Abstract
Chronotherapy or pulsatile drug delivery system could be achieved by increasing drug plasma concentration exactly at the time of disease incidence. Cholesterol synthesis shows a circadian rhythm being high at late night and early in the morning. Simvastatin (SIM) inhibits hydroxymethylglutaryl coenzyme A reductase, which is responsible for cholesterol synthesis. In this study, SIM lipid-based formulation filled in gelatin capsules and coated with aqueous Eudragit® S100 dispersion was prepared for chronotherapeutic treatment of hypercholesterolemia. The pharmacokinetic parameters of SIM capsules were studied in human volunteers after a single oral dose and compared with that of Zocor® tablets as a reference in a randomized cross-over study. Pharmacokinetic parameters such as AUC0–∞, Cmax, Tmax, t1/2 and elimination rate constant were determined from plasma concentration-time profile for both formulations. The tested formulation had the ability to delay drug absorption and provide higher drug concentrations from 3 up to 10 h after oral administration compared to that of commercial tablets. The data in this study revealed that the prepared formulation could be effective in chronotherapeutic treatment of hypercholesterolemia. Moreover, the tested formulation was found to enhance SIM bioavailability by 29% over the reference tablets.
Introduction
Hypercholesterolemia is a problem faced by many societies and is a cause of concern for health professionals since it constitutes one of the major risk factors for the development of cardiovascular diseases (Shabana et al., Citation2013). Hydroxymethylglutaryl coenzyme A reductase (HMG-CoA) inhibitors, statins, are the principal therapeutic for treatment of hypercholesterolemia (Liao, Citation2005). HMG-CoA activity is highest from mid night to early morning, which provides physiological need for HMG-CoA inhibition preferentially during this time. Simvastatin (SIM), one of the statins, is a poorly water soluble non-hygroscopic white crystalline powder. SIM is only available in tablets dosage form, with dose ranging from 20 to 80 mg/d. SIM has been proved to decrease total mortality risk by reducing death caused by coronary heart disease and lowering the risk of myocardial infarction (Ward et al., Citation2007). It is also effective in lowering low density lipoprotein plasma levels (Zhang et al., Citation2010). Moreover, some anticancer drugs such as doxorubicin believed to have drug resistance due to drug efflux via P-glycoprotein. Amount of cholesterol in plasma membrane is one of the factors responsible for P-glycoprotein activity, so administration of SIM with these drugs may enhance their cellular uptake and potentiate their antineoplastic effect (Kopecka et al., Citation2011).
Chronotherapy is a kind of disease treatment, which provides a relatively higher in vivo drug concentration exactly at the time of crises or disease attack (Yassin et al., Citation2012). There is evidence that the pharmacological properties of drugs could be altered by the circadian rhythm (Sarasija & Stutie, Citation2005). Cholesterol synthesis shows a circadian phase-dependent pattern. High level of cholesterol production occurs during late night or early in the morning. So, cholesterol-lowering drugs are preferably taken at night rather than day (Smolensky & Peppas, Citation2007; Mandal et al., Citation2010). Unfortunately, traditional SIM products could not provide reasonable SIM plasma concentrations at that specific time even if the patient takes the tablets at bed time.
Formulation of time-dependent and site-specific drug delivery system is an important strategy for approaching chronotherapeutic treatment (Chourasia & Jain, Citation2003). Therefore, colonic drug delivery systems (CDDSs) could be utilized as chronotherapy for diseases that show a circadian rhythm and having peak symptoms at late night or early morning (Ghimire et al., Citation2007; Mastiholimath et al., Citation2007) like in the case of nocturnal asthma and in vivo cholesterol production. Utilizing pH-dependent polymers such as Eudragit® S100 as coating material provide a time-dependent and site-specific characteristic to the formulation, which could be helpful in developing CDDSs and hence pulsatile drug delivery systems effective as chronotherapy (Ali et al., Citation2010). CDDS could be in a capsule dosage form contain drug molecules in suspension (Niwa et al., Citation1995; Takaya et al., Citation1995). Coating these capsules with polymeric coating materials allows the coated capsules to withstand the acidic gastric fluid and provides a colon drug targeting to the formulation. Subsequently, the coating material disintegrates and releases the drug in the duodenum or jejunum allowing drug absorption (De Oliveira et al., Citation2009). According to biopharmaceutical classification system, SIM is a class II drug, so its solubility is the rate-limiting step in the dissolution process and hence its bioavailability (Stella & Nti-Addae, Citation2007). Several strategy for delivery of this kind of drugs were used, among these strategy is the lipid-based formulations. Lipid-based formulations have been established to enhance the bioavailability of water-insoluble drugs (Taha et al., Citation2007; Pouton & Porter, Citation2008). These formulations were divided into four different classes. Class IV contains surfactants and co-solvents (Pouton, Citation2000, Citation2006). Class IV lipid-based formulations can be used to accelerate the dissolution step of lipophilic drugs in gastrointestinal tract (GIT) and hence its absorption (Humberstone & Charman, Citation1997), particularly in low fluid content of the colon (Barakat et al., Citation2011).
