Abstract
The objectives of this study were first to encapsulate norcantharidate into albumin microspheres by the emulsion crosslinking method and second to characterize the microspheres in terms of the morphological examination, particle size, and encapsulation efficiency. The in vitro release of norcantharidate from the microspheres was studied by using the dialysis bag method. Pharmacokinetics and biodistribution studies were used to evaluate the advantages of microspheres than the conventional formulations. The microspheres prepared by crosslink emulsion were with uniform size, smooth surface, spherical shape, and disperse evenly. The particle size was uniform (13.3 ± 0.4 µm) and the encapsulation efficiency was 54.3 ± 4.18%. In vitro release indicated that the norcantharidate microspheres had a well-sustained release efficacy and fitted Korsmeyer’s Peppas release model. In vivo studies showed that pharmacokinetics of norcantharidate microspheres could be described by the model of two-compartment after i.v. administration and had higher AUC inside liver and spleen than the injection group. No histological change occurred to the rat liver after the administration of norcantharidate microspheres.
Introduction
Norcantharidate (NCTD, ) is a demethylated analogue of cantharidin isolated from the dried body of the Chinese blister beetle (Wang, Citation1989). It was found that NCTD is one of the new chemotherapy agents that have been shown to be very effective against cancer, breast cancer, abdominal cancer, and especially against primary hepatic carcinoma and has the advantage of inducing the production of leucocytes (Yi et al., Citation1991). Clinical studies showed that NCTD was effective against primary liver cancer but its main clinically adverse effect is that it produces gastrointestinal and urinary system toxicity (Liu et al., Citation1995; Tagwireyi et al., Citation2000). Side-effects may limit its use and decreased the dose. Additionally, due to the maximum solubility of NCTD in water is 2.5 mg/ml at pH 6.0 and this increase to 9.5 mg/ml at pH 9.5. Clinically, NCTD is mostly given by injection of its sodium salt (5 mg/ml, 2 ml) at a pH of about 9.0. The high pH may produce intense irritation at the injection site. Meanwhile, NCTD has a short biological half-life of 2–4 h and is eliminated rapidly after oral or intravenous administration. Clinically, in order to maintain an effective therapeutic concentration, it requires frequent small doses of NCTD injections to patients, which causes both pain and inconvenience to patients and leads to side effects because of accumulated blood concentration. Hence, the aforementioned limitation of NCTD may be overcome by encapsulating the drug in one carrier for chemoembolization at the target site. Recently, to improve the effectiveness of intratumoural chemotherapy, various NCTD-loaded microspheres have been designed to prolong very high intratumoural drug concentrations and further minimize any risk of systemic toxicity (Lin et al., Citation2012; Wang & Guo, Citation2008a,Citationb; Wang et al., Citation2008). In the current study, albumin microspheres were used as carriers for the anti-tumour agent NCTD.
Microspheres can be defined as solid, approximately spherical particles and be prepared from a variety of synthetic polymers and biopolymers have been widely studied for their application in cancer treatment (Kawaguchi, Citation2000; Okada & Toguchi, Citation1995). Among the various biodegradable particulate drug carriers available for consideration, we regard the most abundant natural plasma protein, serum albumin, as a most appealing biocompatible carrier for drug delivery (Longo & Goldberg, Citation1985; Longo et al., Citation1982). Nowadays, the paclitaxel–human albumin nanosuspension freeze-dried powder (Abraxane), developed by the US Bioscience Company (American BioScience Inc. Blauvelt, NY), is now available in many countries worldwide. The III period clinical study showed that the incidence of allergic reactions is less than 1%, which is significantly lower than Taxol with higher response rate.
The objectives of this study are first to encapsulate NCTD into albumin microspheres by the emulsion cross-linking method and second to characterize the microspheres in terms of particle size, morphology, encapsulation efficiency, and drug release. Moreover, pharmacokinetics and biodistribution studies of NCTD-loaded microspheres were investigated.
