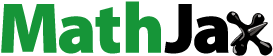
Abstract
Clinical application of ivermectin (IVM) is limited by several unfavorable properties, induced by its insolubility in water. Slight differences in formulation may change the plasma pharmacokinetics and efficacy. In this study, an IVM-loaded Soy phosphatidylcholine-sodium deoxycholate mixed micelles (IVM-SPC-SDC-MMs) were developed to improve its aqueous solubility, aiming to make it more applicable for clinical use. First, IVM-SPC-SDC-MMs were prepared using the co-precipitation method. After formulation optimization, the particle size was 9.46 ± 0.16 nm according to dynamic light scattering. The water solubility of IVM in SPC-SDC-MMs (4.79 ± 0.02 mg/mL) was improved by 1200-fold, comparing with free IVM (0.004 mg/mL). After subcutaneous administration, the pharmacokinetic study showed that IVM-SPC-SDC-MMs and commercially available IVM injection were bioequivalent. Also, the local irritation study confirmed that IVM-SPC-SDC-MMs reduced side reactions of the commercially available IVM injection. These results indicated that IVM-SPC-SDC-MMs represented a promising new drug formulation suitable for subcutaneous delivery of IVM.
Introduction
Ivermectin (IVM), produced by Streptomyces avermitilis, consists of over 80% of 22, 23-dihydroavermectin-B 1a and <20% of 22, 23-dihydroavermectin-B 1b (). As a potent antiparasitic agent, ivermectin was first marketed in 1981 by Merck Sharp and Dohme (Steel, Citation1993), which has remained highly active in the broad-spectrum antiparasitic therapy of onchocerciasis, lymphatic filariasis and scabies (Tagboto & Townson, Citation1996; Dourmishev et al., Citation1998). Ivermectin is available for oral, subcutaneous and topical administration, among which subcutaneous injection has been the most efficient route for ivermectin administration due to its higher bioavailability in sheep, cattle and goats (Alvinerie et al., Citation1998). Despite the promising biological effects of IVM, its clinical application is restricted by low aqueous solubility (4 µg/mL) (Lo et al., Citation1985).
To solve the problem of poor water solubility and to obtain an injectable formulation, a commercial injection of IVM has been produced with organic solvents. However, high concentration of organic solvents resulted in side reactions when applied in particular species. For instance, IVM-propylene glycol/glycerol or glycerin methylal/propylene glycol injection was reported to lead to pain and inflammation at the injection site (Frosch, Citation1995). In addition, IVM crystals were shown to precipitate at the injection site, which is dangerous to animals. Consequently, the application of these commercially available IVM injections has been greatly limited by their safety concerns.
To solve the aforementioned problems and optimize the pharmacological performance of IVM, formulation methods have been utilized (Lo et al., Citation1985; Hennessy, Citation1997; Liu et al., Citation2005; Bassissi et al., Citation2006; Ali et al., Citation2013). However, some of the reported results were far from ideal. For the subcutaneous administration of IVM in an aqueous vehicle, a micellar formulation containing high concentration of surface active agents such as Tween-80 was developed (Lo & Williams, Citation1983). However, it has been reported that Tween-80 may cause anaphylactic reaction, especially when used in dogs. Additionally, the presence of Tween-80 increased the concentration of histamine that resulted in anaphylaxis (Masini et al., Citation1985; Luo et al., Citation2010). Therefore, the micelle formulation which contains Tween-80 may cause extreme unsafety.
Based on its physiological compatibility and solubilizing capacity, phosphatidylcholine–bile salt-mixed micelles (PC-BS-MMs) was employed as the solubilizing vehicles to deliver IVM, which can be prepared by a simple co-precipitation method (Son & Alkan-Onyuksel, Citation1996; Hammad & Müller, Citation1998a,Citationb; Duan et al., Citation2011). Bile salts existed in the bile at a high concentration that can solubilize phosphatidylcholine to form a clear mixed micellar solution, which can in turn solubilize hydrophobic substances (Hammad & Müller, Citation1998b; Duan et al., Citation2011). Also, solubilized phospholipids in the carriers can help neutralize the hemolytic effects of bile salts (Salvioli et al., Citation1993; Narain et al., Citation1998; Moschetta et al., Citation2000). Most importantly, PC-BS-MMs have been found to turn out no embryotoxic, teratogenic or mutagenic effects after local administration (Teelmann et al., Citation1983). Without a solvent mixture, irritation could be reduced by PC-BS-MMs. Consequently, PC-BS-MMs could be an ideally subcutaneous delivery system of IVM.
Herein, the study aimed to develop a novel carrier system to deliver IVM for clinical application. First, ivermectin-soybean lecithin-sodium deoxycholate-mixed micelles (IVM-SPC-SDC-MMs) were prepared, optimized and characterized. The pharmacokinetic properties and local irritation at the injection site were further studied in rabbits in comparison with commercially available IVM injection.