In a previous work (submitted for publication, 2014), six SIM capsules formulations were prepared utilizing different amount of Cremophor RH 40 Capmul MCM EP and polyethylene glycol (PEG) 400; SIM concentration was fixed in all formulations (40 mg). All capsules were coated with Eudragit S100 coating dispersion until 20% weight gain was obtained. SIM-coated capsules were evaluated for SIM release in simulated gastric fluid (0.1 N HCl of pH 1.2 for 2 h) and in simulated intestinal fluid (phosphate buffer solution of pH 6.8 for 3 h and pH 7.4 till the end of the test) as dissolution media. It was found that SIM-coated capsules containing SIM (40 mg), Cremophor RH 40 (100 mg), Capmul MCM EP (80 mg) and PEG 400 (20 mg) succeeded to withstand up to 2 h at pH 1.2 and had the highest in vitro drug release (88% in 12 h). A logical extension of the work would be to evaluate whether or not SIM is bioavailable from the prepared capsules.
Therefore, the aim of this work was to investigate the pharmacokinetics properties of new SIM-coated capsules as chronotherapy for treating hypercholesterolemia in human volunteers, utilizing pH-dependent solubility behavior of Eudragit® S100; also to investigate the effect of lipid-based formulation in the bioavailability of SIM in comparison to that of commercial Zocor® tablets.
Materials and methods
Materials
SIM and lovastatin acid were gifts from EPICO pharmaceutical company, Sharqiyah, Egypt. Capmul MCM EP (glyceryl caprylate) was from Abitec Corporation, Janesville, WI. Cremophor RH 40 (polyoxyl 40 hydrogenated castor oil) was from BASF, Ludwigshafen, Germany. Eudragit® S100 powder was from Rohm Pharma, Weiterstadt, Germany. PEG 400 was from Winlab, England, UK. Ethanol, ammonia, acetonitrile, ammonium phosphate, triethyl citrate, ethyl cellulose, methyl tert-butyl ether, hydroxypropyl methylcellulose (HPMC) and Tween 80 were from Sigma-Aldrich Chemical Company, St. Louis, MO. Zocor® 40 mg tablets, Merck Sharp & Dohme, Haarlem, the Netherlands.
Preparation of SIM-coated capsules
SIM formulations were prepared by melting Cremophor RH 40 (100 mg), Capmul MCM EP (80 mg) and PEG 400 (20 mg) at 40 °C. Then 40 mg of SIM was added, and the mixture was sonicated using probe sonicator for 30 min then filled in hard gelatin capsules. All SIM capsules were sealed with 5% alcoholic solution of ethyl cellulose. SIM capsules were coated using Caliva Mini Coater/Drier with aqueous Eudragit® S100 dispersion (10% w/w) partially neutralized with 5% of one molar ammonia solution. Triethyl citrate, equivalent to 6% w/w, was added along with 2% Tween 80 solution (33% aqueous solution) to the coating solution (Barakat et al., Citation2011). SIM capsules were coated with Eudragit® S100 coating dispersion till 20% weight gain. Coating level was calculated as the percent weight gain between coated and uncoated capsules. SIM capsules were coated first with an intermediate layer of 10% aqueous solution of HPMC till 3% weight gain and then with Eudragit S100 coating dispersion till weight gain 20%.