Materials
NCTD was bought from Sukang Pharmaceutical Company (batch: 03421, Suzhou, China). Human serum albumin (HAS, 25%) was obtained from Baxter, China. Glutaraldehyde, span-80, castor oil, and diethyl ether were purchased from Sinopharm, China.
Microsphere preparation
DSNC-loaded albumin microspheres were prepared by the emulsion cross-linking method (Mathew et al., Citation2009). Briefly, about 30 mg of NCTD was added to 2.5 ml of diethyl ether. After completely dissolved, the solution is then slowly added to castor oil (25 ml) containing a surfactant (0.25 g span-80) and homogenized at high stir rates, forming a water-in-oil emulsion before the addition of a cross-linking agent. Then 25% glutaraldehyde solution is slowly added to the emulsion system and cross-linked for 2 h until the microspheres were solidified. The microspheres were collected by filtration through a 20 μm sieve, and then washed three times with deionized water. After 1 ml of aqueous mannitol (20%, w/v) was added to prevent the aggregation of microparticles, the microspheres were freeze dried.
Morphology and particle size distribution
The surface morphology of the microspheres was observed by transmission electron microscope (TEM) (Philips CM120, Eindhoven, The Netherlands). In practice, a drop of microspheres solution containing 0.1% (w/v) phosphotungstic acids was placed on a carbon film coated on a copper grid and observed at 80 kV in the electron microscope. Particle size distribution and mean diameter of the prepared NCTD-loaded microspheres were determined by dynamic light scattering (DLS) using a NICOMP 380 Submicron Particle Sizer (Santa Barbara, CA) equipped with a 5 mW heliumneon laser at 632.8 nm. Sample solutions filtered through a 0.22 µm filter membrane were transferred into the light scattering cells. The intensity autocorrelation was measured at a scattering angle of 90 °C at room temperature. Data were analyzed in terms of intensity-weighted NICOMP distributions. Each reported experimental result is the average of at least three dh (hydrate dynamic diameter) values obtained from analysis of the autocorrelation function accumulated for at least 20 min.
Encapsulation efficiency
Encapsulation efficiency (EE%) was calculated as described earlier (Kim & Lee, Citation2001; Shi et al., Citation2005). First, lyophilized microspheres (30 mg) were dissolved in 2 ml of acetonitrile and then 8 ml of purified water was added to precipitate the polymer matrix. The resulting solution was centrifuged for 10 min at 10 000 rpm and the supernatant was collected for HPLC analysis. The encapsulation efficiency was determined as the mass ratio of entrapped NCTD in microspheres to the theoretical amount of drug used in their preparation.
In vitro release
Ten milligrams of freeze-dried NCTD microspheres were poured in a dialysis bag (MWCO = 10 kDa) and suspended in 16 ml of 0.05 M phosphate buffer solution (pH 7.4). The bag was placed under magnetic stirring in a water bath maintained at 37 ± 0.5 °C (a rate of 100 per min). Samples of 0.2 ml were removed from the tubes at sampling times of 5, 15, 30 min, 1, 2, 4, 6, 8, and 10 h after centrifugation at 3000 rpm for 10 min. Then 0.2 ml of the fresh buffer was replenished. The obtained solution was analyzed by HPLC to determine the drug concentration. The experiments were carried out in triplicate.
Stability
This formulation uses albumin as its deliver carrier; thus, the choice on the stability of conditions considers the nature of the albumin. Since albumin is of protein origin and has relatively low thermal stability profile, it is prone to degeneration at 25 °C (Sree & Shobha, Citation2006). So the microspheres were stored at 25 ± 2 °C and 60 ± 5% relative humidity (RH) for a period of 6 months and at 4 ± 1 °C for a period of 12 months, which is the accelerated storage temperature and long-term storage temperature, respectively. The stored samples were tested for their drug content, particle size distribution, and for any physical change. The drug content was determined by a HPLC method.
