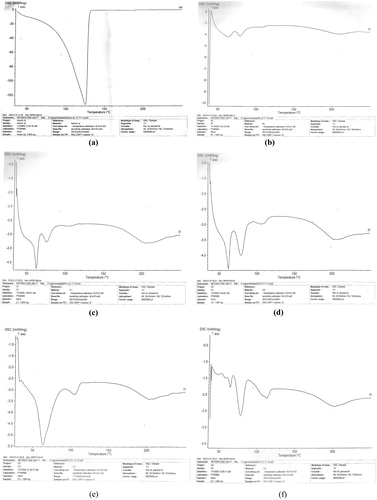
Figure 1B. Overlay of DSC thermograms of the formulations. C1–C4 are insulin-loaded microspheres containing increasing quantities of magnesium stearate (0.1, 0.2, 0.3 and 0.4 g, respectively) while RL is the unloaded microspheres.
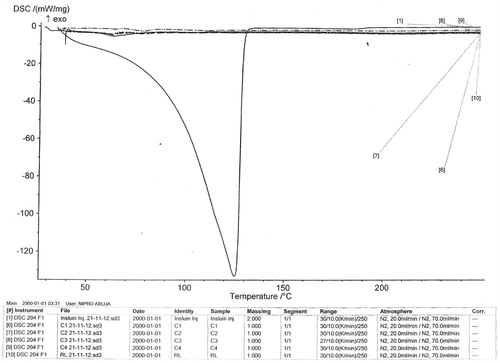
Table 3. Thermal properties of the formulations.
Loading efficiency
The drug-loading efficiency is an important variable for assessing the drug-loading capacity of microspheres and their drug release profiles, thus suggesting the amount of drug that would be available at the site of absorption. This parameter is dependent on the process of preparation, physicochemical properties of drug and formulation variables (Jain et al., Citation2005; Ichikawa & Fukumori, Citation2007). It is also highly influenced by type of polymer, polymer concentration and solvent used to dissolve the drug and polymer (Caliceti & Veronese, Citation1999). The drug loading efficiency was observed to be dependent on the proportion of magnesium stearate (droplet stabilizer) used in preparing the microspheres. shows that drug loading efficiency generally decreased with increasing amount of magnesium stearate in the formulation. Thus, microspheres prepared with least amount of magnesium stearate (100 mg) entrapped the highest amount of insulin, whereas microspheres formulated with highest amount of magnesium stearate (400 mg) entrapped the least amount of insulin in comparison with the rest of the microspheres batches.
Morphology and particle size
shows the mean particle sizes of the various batches of the microspheres. The mean particle size (n = 30) of insulin-loaded microspheres ranged from 30.50 ± 1.90 µm to 42.70 ± 2.30 µm whereas the mean particle size of unloaded microspheres batch was 26.0 ± 1.40 µm. Thus plain microspheres had the smallest mean particle size while insulin-loaded microspheres containing highest amount of magnesium stearate (400 mg) possessed the largest mean particle size. The photomicrographs of the microspheres () indicate that discrete, spherical, brownish and free flowing microspheres were obtained. The sizes of the microspheres were all within the micrometer range, indicating that the production process was able to achieve the intended end-point, consistent with previous reports (Rosa et al., Citation2000; Jain et al., Citation2005). It is discernible from that the average size of the microspheres increased with an increase in the proportion of the droplet stabilizer employed. Particle size of microspheres is an important parameter, since it affects drug release and pharmacokinetics (Yüksel & Baykara, Citation1997). For microspheres engineered for parenteral administration, large particles would find it difficult to pass through the syringe. However, the microspheres evaluated in this study are intended for oral administration and particle size will influence only the rate of drug release and subsequent pharmacokinetics (Ofokansi & Adikwu, Citation2007).