Materials and methods
Reagents and chemicals
IVM was provided by the Hebei Weiyuan Animal Pharmaceutical Co., Ltd (Hebei, China). SPC was obtained from Lipoid GmbH (Ludwigshafen, Germany), purity 99%. SDC was purchased from Amresco Co., Ltd (America). Pyrene was supplied by Sigma-Aldrich (Munich, Germany). Reference substances of avermectin were provided by Solarbio (Beijing, China). The commercially available IVM injection (2 mg/mL, batch number: 100382647) was purchased from Nanjing Golden Shield Animal Pharmaceutical Co., Ltd. (Nanjing, China). Deionized (DI) water and other chemical reagents were of analytical grade.
Animals
New Zealand white rabbits weighting 2.0–2.6 kg were provided by the Special committee, Sichuan experimental animal farm (Chengdu, China). The rabbits were housed in standard rabbit cages in a temperature-controlled room at 27 ± 2 °C with a 12-h light/dark cycle. All animal procedures and protocols were approved by the Institutional Animal Care and Use Committee of Sichuan University.
Preparation of IVM-SPC-SDC-MMs
IVM was easily loaded into bile phospholipid micelle system using the co-precipitation method due to the good solubility of IVM, SPC and SDC in anhydrous ethanol (Son & Alkan-Onyuksel, Citation1996; Hammad & Müller, Citation1998a,Citationb; Duan et al., Citation2011). Simply, IVM, SDC and SPC with different mass ratios and concentrations were dissolved in anhydrous ethanol in a 100-mL round-bottom flask. After the anhydrous ethanol was evaporated completely at 45 °C under vacuum, a uniform thin film was formed and immediately rehydrated in DI water. To remove excess materials and unloaded drugs, the solution was filtered through 0.22-μm membrane filter. The final filtrate containing freshly formed MMs was collected.
Based on the single-factor method, a high-quality formulation was obtained through optimization of several main formulation factors, including the mass ratio of the mass concentration of SPC and SDC, the total concentration of SPC and SDC and the dose of IVM.
Critical micelle concentration determination
The pyrene 1:3 ratio method was used to determine the critical micelle concentration (CMC) value (Aguiar et al., Citation2003) of the binary mixture of SPC-SDC (the optimal ratio) in DI water. Different concentrations of MMs ranging from 1 × 10−4 to 4% were prepared. Then, pyrene was added to SPC-SDC-MMs with different concentrations to acquire a final concentration of 4 × 10−7 mol/L. After equilibrium for 24 h in dark at 25 °C, the mixtures were plated in 96-well plates and measured using Fluoroskan Ascent FL (Thermo Scientific, Waltham, MA). With an excitation wavelength of 336 nm and an emission slit of 5 nm, the intensities I1 and I3 were measured at the wavelengths near 372 and 383 nm corresponding to the first and third vibronic bands. All fluorescence measurements were carried out at 25 °C. The ratio of I1/I3 was analyzed with Origin 8.5 software (Origin Lab Corp, Northampton, MA).
Particle size measurement
Dynamic light scattering (DLS) (Malvern Zetasizer Nano ZS90, Malvern Instruments Ltd, Malvern, UK) was used to measure the particle size and polydispersity index (PDI) of the formed optimal mixed micelles at 25 °C
Transmission electron microscopy
The morphology of optimum IVM-SPC-SDC-MMs was observed with transmission electron microscope (TEM) (Tecnai G2 F20, FEI, Holland). The sample was placed on copper grids. After stained with 2% (w/v) phosphotungstic acid and dried naturally, it was subjected to TEM observation.
Drug solubility, solubilizing efficiency and drug loading of IVM-SPC-SDC MMs
The concentration of the IVM in the optimal IVM-SPC-SDC-MMs was determined by a UV spectrophotometer (Cary100, ELD5033098, VARIAN, California, USA). After multiple dilutions with DI water, solubilizing efficiency as well as drug loading of IVM-SPC-SDC-MMs subsequently was quantified by ultraviolet spectrophotometer at a wavelength of 245 nm. Thus, solubilizing efficiency and drug loading were calculated as follows:
(1)
(1)
(2)
(2)
Clarity and stability study
The IVM-SPC-SDC-MMs formulation and commercially available IVM injection were diluted by certain multiples (0-, 10-, 100-, 200- and 500-folds) with DI water. Briefly, each sample was placed in 10 ml tubes. Then, Type YB-II clarity detector widely used in China was applied to observed small insoluble particles that could not be dissolved in these formulations. Each sample was checked and contrasted with the standard (DI water). All samples were observed at room temperature and checked at fixed time points (0, 1, 2, 8, 12 and 24 h).