Pharmacokinetic study
Twelve S.D. rats (half males, weight 220 ± 20 g) were assigned intravenous (i.v.) administration of 10 mg/kg NCTD-loaded microspheres and NCTD injection. Orbital blood samples were collected immediately before and at 2, 5, 10, 30 min and 1, 2, 4, 6, 8, 12 h after drug administration. The blood samples (0.5 ml) were withdrawn into heparinized Eppendoff tubes and were centrifuged at 12 000 rpm for 10 min at 4 °C. A 0.2 ml volume of plasma was obtained and stored at −20 °C until analysis. Pharmacokinetic parameters were calculated from the plasma concentration–time data. The elimination half-life (T1/2) was determined by linear regression of the terminal portion of the plasma concentration–time data. The area under the plasma concentration–time curve from zero to the last measurable plasma concentration point (AUC0–t) was calculated by the linear trapezoidal method. Extrapolation to time infinity (AUC0–∞) was calculated as follows: AUC0–∞ = AUC0–t + Ct/ke, where Ct is the last measurable plasma concentration and ke is the terminal elimination rate constant.
Tissue distribution
Forty-eight S.D. rats (half males, weight 220 ± 20 g) were randomly divided into two group with 24 for each group. NCTD injection and microspheres were, respectively, injected in the same method of pharmacokinetics experiment. Six rats in each group were killed at 30 min, 2, 6, and 12 h after administration. Heart, liver, spleen, lung, kidney, and blood were taken out immediately and washed with distilled water, and then 0.5 g of tissue sample was collected, respectively, after surface water was dried. The drug concentration in tissues was determined by LC-MS/MS.
Histopathological studies
After 12 h of administration of the formulation, animals (including control saline group) were sacrificed by excess anesthesia and the liver was dissected and washed with cold saline. The organs were pressed between filter pads and weighed. Liver tissues were fixed in 10% neutral formalin and embedded in paraffin. The paraffin sections were cut on glass slides and stained with eosin for histopathological examination. The sections were examined under a light microscope to detect any damage to the tissue with 500 × magnification (Nanji et al., Citation2002).
LC-MS/MS analysis
An Agilent 1100 system consisting of a G1312A quaternary pump, a G1379A vacuum degasser, a G1316A thermostated column oven (Agilent, Waldbronn, Germany), and a HTS PAL auto sampler (CTC Analytics, Zwingen, Switzerland) was used. Mass spectrometric detection was performed on an API 3000 triple quadrupole instrument (Applied Biosystems, Toronto, Canada) in multiple reaction monitoring (MRM) mode. A Turbo Ion Spray ionization (ESI) interface in positive ionization mode was used. Data processing was performed with Analyst 1.4.1 software package (Applied Biosystems, Toronto, Canada).
The chromatographic separation was achieved on a Gemini C18 column (150 mm × 2.0 mm, 5 µm, Phenomenex, Torrance, CA) with a Security Guard C18 guard column. A mixture of acetonitrile and water (12:88, pH 3.1, adjusted with phosphoric acid) was used as a mobile phase at a flow rate of 0.3 ml/min. The temperature of column and auto sampler was maintained at 40 and 4 °C, respectively. The chromatographic run time of each sample was 3.0 min. The mass spectrometer was operated using an ESI source in the positive ion detection. Quantitation was done using MRM mode to monitor protonated precursor → product ion transition of m/z 169 → 123 for NCTD and 267 → 135 for IS (ribavirin 1 µg/ml, 100 µl, ).
All the parameters of LC and MS were described as follows: turbo spray voltage (IS) was set at 4500 V. The source temperature was maintained at 550 °C. The entrance potential (EP) was set at 6 V. Nitrogen was used as a nebulizing gas (10 L/min) and curtain gas (8 L/min). For collision activated dissociation (CAD), nitrogen was employed as the collision gas at a pressure of 4 L/min.
Statistical analysis
All data were collected from at least three independent experiments, and the data were expressed as the mean ± standard deviation (SD). Comparisons of two different groups were performed using an unpaired Student’s t-test. A significance level of p < 0.05 denoted significance in all cases.