Pharmacokinetics and bioequivalence study in healthy volunteers
For the assessment of the pharmacokinetics and bioequivalence, six healthy male adult volunteers participated in fasting open randomized two-period crossover experiment. The average ages and body weights were 53 ± 4.3 years and 73 ± 6.8 kg, respectively. One month was kept as a wash out period before crossover study. After explaining the research protocol with possible side effects, the volunteers were asked to sign consent forms. Verbal assurance was taken from all volunteers that they have not taken any drugs during and for one week preceding the experiment day. The selected volunteers were considered healthy on the basis of detailed medical history. During the test period, all subjects remained under close medical supervision and were supplied uniform diets. Venous blood samples were withdrawn by an indwelling catheter into heparin-containing tubes immediately just before dosing and after specific time intervals of drug administration. The blood samples were centrifuged at 5000 rpm for 15 min (CT5, Germany), and the separated plasma samples were frozen until analysis.
Sample preparation
The frozen plasma samples were thawed at ambient temperature before extraction. One-hundred milliliters of internal standard solution was added to 1 ml of each plasma sample. Internal standard, lovastatin acid, was dissolved in acetonitrile and water mixture at a ratio of 6:4 v/v at concentration of 10 μg/ml. The mixture was centrifuged for one minute at 5000 rpm and then mixed by vortex for three minutes after the addition of 2 ml of methyl tert-butyl ether. The mixture was centrifuged again for 10 min at 5000 rpm. Two milliliters of the organic layer was taken and allowed to dry under a stream of nitrogen gas. The residual was reconstituted in 0.5 ml of acetonitrile and water mixture and mixed using a vortex for two minutes, then centrifuged for 10 min at 5000 rpm (Ding et al., Citation2011). One-hundred milliliters of the supernatant was injected into HPLC system for drug analysis.
SIM analysis in human plasma
SIM acid was analyzed using HPLC Waters system consisting of auto-sampler, model no. 717 plus, binary HPLC pump, model no. 1525, Dual λ Absorbance, model no. 2487, Nova-Pak C18 3.9 × 150 mm Column (Waters, Milford, MA). The mobile phase was consisted of buffer solution of ammonium phosphate and acetonitrile in a ratio of 55:45 v/v. The mobile phase was freshly prepared on each day of analysis, filtered through 0.45-µm Millipore filter and degassed. The flow rate was adjusted at 1.2 ml/min, and UV detector was adjusted at 238 λ (Kang et al., Citation2004).
Statistical analysis
The results were presented as mean ± standard deviation (SD). Statistical analysis was performed using a Student’s t-test. The differences were considered significant for p < 0.05, and very significant for p < 0.01.
Results
The pharmacokinetic parameters were calculated from the data of analysis of SIM level in plasma samples and presented as mean ± SD. Maximum SIM concentration (Cmax) and the time to reach this maximum (Tmax) were determined. The total area under the plasma concentration-time curve (AUC0–∞, ng hr/ml), and the area under the first momentum curve (AUMC0-∞) up to the last time point were estimated based on the trapezoidal rule. The area of the tail was calculated using the plasma concentration at the last time point and the terminal elimination rate constant (k). The value of k was estimated from the least square regression analysis of the final segment of the curve. The mean residence time values were calculated as the ratio of the calculated AUMC0–∞ to AUC0–∞ in each case. Relative bioavailability was measured by comparing bioavailability of SIM-coated capsules with that of the commercial tablets (Zocor®).
shows CPX plasma concentration versus time curve for SIM-coated capsules compared with Zocor® tablets. SIM pharmacokinetics parameters after administration of two SIM-coated capsules (80 mg) and two Zocor® tablets (80 mg) are listed in .
Figure 1. Plasma concentration-time curve after oral administration of SIM-coated capsules and Zocor® tablets in human volunteers.
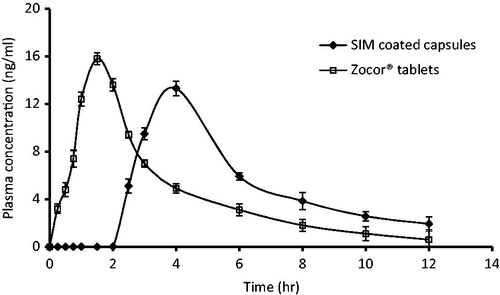
Table 1. Pharmacokinetic parameters after oral administration of SIM coated capsules and Zocor® tablets in human volunteers.