Mucoadhesiveness of the microspheres
also shows the results of the mucoadhesion of the microspheres to cow everted intestinal tissue as evaluated in SIF. It is evident from the table that the microspheres formulated showed good mucoadhesive properties and exhibited percentage mucoadhesion as high as 73.0% for unloaded microspheres and between 70.0 and 90.0% for insulin-loaded microspheres. The mucoadhesive property of unloaded microspheres was however lower than that of drug-loaded microspheres. The percentage mucoadhesion of the different batches of the insulin-loaded microspheres were found to increase with increase in the concentration of magnesium stearate, with batch C4 containing the highest amount of magnesium stearate (400 mg) showing the highest mucoadhesion in SIF. It implies that magnesium stearate potentiated the mucoadhesiveness of insulin-loaded Eudragit® entrapped microspheres in SIF. The high percentage mucoadhesion of the different batches of the microspheres in SIF signifies high mucoadhesive property in intestinal condition where insulin absorption takes place (Yamamoto et al., Citation1994; Chetty & Chien, Citation1998; Rosenstock, Citation2001; Dorkoosh, Citation2002; Eaimtrakarn et al., Citation2002; Cefalu, Citation2004; Chung, Citation2004; Attivi, Citation2005; DeFronzo et al., Citation2005; Ahmed & Goldstein, Citation2006; Chalasani, Citation2007; Cui, Citation2007; Damage et al., Citation2007; Evans et al., Citation2011; Akhter & Nijhu, Citation2012). This shows that the insulin-loaded microspheres will have a prolonged release and thereby enhance bioavailability of insulin, consistent with previous reports (Rosa et al., Citation2000; Jain et al., Citation2005; Builders et al., Citation2008a). The quaternary ammonium groups of Eudragit® RL 100 confer a positive zeta potential to microspheres which can interact with the negative charges of intestinal mucus (due to the presence of sialic acid in its composition) (Zhang et al., Citation2012b; Ofokansi et al., Citation2014). These interactions could be responsible for mucoadhesion of microspheres on the surface of the intestinal barrier, allowing a closer intimacy of contact between drug and mucous membrane at the absorption sites, thus enhancing the permeability as well as reducing the local degradation of the drug (Agyilirah & Banker, Citation2000; Haznedar & Dortunc, Citation2004; Sahoo et al., Citation2009; Apurba et al., Citation2009; Kishori et al., Citation2013). In other words, the mucoadhesive results indicate that the microspheres may be preferable as carriers for drugs such as insulin targeted to have drug residence time in the small intestine. By implication, the microspheres formulations may be a novel oral controlled drug delivery system for the delivery of insulin to the intestine owing to its high mucoadhesiveness in SIF.
Micromeritic properties of the microspheres
The consideration of the particle properties of microspheres intended for application is of critical importance. This is because important processes such as mixing, flow and compression are procedures that are dependent on particle properties (Haznedar & Dortunc, Citation2004; Singh et al., Citation2010; Kishori et al., Citation2013). The Hausner’s quotient and Carr’s compressibility index are some of the fundamental parameters from which certain important powder properties especially flow can be predicted. shows the values for bulk and tapped densities, Hausner’s quotient and Carr’s compressibility index for the various batches of the microspheres. These parameters are primarily dependent on the particle size distribution, shape and tendency of the particles to adhere to one another. The bulk and tapped densities of the formulations have values less than 1 g/ml while the Carr’s index values ranged from 18.75 ± 1.95% to 34.08 ± 2.10%, Hausner’s quotient ranged from 1.23 ± 0.11 to 1.52 ± 0.10. Hausner’s quotient values less than 1.25 have been reported to indicate good flow characteristics for a powder (Agyilirah & Banker, Citation2000; Zhao & Augsburger, Citation2005; Sahoo et al., Citation2009; Apurba et al., Citation2009). The HQ values obtained for the microspheres indicate that the microspheres generally exhibited fair to poor flow-ability. Furthermore, the percentage compressibilities or Carr’s indices of the various batches of the microspheres are presented in . Theoretically, the less compressible a material is the more flowable it would be. A value of 5–15% is indicative of excellent flow, 12–16% is good flow and 18–21% is fair flow (Builders et al., Citation2008b; Builders et al., Citation2009). Thus, it could be evaluated from the values obtained from the compressibility index that the microspheres have a generally fair to poor flow in line with the compressibility indices results. It is discernible from the micromeritics properties that the flow property increases from poor flowability to fair flowability with increased concentration of magnesium stearate (lubricant and droplet stabilizer) (Yüksel & Baykara, Citation1997; Hogan, Citation2008). In other words, the microspheres showed poor-fair flow properties as magnesium stearate in the preparation increases from batch C1 to C4.