In vivo study in rabbits
Twelve New Zealand white rabbits (2.0–2.6 kg) were randomly allocated to two groups. Rabbits were fasted for 12 h with access to water before the experiment. IVM-SPC-SDC-MMs and commercially available IVM injection with an equivalent dose of IVM were subcutaneously injected at a dose of 5 mg/kg.
After administration, blood samples (1–2 mL) were collected from the ear veins with a medical catheter at predetermined time points (0, 1, 2, 6, 8, 10, 12, 24, 48, 72, 96, 120 and 144 h) and placed into heparinized centrifuge tubes. Plasma samples (0.5 mL) were collected immediately following centrifugation at 3500 rpm in an Allegra X-22 Centrifuge (Beckman Coulter Inc, Brea, CA) for 10 min. All Plasma samples were stored at −40 °C for pretreatment and high-performance liquid chromatography (HPLC) analysis.
Determination of IVM in plasma sample by HPLC
After a liquid–liquid phase extraction procedure, the concentration of IVM in plasma was analyzed by HPLC system (Agilent 1260 infinity, with a G1329 B autosampler, G1312 B pump and G1314 B detector; Agilent Technologies, Inc, Santa Clara, CA) with ultraviolet detection according to a previously described method (Zhang et al., Citation2011). The analysis was performed on a reverse-phase column (C18, 250 × 4.6 mm, 5 μm) with a security guard column (C18, 10 × 4 mm, 5 μm) (Phenomenex, Torrance, CA). The column temperature was maintained at 35 °C. The mobile phase consisted of a mixture of methanol and DI water (90:10). The flow rate was 1.0 mL/min and the detection wavelength was 245 nm.
Briefly, 0.5 mL of plasma sample was combined with 100 ng of internal standard and 1 mL of acetonitrile, followed by vortexing for 1 min. The mixture was centrifuged at 12 500 rpm for 10 min. Then, the supernatant was transferred to a 10 mL centrifuge tube, and 4 mL of ethyl acetate was added for extraction, which was repeated twice, all the supernatant liquid was collected in 15 mL tubes, dried at 50 °C with nitrogen, dissolved in 200 μL of mobile phase. Finally, 20 μL of the sample solution was injected into the reverse HPLC system for quantitative study.
Using afore-mentioned HPLC method condition, the linear standard curve showed good linearity over the concentration range of 20–1000 ng/mL. The standard curve equation of IVM in plasma was: y = 3.3545x + 0.1903 (r = 0.9994). Where y stands for the concentration of IVM, x stands for the peak area ratio of IVM to internal standard and r corresponds to the correlation coefficient. The recovery rates of IVM from high, middle and low concentrations were 109.6% ± 0.97%, 113.6% ± 3.2% and 95.4% ± 6.5%, respectively. The relative standard deviations of between-day were 6.90%, 1.70% and 2.30%, whereas the relative standard deviations of within-day were 0.80%, 2.90% and 10.10%, respectively. This method was then applied to the pharmacokinetic studies of IVM in rabbits.
Local irritation at injection site in rabbits
Twelve New Zealand white rabbits with body weight ranging from 2.1 to 2.6 kg of both sexes were randomly divided into two groups with free diet before experiment. Self-control method was adopted in the experiment (Draize et al., Citation1944). The first group was subcutaneously given commercially available IVM injection and sodium chloride injection, the second group was subcutaneously administered with IVM-SPC-SDC-MMs and sodium chloride injection. The standard approach includes using a single dose of the test material (0.5 mL or 0.5 g) to a small area of the shaved skin of rabbits (Draize et al., Citation1944). Briefly, the hairs of all rabbits were shaved 24 h before injection. Then, the first group was administered with the IVM injection on the left and sodium chloride injection on the right. The animals in the second group were injected with IVM-SPC-SDC-MMs on the right and sodium chloride injection on the left. The injection site reactions was visually observed and recorded as pathological samples at intervals until fourth and 14th days. On Day 4, three animals were sacrificed in each group. On Day 14, the rest of the six animals were sacrificed. The obtained samples were washed immediately with saline and fixed in 4% paraformaldehyde for 48 h (Song et al., Citation2011). After dehydration, the samples were embedded in paraffin, cut into tissue sections and stained using hematoxylin and eosin. All prepared sections were observed at ×100 magnification with an Axiovert 200 (Zeiss, Oberkochen, Germany) for pathological analysis and evaluation.
Statistical analysis
All experiments were repeated at least three times. The Drug and Statistics Software (DAS 2.0, Mathematical Pharmacology Professional Committee of China) was employed to analyze pharmacokinetic data. The best compartment model for evaluating pharmacokinetic parameters was then estimated. Student’s t-test was performed to compare differences between two treatment groups, and a p value <0.05 was considered as statistically different.
Results
Determination of solubilizing efficiency of IVM-SPC-SDC-MMs in different formulations
The main consideration of the optimal formulation was the mass ratio of SPC/SDC, the total concentration of SPC and SDC and dosage.