One-way ANOVA was performed to analyze the pharmacokinetic parameters calculated after administration of SIM formulations. Maximum plasma concentration (Cmax) was achieved (13.3 ± 0.9 ng/ml) from SIM-coated capsules compared with 15.8 ± 1.1 ng/ml Zocor® tablets.
Time to reach maximum concentration (Tmax) showed significant differences between the two tested SIM formulations (p > 0.05). Tmax values were 4 h for SIM-coated capsules, while it was 1.5 h for commercial tablets. The value of AUC0–∞ of SIM-coated capsules was found to be 71.4 ± 5.4 ngh/ml compared to 55.2 ± 4.1 ngh/ml for Zocor® tablets, which indicate improve of SIM-coated capsules bioavailability over the commercial tables. The value of the AUC0–∞ for SIM-coated capsules was significantly higher (p < 0.05) than that obtained for commercial SIM tablets.
Discussion
Lipid-based formulations, type IV, which contain surfactants and co-solvents may enhance the absorption rate and hence the bioavailability of lipophilic drugs particularly at low fluid content of the colon (Pouton, Citation2000, Citation2006; Barakat et al., Citation2011). These formulations facilitate the solubilization of drug by micellar formation, which reduces drug particle size and hence enhance its absorption rate. On the other hand, it was reported that these formulations have no effect on the bioavailability of lipophilic drugs. Charman et al. (Citation1992) reported that lipid-based formulations improved the reproducibility of the plasma profile in terms of Cmax and Tmax of the lipophilic compound WIN 54954, but there was no significant difference in the absolute bioavailability from the lipid-based formulations. In this study, incorporation of Cremophor RH 40 in type IV lipid-based formulation enhances SIM solubility in GIT content and hence its absorption rate; this could be due to the higher hydrophilic lipophilic balance (<16) and water solubility of Cremophor RH 40 (Memvanga et al., Citation2013; Jang et al., Citation2014).
On the other hand, presence of water insoluble surfactant like Capmul MCM EP (HLP < 6) in the formulation prevents SIM crystallization and precipitation in GIT. So, Capmul MCM EP improves SIM absorption rate and hence its bioavailability (Do et al., Citation2011). Furthermore, Capmul MCM EP could be entrapped in Cremophor RH 40, which increases the interfacial fluidity of Cremophor RH 40 and in colon content and enhances SIM solubility (Taha et al., Citation2004, Citation2007).
Furthermore, the incorporated amount of PEG 400 has the ability to improve water solubility of SIM in the formulation and hence its dissolution in GIT fluids (Tyagi et al., Citation2013). The quantity of PEG 400 must be adjusted in the formulation to prevent SIM precipitation. That is because PEG 400 has limited “on going” solubilization capacity after diluting the formulation in GIT fluids (Porter et al., Citation2008).
The value of AUC0–∞ of SIM-coated capsules confirmed a superior bioavailability over that of Zocor® tablets. SIM lipid-based coated capsules was found to significantly improve the bioavailability of SIM in comparison with Zocor® tablets (). Moreover, the tested formulation has the ability to significantly provide higher SIM plasma concentrations from 3 to 10 h after oral administration compared to Zocor® tablets.
In this study, Eudragit® S100 coat allows SIM capsules to withstand the acidic pH of the stomach, which could be indicated by the absorption lag time (up to 2.5 h) and significant higher Tmax value of 4 h compared to 1.5 h of Zocor® tablets (). It has been reported that Eudragit® S100 as coating material could be effective colon delivery for several drugs based on chronopharmaceutical considerations (Barakat et al., Citation2011; Soni et al., Citation2011). Some problems, such as shell softening and capsule sticking, may appear during aqueous dispersions coating process due to water solubility of gelatin capsules (Cole et al., Citation2002; Huyghebaert et al., Citation2014). So to eliminate these problems, SIM capsules were coated with HPMC solution prior to the final coating with Eudragit® S100 dispersion. Similar study had investigated the pulsatile release of tramadol hydrochloride for chronotherapeutic of arthritis utilizing HPMC pH-dependent polymer. Drug release was found to be increased by 15–30% in the presence of colonic microbial flora. It was observed that the lag time depends on the coating ratio of tramadol hydrochloride HPMC. The results show the capability of the system in achieving pulsatile release for a programmable period of time and pH-dependent release to attain colon-targeted delivery (Dabhi et al., Citation2010).