In vitro release studies
The in vitro release profiles of insulin in SIF (pH 7.2) was high, indicating significant release of insulin from all batches of the formulation as shown in . Batch C2 formulation gave the maximum release of 79.4%, while batch C1 gave the least (68.2%) and batches C3 and C4 released 76.6% and 70.0% of insulin respectively at 12 h. There was insignificant difference (p ≥ 0.05) in the release profiles of the various batches of the microspheres. The drug concentration and the carrier are some of the main factors affecting drug release (Tozaki et al., Citation1997). Drug release from microspheres should theoretically be slower as the concentration of polymer is increased because of an increase in the path length through which the drug has to diffuse (Tozaki et al., Citation1997). However, results obtained indicate that increase in the concentration of magnesium stearate caused an increase and thereafter a decrease in the drug release rate from the microspheres formulations. Since uniform dose of insulin (0.4 units of 100 IU/ml of insulin) as well as uniform quantity of Eudragit® (1.5 g) was loaded into the microspheres, varying insulin release rates observed in the formulations could be attributed to variation in the concentration of magnesium stearate. The initial increased rate of insulin release with increased magnesium stearate could be attributed to burst effect caused by the leaching out of the unentrapped drug adhering to the surface of the microspheres after the initial rapid hydration and swelling; thereafter, because of an increase in the path length through which the drug has to diffuse, the rate of insulin release became slower with further increase in the concentration of magnesium stearate (Tozaki et al., Citation1997). The release profile of insulin from the microspheres was investigated in SIF. This medium was used to simulate the intestinal environment to aid in the prediction of the release profile of the various formulations of the drugs in the small intestine (Builders et al., Citation2008a). A characteristic feature of the release profile of insulin from the various batches of the microspheres in SIF is the biphasic pattern of release. The rapid release of insulin from the microspheres within the first 60 min is possibly due to a burst effect caused by the leaching out of the unentrapped drug adhering to the surface of the microspheres after the initial rapid hydration and swelling. Burst release resulting in biphasic release pattern may be utilized in therapeutic design of dosage forms. This has been observed in microparticles prepared using varying concentrations of magnesium stearate (Yüksel & Baykara, Citation1997; Rosa et al., Citation2000; Hogan, Citation2008; Nilkumhang & Basit, Citation2009). There is a lot of peripheral attachment of the drug as a result of expulsion during microspheres drying (elastic contraction as seen in gels) or drug migration as a result of solvent drag during drying. In other words, the amounts of insulin released as a result of burst effect may likely represent the amounts that adhered weakly to the surface of the formulated microspheres. The remaining amounts which were released in a more gradual pattern most likely represented the amounts that were entrapped into the core (matrix) of the microspheres. This may be an advantage because it would lead to a high initial blood concentration of the drug and a gradual release of the remaining drug. The microspheres had the tendency to fully sustain the release of insulin, as had been demonstrated for insulin microspheres in previous studies (Rosa et al., Citation2000; Jain et al., Citation2005). The high and rapid release of insulin from the microspheres in SIF, in addition to the burst effect, may also be a result of the high rate of hydration and swelling of the microspheres in SIF, which, in turn, could be attributable to the properties of the excipients used in preparing the microspheres. The subsequent slow release phase could be a consequence of the decreasing residual amount of drug in the microspheres and the build-up of drug concentration in the dissolution medium in the course of time. This indicates that once the drug adhering to the microspheric surface has leached, the drug release becomes diffusion-controlled (Builders et al., Citation2008a). Moreover, Eudragit® RL 100 possesses bioadhesive properties (Agyilirah & Banker, Citation2000; Haznedar & Dortunc, Citation2004; Sahoo et al., Citation2009; Apurba et al., Citation2009; Zhang et al., Citation2012b; Kishori et al., Citation2013; Ofokansi et al., Citation2014). This is an added advantage since the transit time of the dosage form would be prolonged in the small intestine for maximum absorption of the active ingredient.