First, the influence of the mass ratio of SPC and SDC on solubilizing efficiency of IVM-SPC-SDC-MMs (IVM concentration was kept at 5 mg/mL) was shown in . When the mass ratio of SPC and SDC decreased from 1:1 to 1:1.4, a significant increase in IVM solubilizing efficiency was observed. However, a further decrease of the mass ratio of SPC and SDC (from 1:1.4 to 1:2) slightly reduced IVM solubilizing efficiency. Hence, the SPC/SDC mass concentration ratio of 1:1.4, the solubilizing efficiency reached 94% was selected for following studies.
Figure 2. Influence of the mass concentration ratio of SPC and SDC on IVM solubilizing efficiency at 25°C (N = 3).
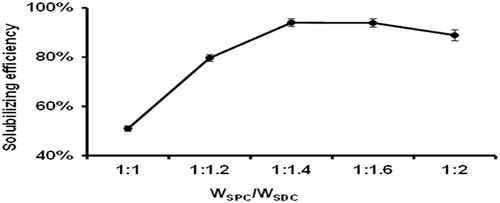
When the mass concentration ratio of IVM was fixed at 5 mg/mL, we also investigated the impact of total concentration of SPC and SDC, as shown in . When the total concentration of SPC and SDC was 72 mg/mL, IVM solubilizing efficiency reached the maximum. Moreover, when the total concentration of SPC and SDC was >72 mg/mL, the solubilizing efficiency did not increase anymore.
Figure 3. Influence of the total concentration of SPC and SDC on IVM solubilizing efficiency at 25°C (N = 3).
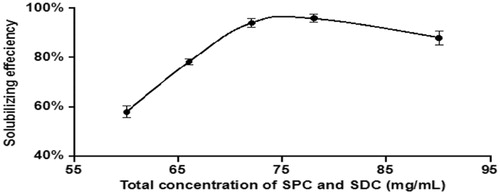
The effect of the concentration of IVM on the stability of SPC-SDC-MMs was shown in . The results showed that IVM concentration affects the stability of the IVM-SPC-SDC-MMs. The concentration limit of IVM in the optimized SPC-SDC-MM system was around 5 mg/mL. Just as the result, further increases in the concentration of IVM resulted in the instability of IVM-SPC-SDC-MMs, as confirmed by the separation of IVM from the formulation.
Table 1. Influence of IVM concentration on the stability of IVM-SPC-SDC-MMs.
CMC determination
According to previous studies (Amiji, Citation1995; Aguiar et al., Citation2003; Quagliotto et al., Citation2009), the CMC value can be obtained from two singular points in the pyrene 1:3 ratio plots, which can be described by a decreasing sigmoid of the Boltzmann type (), as shown in the following equation:
where y is the pyrene 1:3 ratio, the independent variable χ represents the total concentration of surfactant. A1 and A2 correspond to the upper and lower limits of the sigmoid; χ0 is the midpoint of the curve and Δχ corresponds to the independent variable range. In , the shape of the plot displayed a typical sigmoid decrease. According to the Boltzmann-type equation, the values for A1, A2, χ0, and Δχ were 1.10, 0.89, −2.24 and 0.58, respectively; R2 = 0.92, indicated that the Boltzmann-type model confirmed the typically sigmoidal decrease of the plot. As the value of χ0/Δχ < 10, χ0 was considered as the CMC value (Aguiar et al., Citation2003). Consequently, the CMC value of SPC/SDC (1:1.4) was 0.013 mg/mL.
Drug solubility, solubilizing efficiency and drug loading of IVM-SPC-SDC-MMs
The solubility of IVM in water was about 0.004 mg/mL. However, after formation of IVM-SPC-SDC-MMs, the solubility was significantly increased to 4.79 ± 0.02 mg/mL. As shown in , the solubilizing efficiency and drug loading of IVM-SPC-SDC-MMs were 94% ± 2.8% and 6.22% ± 0.26%, respectively.
Table 2. Solubilizing efficiency and drug loading of IVM-SPC-SDC-MMs.
Particle size and morphology
The mean size of the optimal IVM-SPC-SDC-MMs was 9.46 ± 0.16 nm with a polydispersity index of 0.192 ± 0.018 (). It suggested that the micelle formulation was homogeneous and monodisperse. In addition, the TEM study showed that the IVM-SPC-SDC-MMs were spherical in shape with an average particle size ∼9 nm ().
Study in clarity and stability of the formulations
Clarity of these two formulations was observed after dilutions at predetermined time points. The MMs formulation remained clear and transparent in the interval time at any degree of after dilutions. In contrast, IVM injection turned cloudy immediately only after 10 times dilution of commercially available IVM injection; and crystals immediately appeared after 100-fold dilutions.