The results obtained in this study showed that SIM-coated capsules could be more efficient for treatment of hypercholesterolemia than commercially available SIM tablets. Time-controlled and site-specific drug delivery systems were found to be an effective approach as chronotherapy (Bussemer et al., Citation2001).
Conclusions
This investigation revealed that SIM lipid-based coated capsules could be a suitable formulation as time dependent delivery system for chronotherapeutic treatment of hypercholesterolemia. It also enhances the oral bioavailability of SIM over that of commercial product.
Declaration of interest
The author declares that there are no conflicts of interest. The author would like to thank the College of Pharmacy Research Center, King Saud University, for the financial support of this study.
References
- Ali J, Saigal N, Qureshi MJ, et al. (2010). Chronopharmaceutics: a promising drug delivery finding of the last two decades. Recent Pat Drug Deliv Formul 4:129–44
- Barakat NS, Al-Suwayeh SA, Taha EI, Bakry Yassin AE. (2011). A new pressure-controlled colon delivery capsule for chronotherapeutic treatment of nocturnal asthma. J Drug Targe 19:365–72
- Bussemer T, Otto I, Bodmeier R. (2001). Pulsatile drug-delivery systems. Crit Rev Ther Drug Carrier Syst 18:433–58
- Charman SA, Charman WN, Rogge MC, et al. (1992). Selfemulsifying drug delivery systems: formulation and biopharmaceutic evaluation of an investigational lipophilic compound. Pharm Res 9:87–93
- Chourasia MK, Jain SK. (2003). Pharmaceuticxal approaches to colon targeted drug delivery systems. J Pharm Pharmaceut Sci 6:33–66
- Cole ET, Scott RA, Connor AL, et al. (2002). Enteric coated HPMC capsules designed to achieve intestinal targeting. Int J Pharm 231:83–95
- Dabhi C, Randale S, Belgamwar V, et al. (2010). Predictable pulsatile release of tramadol hydrochloride for chronotherapeutics of arthritis. Drug Deliv 17:273–81
- De Oliveira HP, Albuquerque JJ Jr, Nogueiras C, Rieumont J. (2009). Physical chemistry behavior of enteric polymer in drug release systems. Int J Pharm 366:185–9
- Ding MJ, Yuan LH, Li Y, et al. (2011). Pharmacokinetics and bioequivalence study of simvastatin orally disintegrating tablets in Chinese healthy volunteers by LC-ESI-MS/MS. J Bioequiv Availab 3:032–7
- Do TT, Van Speybroeck M, Mols R, et al. (2011). The conflict between in vitro release studies in human biorelevant media and the in vivo exposure in rats of the lipophilic compound fenofibrate. Int J Pharm 414:118–24
- Ghimire M, McInnes FJ, Watson DG, et al. (2007). In-vitro/in-vivo correlation of pulsatile drug release from presscoated tablet formulations: a pharmacoscintigraphic study in the beagle dog. Eur J Pharm Biopharm 67:515–23
- Humberstone A, Charman WN. (1997). Lipid-based vehicles for the oral delivery of poorly water soluble drugs. Adv Drug Deliv Rev 25:103–28
- Huyghebaert N, Vermeire A, Remon JP. (2014). Alternative method for enteric coating of HPMC capsules resulting in ready-to-use enteric-coated capsules. Eur J Pharm Sci 21:617–23
- Jang DJ, Kim ST, Lee K, Oh E. (2014). Improved bioavailability and antiasthmatic efficacy of poorly soluble curcumin-solid dispersion granules obtained using fluid bed granulation. Biomed Mater Eng 24:413–29
- Kang BK, Lee JS, Chon SK, et al. (2004). Development of self-microemulsifying drug delivery systems (SMEDDS) for oral bioavailability enhancement of simvastatin in beagle dogs. Int J Pharm 274:65–73
- Kopecka J, Campia I, Olivero P, et al. (2011). A LDL-masked liposomal-doxorubicin reverses drug resistance in human cancer cells. J Control Release 149:196–205