In vitro release kinetics and mechanisms
Different mathematical models were used to describe the kinetics of insulin release from the microspheres. The criterion for selecting the most appropriate model was chosen on the basis of goodness-of-fit test. The result is presented in . A comparative evaluation of the (r2) values for the microspheres shows that all the formulae exhibited the highest regression coefficient when the percentage of the drug released was plotted against the square root of time. These data revealed that the Higuchi square root and Hixson-Crowell models were the predominant models of drug release from the polymeric matrix. These results conform to previous reports on insulin microspheres (Rosa et al., Citation2000; Jain et al., Citation2005). Higuchi’s square root model primarily describes drug release from a heterogeneous hydrated or solvated matrix where the drug is dispersed uniformly and it aims to describe the drug release by a combination of dissolution and diffusion out of the polymer matrix Tozaki, Citation2001; Sarmento, Citation2006; Tuesca & Lowman, Citation2006). The values () show that all the batches of the microspheres obeyed the models and thus exhibited diffusion-controlled release. Although the matrix was not soluble in water, the ability to hydrate in the medium paved way for the drug to diffuse due to structural disorientation that led to the formation of pores through which the drug is eventually released in a controlled manner. Differences in the ratios of the polymer used in the formulation accounted for the variation in the kinetic results obtained.
Table 4. Kinetics of release of insulin from the microspheres.
Blood glucose reducing efficiency
The essence of this study was to access the anti-hyperglycaemic effects of the formulations and these were taken as a monitor for insulin release and absorption in its physiologically active form. The oral insulin microspheres prepared with Eudragit® RL 100 was assessed based on its effectiveness to reduce blood glucose levels in alloxan-induced (diabetic) rats. The administration of equal doses of insulin, subcutaneously, orally and in the formulations was to give direct comparison of the dose response in the study. The response curves obtained by plotting the blood glucose levels versus time are shown in . The formulations showed varying degrees of reduction in blood glucose level but with an initial increase in the first hour after administration in some animals which could be due to the stress associated with the administration of the microspheres (Simon, Citation2007; Builders et al., Citation2008a). A more efficient reduction in the blood glucose level could have masked this initial increase in other animals. A prominent anti-hyperglycemic effect was noticed with batches C1 and C4 formulations from 4 and 2 h respectively, and the effect was visible up to 12 h. This is an indication that the microspheres protected insulin against proteolytic degradation in the GIT, consistent with previous reports (Tiyaboonchai, Citation2003; Nakamura et al., Citation2004; Toorisaka et al., Citation2005). The maximum plasma glucose reduction from the baseline was found to be 64 mg/dl and the time to reach the maximum plasma glucose reduction was 12 h. Orally administered insulin solution, distilled water and unloaded microspheres preparation (C0) (serving as negative controls) showed a general increase in the blood glucose level unlike subcutaneously administered insulin that served as positive control. Reduction in the blood glucose level for the subcutaneously (sc) administered insulin was significantly (p ≤ 0.05) higher than for all the formulations except batch C4. Eudragit® RL is a low water permeable, cationic, non-biodegradable