In vivo study in rabbits
The mean plasma concentration–time curves of IVM after subcutaneous administration of IVM injection and IVM-SPC-SDC-MMs to rabbits (n = 5) are shown in . The results indicated that IVM-SPC-SDC-MMs and IVM injection basically shared the same trend. Before reaching Cmax at 24 h, the concentration of IVM in both groups experienced a continuous increase. (The break release effect around 8 h could be probably caused by enterohepatic circulation and animal individual differences (Ali & Hennessy, Citation1996; González Canga et al., Citation2009)). After 24 h, IVM was slowly eliminated from the blood till 144 h, when the concentration of IVM was at the minimum and then could not be detected. The main pharmacokinetic parameters are shown in . The AUC(0–∞), Cmax, MRT, t1/2z and CLz of IVM between the IVM-SPC-SDC-MMs group and IVM injection group showed no significant differences (p > 0.05), demonstrating that the two formulations were bioequivalent.
Figure 6. Mean plasma concentration–time curve of IVM after subcutaneous administration of commercially available IVM injection and IVM-SPC-SDC-MMs in rabbits. Data represent mean ± standard deviation (N = 5).
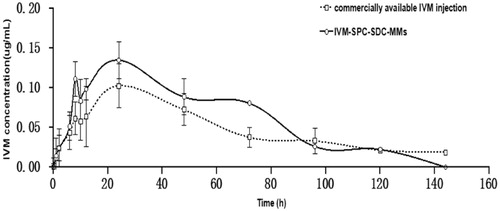
Table 3. The main pharmacokinetic parameters of IVM obtained after subcutaneous administration of the commercially available IVM injection and the IVM-SPC-SDC-MMs formulation at a dose of 5.0 mg/mL in rabbits.
Local irritation at the injection site in rabbits
The local irritation test at the injection site after single subcutaneous injection was conducted to evaluate the irritation of IVM-SPC-SDC-MMs and sodium chloride injection versus IVM injection. All tested animals displayed normal behavior following administration. Compared with the IVM injection group, the IVM-SPC-SDC-MMs formulation-treated rabbit did not show intense struggle. After HE staining, differences were observed at the injection site, especially on Day 14 (). On Day 4, IVM injection-treated group displayed mild inflammatory cell infiltration and hemorrhage (). On Day 14, severer inflammatory response and hemorrhage were observed () together with crystal formations at the injection site (). In contrast, no inflammation reaction and crystallization were observed on Days 4 and 14 for the IVM-SPC-SDC-MMs group (), the results of which were comparable with the sodium chloride group ().
Figure 7. Pathological paraffin sections from local injection site of rabbits after injection on the Days 4 and 14 (magnification, ×100). (A), (B) and (C) Skin samples from rabbits given the commercially available IVM injection. (A) Small amount of inflammatory cell infiltration was shown at the injection site on the fourth day. (B), (C) Until 14th day, severer inflammation and hemorrhage appeared and crystals were also observed. (D), (E) Skin samples from rabbits administered IVM-SPC-SDC-MMs on fourth and 14th days, respectively. (F) Skin sample from rabbits given sodium chloride injection. The phenomenon shown in (A), (B) and (C) were not observed in (D), (E) or (F).
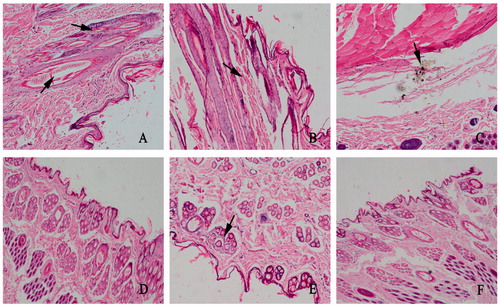
Discussion
One of the main goals of modern antiparasite chemotherapy is to optimize drugs to the pathogen and minimize side effects by innovative formulations to enhance the antihelmintic efficacy (Bassissi et al., Citation2006). Studies showed that the PC-BC-MMs system had great potential to improve the solubility of poorly soluble drug, particularly the lipophilic drugs. The effect of the types of BS and PC was shown in agreement with the results of Alkan-Onyuksel and Son (Citation1992) for solubilizing teniposide. The solubilizing capacity of BS-SPC-MMs was proven better than that of BS-EPC-MMs, which may be attributed to the fatty acid of SPC with longer chain lengths (Hammad & Müller, Citation1998a,Citationb). With respect to bile salts, compared with SGC, SDC alone or in mixed micelle with PC displayed a higher solubilizing capacity for diazepam (Hammad & Müller, Citation1998a). This observation has also been reported for other drugs such as diazepam (Alkan-Onyuksel et al., Citation1994). With optimized IVM-SPC-SDC-MMs, the solubility of IVM was significantly improved and reached 4.79 ± 0.02 mg/mL, which was about 1200-fold higher than that of free IVM in water (4 µg/mL). Thus, IVM-SPC-SDC-MMs were proven a promising carrier for improving the solubility of IVM in water.