Results and discussion
Microsphere preparation
Due to different preparation conditions, the prepared NCTD microspheres have different properties. This study found that the main factors affecting NCTD microspheres during the optimization process include initial drug dosage and the proportion of albumin, concentration of the surface active agent, and the time of emulsifying cross link. The final optimization result showed that when the dosage is 1:9 with albumin, using 25% of the glutaraldehyde solution, the microspheres prepared by 2 h crosslink emulsion were with uniform size, smooth surface, spherical shape, and disperse evenly. Examination using transmission electron micrographs showed spherical particles with many pores, which is responsible for solvent evaporation (). The particle size distribution is an important factor since it controls the tissue location of the microspheres after their intra-arterial infusion. Previous reports pointed out that the microspheres with the size range of 5–25 µm have a notable liver-targeting capacity (Kanke et al., Citation1980). The mean diameter was 13.3 ± 0.4 µm from three batches. The poly disperse index (PDI) was 0.129 ± 0.039 and the zeta potential was −12.1 ± 0.8 mV. The average encapsulation efficiency was 54.3 ± 4.18%.
In vitro release
shows the NCTD release curve from NCTD injection and NCTD microspheres. The release curve showed that free NCTD released rapidly in this release medium, which can be released completely (96%) within 1 h. This phenomenon is called burst phenomenon clinically. While, on one hand, the drug may cause temporary high concentration, on the other hand, the drug efficiency cannot be sustained. On the contrary, NCTD microsphere group can release the drug slowly with only 80% drug release within 8 h. The results indicated that the NCTD microspheres had a well-sustained release efficacy. The in vitro release was kinetically analyzed according to the first-order, Baker and Lonsdale, Korsmeyer’s Peppas and Higuchi diffusion-controlled release mechanism. The correlation coefficient value, R2, is taken into account to decide upon the relevance of the model/curve fit which will best describe the extent of fit. According to the R2 values given by different data fits for NCTD microspheres, Korsmeyer’s Peppas model was to be an ideal fit having R2 = 0.9993. According to Korsmeyer’s Peppas fit, the release of the drug is decided upon by the diffusion of the polymeric matrix and the drug release is governed by Fick’s law of diffusion.
Figure 3. In vitro release profiles of NCTD-loaded microspheres from three batches. Release experiments were carried out in phosphate buffer solution (PBS) (pH 7.4), at 37 ± 0.5 °C. Each point represents the mean value of three different experiments ± S.D. ⋄: NCTD injection; ▵: NCTD microspheres. *p < 0.05: NCTD-loaded microspheres (▵) versus NCTD injection (⋄).
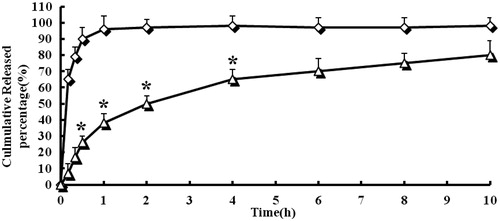
Stability
In both accelerated and long-term stability tests, the data showed no significant change in NCTD microspheres (). During the observation period, the microspheres maintained good round shape. The particle size remained substantially unchanged between 13.4 and 13.6 μm. With the prolonged preservation time, the drug content declined and the accelerated stability data showed that NCTD content decreased by 2.3% (time = 6 m). The long-term stability data showed that NCTD content decreased by 2.2% (time = 12 m). It was obvious that the NCTD microspheres prepared by the emulsion cross-linking method in this experiment were stable with controllable quality.
Table 1. Stability data of NCTD microspheres.