polymer commonly used for the preparation of controlled-release dosage forms, and represents a good material for the dispersion of drugs (Agyilirah & Banker, Citation2000; Haznedar & Dortunc, Citation2004; Apurba et al., Citation2009; Sahoo et al., Citation2009; Kishori et al., Citation2013). The high blood glucose reduction resulting from insulin-loaded microspheres may be attributed to insulin protection or absorption within the GIT. The microspheres prepared with 400 mg of magnesium stearate produced maximum blood glucose reduction 12 h after oral administration that was equal to that of subcutaneously administered insulin. also shows a slight reduction in blood glucose level after oral administration of insulin solution. It is quite improbable for insulin absorption to occur in the stomach (Chandler et al., Citation1994; Damage et al., Citation1995; Sarciaux et al., Citation1995; Iwanaga et al., Citation1997; Krauland et al., Citation2004; Li & Deng, Citation2004; Nakamura et al., Citation2004; Sajeesh & Sharma, Citation2006; Lin, Citation2007); thus the reduction in the blood glucose could be due to some of the insulin solution reaching the intestine since high doses of insulin solution were administered to the rats. Blood glucose reduction occurred within 30 min of oral administration in some samples. This could be attributed to such factors as early gastric emptying of the drug from the stomach into the small intestine and the effective mucoadhesiveness of the polymer matrix (Eudragit® RL 100) that was efficient in adhering the microspheres to the gastric mucosa and protecting the insulin from degradation which is one of the major drawbacks in oral insulin delivery (Beals & Kovach, Citation1997; Kisel et al., Citation2001; Owens, Citation2002; Giriraj & Giriraj, Citation2003; Gowthamarajan & Kulkarni, Citation2003; Kim & Peppas, Citation2003; Whitehead et al., Citation2004; Cui et al., Citation2006; Morishita & Peppas, Citation2006; Sarmento et al., Citation2007; Pamnani, Citation2008). It was helpful to protect insulin activity against the enzymatic attack in harsh environment of stomach and intestine. The modification of the microspheres and suitable size of the microspheres made them easily adhere on the intestinal mucosa and transfer into the blood circulation, consistent with similar studies (Rosa et al., Citation2000; Jain et al., Citation2005; Builders et al., Citation2008a). The results indicate that the microspheres could be orally administrated for management of diabetes.
Conclusions
Insulin-loaded mucoadhesive microspheres were successfully prepared using Eudragit® RL 100. In vivo study undertaken with the microspheres demonstrated a prominent hypoglycemic effect, suggesting that the microspheres could protect insulin against proteolytic degradation in the gastrointestinal tract. Results from the loading efficiency suggests that magnesium stearate at optimum amount could promote encapsulation of higher quantity of insulin using Eudragit® RL 100. Microspheres prepared with highest amount of magnesium stearate (400 mg) produced blood glucose lowering effect comparable to that of the subcutaneously administered insulin after 12 h. This indicates that oral delivery of insulin for effective control of blood glucose is indeed possible using the right carrier system and formulation technique and that insulin-loaded microspheres based on emulsification-coacervation technique could be an alternative for the delivery of insulin.
Declaration of interest
We declare that we have no conflict of interest.
References
- Agyilirah GA, Banker GS. (2000). Polymer for enteric coating application. In: Tarcha PJ, ed. Polymer for controlled drug delivery. : CRC press, 41–60