In order to optimize the IVM-SPC-SDC-MMs formulation, the SPC/SDC mass concentration, the dose of IVM and the total concentration of SPC and SDC were studied, respectively.
Moreover, solubilizing efficiency, and clarity of formulation were employed as evaluation parameters for optimizing the formulation. The highest solubilizing efficiency was obtained when the mass concentration ratio of SPC to SDC was 1:1.4. However, a further decrease in the mass concentration ratio from 1:1 to 1:2 led to remarkable reductions in solubilizing efficiency and drug loading. The presence of excessive SPC greatly enhanced the capacity of IVM-SPC-SDC-MMs to solubilize IVM (Hammad & Müller, Citation1998a). Thus, a significant increase in IVM solubilizing efficiency was expected when the SPC/SDC mass ratio increased from 1:1 to 1:1.6. However, previous studies reported that an increase in the SPC concentration resulted in a higher viscosity and less partition of drug into the mixed micellar space (Duan et al., Citation2011).
In addition, the total concentration of SPC and SDC was another important factor affecting the solubilizing capacity of the micelle formulation. Increasing the total concentration of SPC and SDC, the amount of single micelles increased and the stability of IVM was thus improved. When the total concentration of SPC and SDC reached 72 mg/mL, IVM solubilizing efficiency reached the maximum. However, the solubilizing efficiency of such MMs did not increase anymore with addition of extra SPC and SDC after reaching its maximum solubilizing capacity. Besides, to avoid adding excessive excipients, a lower total concentration of SPC and SDC were selected.
The formation of drug-loaded micelles is associated with the physicochemical properties of the drug, including polarity, polarization degree, chain length, chain branching, molecular size, shape and structure (Attwood & Florence, Citation1983; Hammad & Müller, Citation1998a). According to the systematic research, the structure of the PC-BS-MMs is generally considered to be a “mixed disk model” (Mazer et al., Citation1980). In brief, the mixed micelle consists of a disk-like core in which the phospholipid bilayer was surrounded by BS molecules at its perimeter. The aforementioned model corresponded with our results. When the mass concentration of SPC increased, the IVM solubility increased. In the optimized SPC-SDC-MMs system, the limiting concentration of IVM was 4.79 ± 0.02 mg/mL. When the concentration of IVM was above the critical value, IVM may no longer remain stable, but precipitate out of the MMs system gradually. This may be due to the presence of oxygen atoms in the IVM structure, which can easily form hydrogen bonds with water.
The pyrene 1:3 ratio method has been one of the most popular methods for the determination of CMC values of both pure micelle solution as well as mixed micelles (Amiji, Citation1995; Aguiar et al., Citation2003; Quagliotto et al., Citation2009). Pyrene, as a hydrophobic molecule, was very sensitive in a hydrophobic environment (Amiji, Citation1995). Initially, the I1/I3 ratio decreased significantly, illustrating the pyrene was encapsulated in micelles, which resulted in a hydrophilic environment with low surfactant concentration (Ménard et al., Citation2012). When the I1/I3 ratio kept decreasing till reaching the steady state, pyrene was exposed in a hydrophobic environment with high surfactant concentrations (Song et al., Citation2011).
Study in clarity and stability of the MMs was performed to evaluate the safety of the IVM-SPC-SDC-MMs for clinical use (Lazarewicz et al., Citation1989). As is shown in the results, when the commercially available IVM injection was diluted to a certain concentration, due to the reduction of the organic solvent proportion, IVM crystal appeared immediately, which is disadvantageous in clinical application. In contrast, after the formation of the IVM-SPC-SDC-MMs, IVM was encapsulated in the micelle hydrophobic core. Herein, the formulation was relatively stable when the IVM-SPC-SDC-MMs were diluted. In the experiment, When the IVM-SPC-SDC-MMs was diluted 500-fold wherein concentration of SPC and SDC was 0.14 mg/mL, which is much higher than CMC (0.013 mg/mL); the IVM crystal was not observed, suggesting IVM-SPC-SDC-MMs formulation is more safe for clinical use than commercially available IVM injection with the organic solvent.
This article reports the first study in rabbits on the comparative pharmacokinetics after subcutaneous administration of IVM injection and IVM-SPC-DOC-MMs. No significant differences were found in the primary pharmacokinetic parameters of the two formulations. Previous studies have illustrated that the pharmacokinetic behavior of endectocides was significantly affected by the delivery vehicles, which could influence drug absorption, disposition (Lo et al., Citation1985, Wicks et al., Citation1993) and targeting efficiency (Lifschitz et al., Citation2000). Subcutaneous injection of macromolecules and particles were known to be absorbed into local lymphatics first before entering the systemic circulation (Leak, Citation1971); then, these substances passed through the chain of lymphatic node, lymphatic trunks into the blood. This suggested that SPC-SDC-MMs containing IVM could reach the bloodstream by the lymphatic pathway, which depended on the lymph node chains that absorbed the drug from the injection site. Also, the IVM injection demonstrated a slow and prolonged process of absorption due to the precipitation of the drug at the site of injection (Bassissi et al., Citation2006). Despite the difference in the absorption process, the two formulations shared similar pharmacokinetic profiles in rabbits.