Pharmacokinetics
The in vivo pharmacokinetics of microspheres was studied with “3P97” and was fitted by one-compartment model, two-compartment model and three-compartment model. shows the mean plasma concentration–time profiles of NCTD after i.v. administration of a single 10 mg/kg dose of injection and microspheres to rats. Based on the analysis of the models and parameters, it was concluded that the in vivo pharmacokinetics of microspheres in blood could be described by the model of two-compartment with i.v. injection. The plasma concentration and pharmacokinetic parameters are reported in and , respectively. From , one can see that in comparison with NCTD injection, NCTD microspheres altered the distribution of NCTD in vivo and the half-life after i.v. injection of NCTD microspheres (t1/2(α) = 0.92 h, t1/2(β) = 23.52 h) were prolonged remarkably than those (t1/2(α) = 0.56 h, t1/2(β) = 14.19 h) after i.v. injection of NCTD injection. The result indicated that the NCTD microspheres had sustained release efficacy.
Figure 4. Mean plasma concentration–time profiles of NCTD after i.v. administration of a single 10 mg/kg dose of injection and microspheres to rats (each point represents the mean ± SD of six rats). *p < 0.05: NCTD-loaded microspheres (▵) versus NCTD injection (⋄).
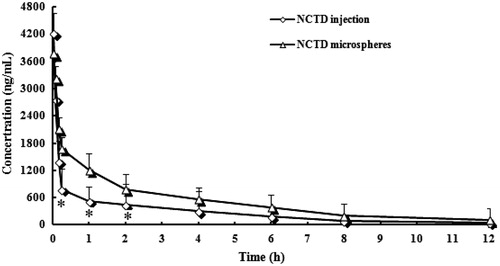
Table 2. Pharmacokinetic parameters of the two formulations.
Table 3. The AUC0–12h of NCTD in plasma and tissues after i.v. administration of injection and microspheres to rats (n = 6).
In addition, NCTD has strong polarity. After intravenous injection, rats had a faster rate of drug distribution inside the body with short elimination time and difficult to accumulate. After the NCTD was made to microspheres, AUC and t1/2(β) increased significantly, which were 1.11 times and 1.66 times respectively of injections. The rate of in vivo clearance was significantly reduced. It proved that albumin microspheres NCTD can significantly improve the drug targeting, rapidly distribute the drug in the body, prolong the drug circulation time in vivo, significantly increase the relative bioavailability of the drug, and also contribute to its anti-tumor effect.
Biodistribution studies
and present the mean concentration–time profiles of NCTD in unit mass of each organ in rats. The drug concentration in tissues indicated that the microspheres could deliver NCTD mainly to liver after intravenous administration. From the perspective of the drug concentration in blood, its data were close to the pharmacokinetic data; the AUC0–t of microspheres was 1.27 times of injection (1.11 times in pharmacokinetics). The concentration of NCTD microspheres in liver was significantly higher than those in other tissue and plasma (). The total amount of drug accumulated in each organ within 12 h (AUC0–t) was calculated, and the results are shown in . The classical Gupta method (Gupta & Hung, Citation1989) was usually used to evaluate the targeting efficiency (Target Index, TI). As shown in , NCTD microspheres had higher AUC inside liver and spleen than the injection group. TI was 3.49 and 1.03 but there was no significant difference in the increase of the spleen. The reasons may due to that the reticulo endothelial system (RES) was mainly in the liver, spleen, bone marrow, and other organs. The microspheres were thus easily to be detected and swallowed by RES. Thus, the drug made to microspheres carrier was more easily targeted to the above tissue.
Histopathological studies
In case of the targeted drug delivery system such as microspheres, major portion of the drug and excipient are accumulated in specific tissue (liver), and therefore, determining the compatibility between these tissues and the microspheres formulation becomes a necessity in order to rectify the safety of the formulations. On comparison of the microspheres formulations with the placebo group, the cytoarchitecture of the tissue (liver) did not show any major difference (degenerative changes) as evidenced in . Based on this observation, no histological change occurred to the rat liver after the administration of NCTD microspheres.
Conclusion
This study was to encapsulate NCTD into albumin microspheres by the emulsion cross-linking method. The microspheres prepared were with uniform size, smooth surface, spherical shape, and disperse evenly. In vitro release indicated that the NCTD microspheres had a well-sustained release efficacy and fitted Korsmeyer’s Peppas release model. In vivo studies showed that pharmacokinetics of NCTD microspheres could be described by the model of two-compartment after i.v. administration and had higher AUC inside liver and spleen than the injection group. No histological change occurred to the rat liver after the administration of NCTD microspheres.