- Ahmed I, Goldstein B. (2006). Diabetes Mellitus. Clin Dermatol 24:237–46
- Akhter DT, Nijhu RS. (2012). Diabetes mellitus: a journey of insulin. Int Curr Pharm J 1:32–42
- Apurba SA, Atqul HP, Golam K, Reza-ul J. (2009). In vitro release kinetic study of theophylline from Eudragit® RL PO matrix tablets. Dhaka Univ J Pharm Sci 8:1–6
- Attivi D. (2005). Formulation of insulin-loaded polymeric nanoparticles using response surface methodology. Drug Dev Ind Pharm 31:179–89
- Beals JM, Kovach P. (1997). Insulin. In: Crommelin DJA, Sindelar RD, eds. Pharmaceutical Biotechnology, Vol. 10. : Harwood Academic Publishers, 229–39
- Builders PF, Ibekwe N, Okpako LC, et al. (2009). Preparation and characterization of mucinated cellulose microparticles for therapeutic and drug delivery purposes. Eur J Pharm Biopharm 72:34–41
- Builders PF, Kunle OO, Adikwu MU. (2008a). Preparation and characterization of mucinated agarose: a mucin-agarose physical crosslink. Int J Pharm 356:174–80
- Builders PF, Kunle OO, Okpaku LC, et al. (2008b). Preparation and evaluation of mucinated sodium alginate microparticles for oral delivery of insulin. Eur J Pharm Biopharm 70:777–83
- Caliceti P, Veronese EM. (1999). Improvement of the physicochemical and biopharmaceutical properties of insulin by poly (ethylene glycol) conjugation. STP Pharm Sci 9:107–13
- Cárdenas-Bailón F, Osorio-Revilla G, Gallardo-Velázquez T. (2013). Microencapsulation techniques to develop formulations of insulin for oral delivery: a review. J Microencapsul 30:409–24
- Cefalu WT. (2004). Evolving strategies for insulin delivery and therapy. Drugs 64:1149–61
- Chalasani KB. (2007). A novel vitamin B12-nanosphere conjugate carrier system for peroral delivery of insulin. J Control Rel 117:421–9
- Chandler SG, Thomas NW, Ilum L. (1994). Nasal absorption in the rat. III. Effect of orally lysophospholipids on insulin absorption and nasal histology. Pharm Res 11:1623–30
- Chetty DJ, Chien YW. (1998). Novel methods of insulin delivery: an update. GIT Rev Ther Drug Carrier Syst 15:629–70
- Chung H. (2004). Self-assembled nanocubicle as a carrier for peroral insulin delivery. Diabetologia 45:448–51
- Cui F, Shi K, Zhang L. (2006). Biodegradable nanoparticles loaded with insulin-phospholipid complex for oral delivery: preparation, in vitro characterization and in vivo evaluation. J Control Rel 114:242–50
- Cui FD. (2007). Preparation of insulin-loaded PLGA-Hp55 nanoparticles for oral delivery. J Pharm Sci 96:421–7
- Damge C, Hillaire-Buys D, Peuche R, et al. (1995). Effect of orally administered insulin nanocapsules in normal and diabetic dogs. Diabetes Nutr Metab 8:3–9
- Damge C, Maincent P, Ubrich N. (2007). Oral delivery of insulin associated to polymeric nanoparticles in diabetic rats. J Control Rel 117:163–70
- DeFronzo RA, Bergenstal RM, Cefalu WT. (2005). Efficacy of inhaled insulin in patients with type 2 diabetes not controlled with diet and exercise. Diabetes Care 28:1922–8
- Dorkoosh FA. (2002). Peroral delivery systems based on superporous hydrogel polymers: release characteristics for the peptide drugs buserelin, octreotide and insulin. Eur J Pharm Sci 15:433–9
- Eaimtrakarn S, Ramaprasad YV, Ohno T, et al. (2002). Absorption-enhancing effect of labrasol on the intestinal absorption of insulin in rats. J Drug Target 10:255–60
- Evans M, Schumm-Draeger PM, Vora J, King AB. (2011). A review of modern insulin analogue pharmacokinetic and pharmacodynamic profiles in type 2 diabetes: improvements and limitations. Diabetes Obes Metab 13:677–84
- Giriraj KG, Giriraj KT. (2003). Oral insulin – fact or fiction. Resonance 17:38–43
- Gowthamarajan K, Kulkarni GT. (2003). Oral insulin – fact or fiction? Possibilities of achieving oral delivery for insulin. Resonance 19:38–46