In local irritation study, severe local irritation was observed when the commercially available IVM injection was applied in antiparasite. The experimental rabbits struggled frequently with administration of commercially available IVM injection. However, the reaction above did not appear in both IVM-SPC-SDC-MMs formulation and control groups. The result is likely due to the presence of high concentrations of organic solvents such as propylene glycol/glycerol or glycerin methylal/propylene glycol, contained in IVM injection (Tompkins et al. Citation2010). The high concentration of organic solvents was reported to result in great pain and more intense struggles in rabbits (Tompkins et al., Citation2010). For the IVM injection group, severe local irritations indicated by inflammatory response and drug precipitation were observed microscopically. These suggested that commercially available IVM injection not only led to great agony but also arouse strong inflammatory response. In comparison, no obvious irritation was observed in the control group and IVM-SPC-SDC-MMs after single subcutaneous administration, indicating that IVM-SPC-SDC-MMs could be a safe and reliable formulation for clinical application.
To sum up, IVM-SPC-SDC-MMs were successfully prepared using a simple co-precipitation method. After optimization of the formulation, IVM-SPC-SDC-MMs exhibited suitable particle size with sufficient solubilizing capacity for IVM. IVM-SPC-SDC-MMs and IVM injection exhibited comparable pharmacokinetic behavior in rabbits. Moreover, IVM-SPC-SDC-MMs did not induce local irritation at the injection site, solving a problem commonly seen in the application of IVM injection. Thus, IVM-SPC-SDC-MMs were proven an attractive formulation for subcutaneous administration of IVM. With remarkable improvement in the water solubility of IVM and safety, IVM-SPC-SDC-MMs gain the potential to be a promising replacement for IVM injection which contains organic solvents. In addition, IVM-SPC-SDC-MMs could be prepared by a simple and reproducible method, thus having the possibility for large-scale industrial production.
Declaration of interest
The authors report no conflicts of interest. The authors alone are responsible for the content and writing of this article.
This research was supported by the National Basic Research Program of China (973 program, no. 2013CB932504) and the National S & T Major Project of China (grant no. 2012ZX09304004001).
References
- Aguiar J, Carpena P, Molina-BolıVar J, Carnero Ruiz C. (2003). On the determination of the critical micelle concentration by the pyrene 1: 3 ratio method. J Colloid Interf Sci 258:116–22
- Ali D, Hennessy D. (1996). The effect of level of feed intake on the pharmacokinetic disposition and efficacy of ivermectin in sheep. J Vet Pharmacol Ther 19:89–94
- Ali M, Afzal M, Verma M, et al. (2013). Improved antifilarial activity of ivermectin in chitosan–alginate nanoparticles against human lymphatic filarial parasite, Brugia malayi. Parasitol Res 112:2933–43
- Alkan-Onyuksel H, Ramakrishnan S, Chai H-B, Pezzuto JM. (1994). A mixed micellar formulation suitable for the parenteral administration of taxol. Pharmaceut Res 11:206–12
- Alkan-Onyuksel H, Son K. (1992). Mixed micelles as proliposomes for the solubilization of teniposide. Pharmaceut Res 9:1556–62
- Alvinerie M, Escudero E, Sutra J-F, et al. (1998). The pharmacokinetics of moxidectin after oral and subcutaneous administration to sheep. Vet Res 29:113–18
- Amiji MM. (1995). Pyrene fluorescence study of chitosan self-association in aqueous solution. Carbohyd Polym 26:211–13
- Attwood D, Florence A. (1983). Surfactant systems: their chemistry, pharmacy and biology. London: Chapman & Hall
- Bassissi F, Lespine A, Alvinerie M. (2006). Assessment of a liposomal formulation of ivermectin in rabbit after a single subcutaneous administration. Parasitol Res 98:244–9
- Dourmishev A, Serafimova D, Dourmishev L. (1998). Efficacy and tolerance of oral ivermectin in scabies. J Eur Acad Dermatol 11:247–51
- Draize JH, Woodard G, Calvery HO. (1944). Methods for the study of irritation and toxicity of substances applied topically to the skin and mucous membranes. J Pharmacol Exp Ther 82:377–90