- Liao JK. (2005). Clinical implications for statin pleiotropy. Curr Opin Lipidol 16:624–9
- Mandal AS, Biswas N, Karim KM, et al. (2010). Drug delivery system based on chronobiology – a review. J Control Release 147:314–25
- Mastiholimath VS, Dandagi PM, Jain SS, et al. (2007). Time and pH dependent colon specific, pulsatile delivery of theophylline for nocturnal asthma. Int J Pharm 328:49–56
- Memvanga PB, Eloy P, Gaigneaux EM, Préat V. (2013). In vitro lipolysis and intestinal transport of β-arteether-loaded lipid-based drug delivery systems. Pharm Res 30:2694–705
- Niwa K, Takaya T, Morimoto T, Takada K. (1995). Preparation and evaluation of a time-controlled release capsule made of ethylcellulose for colon delivery of drugs. J Drug Target 3:83–9
- Porter CJ, Pouton CW, Cuine JF, Charman WN. (2008). Enhancing intestinal drug solubilisation using lipid-based delivery systems. Adv Drug Deliv Rev 60:673–91
- Pouton CW. (2000). Lipid formulations for oral administration of drugs: non-emulsifying, self-emulsifying and ‘self-microemulsifying' drug delivery systems. Eur J Pharm Sci 11:S93–8
- Pouton CW. (2006). Formulation of poorly water-soluble drugs for oral administration: physicochemical and physiological issues and the lipid formulation classification system. Eur J Pharm Sci 29:278–87
- Pouton CW, Porter CJ. (2008). Formulation of lipid-based delivery systems for oral administration: materials, methods and strategies. Adv Drug Deliv Rev 60:625–37
- Sarasija S, Stutie P. (2005). Chronotherapeutics: emerging role of biorhythms in optimizing drug therapy. Indian J Phrm Sci 67:135–40
- Shabana MF, Mishriki AA, Issac MS, Bakhoum SW. (2013). Do MDR1 and SLCO1B1 polymorphisms influence the therapeutic response to atorvastatin? A study on a cohort of Egyptian patients with hypercholesterolemia. Mol Diagn Ther 5:299–309
- Smolensky MH, Peppas NA. (2007). Chronobiology, drug delivery, and chronotherapeutics. Adv Drug Deliv Rev 59:828–51
- Soni ML, Namdeo KP, Jain SK, et al. (2011). pH-enzyme di-dependent chronotherapeutic drug delivery system of theophylline for nocturnal asthma. Chem Pharm Bull (Tokyo) 59:191–5
- Stella VJ, Nti-Addae KW. (2007). Prodrug strategies to overcome poor water solubility. Adv Drug Deliv Rev 59:677–94
- Taha EI, Al-Saidan S, Samy AM, Khan MA. (2004). Preparation and in vitro characterization of self-nanoemulsified drug delivery system (SNEDDS) of all-trans-retinol acetate. Int J Pharm 285:109–19
- Taha EI, Ghorab DM, Zaghloul AA. (2007). Bioavailability assessment of vitamin A self nanoemulsified drug delivery systems in rats: a comparative study. Med Princ Pract 16:355–9
- Takaya T, Ikeda C, Imagawa N, et al. (1995). Development of a colon delivery capsule and the pharmacological activity of recombinant human granulocyte colony-stimulating factor (rhGCSF) in beagle dogs. J Pharm Pharmacol 47:474–8
- Tyagi VK, Singh D, Pathak K. (2013). Semisolid matrix-filled hard gelatin capsules for rapid dissolution of amlodipine besilate: development and assessment. J Adv Pharm Technol Res 4:42–9
- Ward S, Lloyd JM, Pandor A, et al. (2007). A systematic review and economic evaluation of statins for the prevention of coronary events. Health Technol Assess 11:1–178
- Yassin AE, Aodah AH, Al-Suwayeh S, Taha EI. (2012). Theophylline colon specific tablets for chronotherapeutic treatment of nocturnal asthma. Pharm Dev Technol 17:712–18
- Zhang Z, Bu H, Gao Z, et al. (2010). The characteristics and mechanism of simvastatin loaded lipid nanoparticles to increase oral bioavailability in rats. Int J Pharm 394:147–53