- Haznedar S, Dortunc B. (2004). Preparation and in vitro evaluation of Eudragit® microspheres containing acetazolamde. Int J Pharm 269:131–40
- Heinemann L. (2002). Alternative delivery routes: inhaled insulin. Diabetes Nutr Metab Dec 156:417–22
- Heinemann L, Pfutzner A, Heise T. (2001). Alternative routes of administration as an approach to improve insulin therapy: update on dermal, oral, nasal and pulmonary insulin delivery. Curr Pharm Des Sep 7:1327–51
- Hogan JE. (2008). Film–coating materials and their properties. In: Cole G, ed. Pharmaceutical coating technology. : Infoma Healthcare, 7–19
- Ichikawa H, Fukumori Y. (2007). Design of nanohydrogel-incorporated microcapsules for appropriate controlled-release of peptide drugs. Yakugaku Zasshi 127:813–23
- Iwanaga KS, Ono S, Naroika K, et al. (1997). Oral delivery of insulin by using surface coating liposomes for improvement of insulin in GI tract. Int J Pharm 157:73–80
- Jain D, Panda AK, Majumdar DK. (2005). Eudragit® S100 entrapped insulin microspheres for oral delivery. AAPS PharmSciTech 6:1–27
- Kavimandan NJ. (2006). Synthesis and characterization of insulin-transferrin conjugates. Bioconjug Chem 17:1376–84
- Kim B, Peppas NA. (2003). In vitro release behavior and stability of insulin in complexation hydrogels as oral drug delivery carriers. Int J Pharm 266:29–37
- Kinesh VP, Neelam DP, Punit BP, et al. (2010). Novel approaches for oral delivery of insulin and current status of oral insulin products. Int J Pharm Sci Nanotech 3:1057–64
- Kisel MA, Kulik LN, Tsybovsky IS, et al. (2001). Liposomes with phosphatidylethanol as carrier for oral drug delivery carriers. Int J Pharm 216:105–14
- Kishori LD, Nilima AT, Paraag SG. (2013). Formulation and development of tinidazole microspheres for colon targeted drug delivery system. J Pharm Res 5:158–60
- Krauland AH, Guggi D, Bernkop-Schnurch A. (2004). Oral insulin delivery: the potential of thiolated chitosan-insulin tablets on non-diabetic rats. J Control Rel 95:547–55
- Li CL, Deng YJ. (2004). Oil-based formulations for oral delivery of insulin. J Pharm Pharmacol 56:1101–7
- Lin YH. (2007). Preparation and characterization of nanoparticles shelled with chitosan for oral insulin delivery. Biomacromolecules 8:146–52
- Liu R, Huang SS, Wan YH, et al. (2006). Preparation of insulin-loaded PLA/PLGA microcapsules by a novel membrane emulsification method and its release in vitro. Colloids Surf B-Biointerfaces 51:30–8
- Morishita M, Matsuzawa A, Takayama K, et al. (1998). Improving insulin enteral absorption using water-in-oil-in-water emulsion. Int J Pharm 172:189–98
- Morishita M, Peppas NA. (2006). Is the oral route possible for peptide and protein drug delivery? Drug Discov Today 11:19–20
- Nakamura K, Murray RJ, Joseph JI, et al. (2004). Oral insulin delivery using P(MAA-g-EG) hydrogels: effects of network morphology on insulin delivery characteristics. J Control Rel 95:589–99
- Nilkumhang S, Basit AW. (2009). The robustness and flexibility of an emulsion solvent evaporation method to prepare pH-responsive microparticles. Int J Pharm 377:135–41
- Ofokansi KC, Adikwu MU. (2007). Formulation and evaluation of microspheres based on gelatin-mucin admixtures for the rectal delivery of cefuroxime sodium. Trop J Pharm Res 6:825–32
- Ofokansi KC, Kenechukwu FC, Ogwu NN. (2014). Design of novel miconazole nitrate transdermal films based on Eudragit RS100 and HPMC hybrids: preparation, physical characterization, in vitro and ex vivo studies. Drug Deliv [Epub ahead of print]. DOI: 10.3109/10717544.2013.875604
- Owens DR. (2002). New horizons—alternative routes for insulin therapy. Nat Rev Drug Discov 1:529–40
- Pamnani D. (2008). Reality check on oral insulin. Pharma Express 3:16–31