Declaration of interest
The authors declare that they have no conflict of interest with respect to this paper. Grant support: Major Program of Shanghai Committee of Science and Technology Development (Qiang Fu, 06DZ19010).
References
- Gupta PK, Hung CT. (1989). Albumin microspheres. II: applications in drug delivery. J Microencapsul 6:463–72
- Kanke M, Simmons GH, Weiss DL, et al. (1980). Clearance of 141Ce-labeled microspheres from blood and distribution in specific organs following intravenous and intra arterial administration in beagle dogs. J Pharm Sci 67:755–65
- Kawaguchi H. (2000). Functional polymer microspheres. Prog Polym Sci 25:1171–210
- Kim SY, Lee YM. (2001). Taxol-loaded block copolymer nanospheres composed of methoxy poly(ethylene glycol) and poly(epsilon-caprolactone) as novel anticancer drug carriers. Biomaterials 22:2049–56
- Lin X, Zhang B, Zhang K, et al. (2012). Preclinical evaluations of norcantharidin-loaded intravenous lipid microspheres with low toxicity. Expert Opin Drug Deliv 9:1449–62
- Liu XH, Blazsek I, Comisso M, et al. (1995). Effects of norcantharidin, a protein phosphatase type-2A inhibitor, on the growth of normal and malignant haemopoietic cells. Eur J Cancer 31A:953–63
- Longo W, Iwata H, Lindheimer TA, Goldberg EP. (1982). Preparation of hydrophilic albumin microspheres using polymeric dispersing agents. J Pharm Sci 71:1323–8
- Longo WE, Goldberg EP. (1985). Hydrophilic albumin microspheres. Meth Enzymol 112:18–26
- Mathew ST, Devi SG, Prasanth VV, Vinod B. (2009). Formulation and in vitro–in vivo evaluation of ketoprofen-loaded albumin microspheres for intramuscular administration. J Microencapsul 26:456–69
- Nanji AA, Su GL, Laposata M, French SW. (2002). Pathogenesis of alcoholic liver disease–recent advances. Alcohol Clin Exp Res 26:731–6
- Okada H, Toguchi H. (1995). Biodegradable microspheres in drug delivery. Crit Rev Ther Drug Carrier Syst 12:1–99
- Shi B, Fang C, You MX. (2005). Stealth MePEG-PCL micelles: effects of polymer composition on micelle physicochemical characteristics, in vitro drug release, in vivo pharmacokinetics in rats and biodistribution in S180 tumor bearing mice. Colloid Polym Sci 283:954–67
- Sree H, Shobha R. (2006). Drug targeting to lungs by way of microspheres. Arch Pharm Res 29:598–604
- Tagwireyi D, Ball DE, Loga PJ, Moyo S. (2000). Cantharidin poisoning due to “Blister beetle” ingestion. Toxicon 38:1865–9
- Wang GS. (1989). Medical uses of mylabris in ancient China and recent studies. J Ethnopharmacol 26:147–62
- Wang S, Guo S. (2008a). Disodium norcantharidate-loaded poly(epsilon-caprolactone) microspheres II. Modification of morphology and release behavior. Int J Pharm 353:15–20
- Wang S, Guo S. (2008b). Formation mechanism and release behavior of poly(epsilon-caprolactone) microspheres containing disodium norcantharidate. Eur J Pharm Biopharm 69:1176–81
- Wang S, Guo S, Cheng L. (2008). Disodium norcantharidate loaded poly(epsilon-caprolactone) microspheres I. Preparation and evaluation. Int J Pharm 350:130–7
- Yi SN, Wass J, Vincent P, Iland H. (1991). Inhibitory effect of norcantharidin on K562 human myeloid leukemia cells in vitro. Leuk Res 15:883–6