- Rosa GD, Iommeli R, Rotonda MIL, et al. (2000). Influence of the co-encapsulation of different non-ionic surfactants on the properties of PLGA insulin-loaded microspheres. J Control Rel 69:283–95
- Rosenstock J. (2001). Insulin therapy: optimizing control in type 1 and type 2 diabetes. Clin Cornerstone 4:50–64
- Sahoo J, Murthy PN, Biswal S, Manik D. (2009). Formulation of sustained release dosage form of verapamil hydrochloride by solid dispersion technique using Eudragit® RLPO or Kollidon® SR. AAPS Pharm Sci Tech 10:27–33
- Sajeesh S, Sharma CP. (2006). Cyclodextrin-insulin complex encapsulated polymethacrylic acid based nanoparticles for oral insulin delivery. Int J Pharm 325:147–54
- Sarciaux JM, Acar L, Sado PA. (1995). Using microemulsion formulations for oral delivery of therapeutic peptides. Int J Pharm 120:127–36
- Sarmento B. (2006). Development and characterization of new insulin containing polysaccharide nanoparticles. Colloids Surf B Biointerfaces 53:193–202
- Sarmento B, Martins S, Ferreira D, Souto EB. (2007). Oral insulin delivery by means of solid lipid nanoparticles. Int J Nanomed 2:743–9
- Shima M, Tanaka M, Fujii T, et al. (2006). Oral administration of insulin included in fine W/O/W emulsions to rats. Food Hydrocolloids 20:523–31
- Simon M. (2007). Nanosized insulin-complexes based on biodegradable amine-modified graft polyesters Poly (vinyl-3-(diethylamino)-propylcarbamate-co-(vinyl acetate)-co-(vinyl alcohol)]-graft-poly (l-lactic acid): protection against enzymatic degradation, interaction with Caco-2 cell monolayers, peptide transport and cytotoxicity. Eur J Pharm Biopharm 66:165–72
- Singh MN, Hemant KSY, Ram M, Shivakumar HG. (2010). Microencapsulation: a promising technique for controlled drug delivery. Res Pharm Sci 5:65–77
- Singh S, Patel D, Patel NR, et al. (2010b). Insulin oral delivery may be possible. Int J Pharm Prof Res 1:46–51
- Tiyaboonchai W. (2003). Insulin containing polyethyleni-mine-dextran sulfate nanoparticles. Int J Pharm 255:139–51
- Toorisaka E, Hashida M, Kamiya N, et al. (2005). An enteric-coated dry emulsion formulation for oral insulin delivery. J Control Rel 107:91–6
- Tozaki H. (2001). Enhanced absorption of insulin and (Asu (1, 7)) eel-calcitonin using novel azopolymer-coated pellets for colon-specific drug delivery. J Pharm Sci 90:89–97
- Tozaki H, Komike J, Tada C, et al. (1997). Chitosan capsules for colon-specific drug delivery: improvement of insulin absorption from rats. J Pharm Sci 86:1016–21
- Tuesca A, Lowman A. (2006). The oral delivery of insulin using protein conjugates in complexation hydrogels. Poster presentation, Biomaterials and drug delivery laboratory, Drexel University, Philadelphia, Pennsylvania, USA
- Whitehead K, Shen Z, Mitragotri S. (2004). Oral delivery of macromolecules using intestinal patches: applications for insulin delivery. J Control Rel 98:37–45
- Yamamoto A, Taniguchi T, Rikyuu K, et al. (1994). Effect of various protease inhibitors on the intestinal absorption and degradation of insulin in rats. Pharm Res 11:1496–500
- Yüksel N, Baykara T. (1997). Preparation of polymeric microspheres by the solvent evaporation method using sucrose stearate as a droplet stabilizer. J Microencapsul 14:725–33
- Zhang Y, Wu X, Meng L, et al. (2012b). Thiolated Eudragit nanoparticles for oral insulin delivery: preparation, characterization and in vivo evaluation. Int J Pharm 436:341–50
- Zhao N, Augsburger LL. (2005). The influence of swelling capacity of superdisintegrants in different pH media on the dissolution of hydrochlorothiazide from directly compressed tablets. AAPS Pharm Sci Tech 6:E120–6
- Zhang Y, Wei W, Lv P, et al. (2011). Preparation and evaluation of alginate–chitosan microspheres for oral delivery of insulin. Eur J Pharm Biopharm 77:11–9