- Duan RL, Sun X, Liu J, et al. (2011). Mixed micelles loaded with silybin-polyene phosphatidylcholine complex improve drug solubility. Acta Pharm Sinic 32:108–15
- Frosch PJ. (1995). Cutaneous irritation. Textbook of contact dermatitis. Berlin, Germany: Springer
- González Canga A, Sahag N Prieto AM, Jos Diez Li Bana M, et al. (2009). The pharmacokinetics and metabolism of ivermectin in domestic animal species. Vet J 179:25–37
- Hammad M, Müller B. (1998a). Increasing drug solubility by means of bile salt–phosphatidylcholine-based mixed micelles. Eur J Pharm Biopharm 46:361–7
- Hammad M, Müller B. (1998b). Solubility and stability of tetrazepam in mixed micelles. Eur J Pharmaceut Sci 7:49–55
- Hennessy D. (1997). Modifying the formulation or delivery mechanism to increase the activity of anthelmintic compounds. Vet Parasitol 72:367–90
- Lazarewicz JW, Pluta R, Salinska E, Puka M. (1989). Beneficial effect of nimodipine on metabolic and functional disturbances in rabbit hippocampus following complete cerebral ischemia. Stroke 20:70–7
- Leak LV. (1971). Studies on the permeability of lymphatic capillaries. J Cell Biol 50:300–23
- Lifschitz A, Virkel G, Sallovitz J, et al. (2000). Comparative distribution of ivermectin and doramectin to parasite location tissues in cattle. Vet Parasitol 87:327–38
- Liu X, Sun Q, Wang H, et al. (2005). Microspheres of corn protein, zein, for an ivermectin drug delivery system. Biomaterials 26:109–15
- Lo P-KA, Fink DW, Williams JB, Blodinger J. (1985). Pharmacokinetic studies of ivermectin: effects of formulation. Vet Res Commun 9:251–68
- Lo PKA, Williams JB. (1983). Solubilization of ivermectin in water. Google Patents
- Luo X, Wang Q, Zhou L, et al. (2010). Study on relationship between Tween-80 contained in injections of Chinese herbal medicine and anaphylactic reactions. Adv Drug Reactions J 12:160–5
- Ménard N, Tsapis N, Poirier C, et al. (2012). Drug solubilization and in vitro toxicity evaluation of lipoamino acid surfactants. Int J Pharmaceut 423:312–20
- Masini E, Planchenault J, Pezziardi F, et al. (1985). Histamine-releasing properties of Polysorbate 80in vitro andin vivo: correlation with its hypotensive action in the dog. Agents Actions 16:470–7
- Mazer NA, Benedek GB Carey MC. (1980). Quasielastic light-scattering studies of aqueous biliary lipid systems. Mixed micelle formation in bile salt-lecithin solutions. Biochemistry 19:601–15
- Moschetta A, Portincasa P, Palasciano G, et al. (2000). Sphingomyelin exhibits greatly enhanced protection compared with egg yolk phosphatidylcholine against detergent bile salts. J Lipid Res 41:916–24
- Narain P, Demaria E, Heuman D. (1998). Lecithin protects against plasma membrane disruption by bile salts. J Surg Res 78:131–6
- Quagliotto P, Barbero N, Barolo C, et al. (2009). Characterization of monomeric and gemini cationic amphiphilic molecules by fluorescence intensity and anisotropy. Dyes Pigments 82:124–9
- Salvioli G, Gaetti E, Panini R, et al. (1993). Different resistance of mammalian red blood cells to hemolysis by bile salts. Lipids 28:999–1003
- Son K, Alkan-Onyuksel H. (1996). Physical stability of teniposide in bile salt-egg phosphatidylcholine mixed micelles and liposomes. PDA J Pharm Sci Tech 50:89–93
- Song X, Jiang Y, Ren C, et al. (2011). Nimodipine-loaded mixed micelles: formulation, compatibility, pharmacokinetics, and vascular irritability study. Int J Nanomed 7:3689–99
- Steel J. (1993). Pharmacokinetics and metabolism of avermectins in livestock. Vet Parasitol 48:45–57
- Tagboto S, Townson S. (1996). Onchocerca volvulus and O. lienalis: the microfilaricidal activity of moxidectin compared with that of ivermectin in vitro and in vivo. Ann Tropic Med Parasitol 90:497–505
- Teelmann K, Schl Ppi B, Sch Pbach M, Kistler A. (1983). Preclinical safety evaluation of intravenously administered mixed micelles. Arzneimittel-Forschung 34:1517–23
- Tompkins J, Stitt L, Ardelli B. (2010). Brugia malayi: in vitro effects of ivermectin and moxidectin on adults and microfilariae. Exper Parasitol 124:394–402
- Wicks S, Kaye B, Weatherley A, et al. (1993). Effect of formulation on the pharmacokinetics and efficacy of doramectin. Vet Parasitol 49:17–26
- Zhang, R.-L., Cui, L.-L., Hao, Z.-H, Zhao, Y.-D. 2011. Compare Pharmacokinetics of 5 Different Ivermectin Injection in Rabbit. China Animal Husbandry & Veterinary Medicine, 38(11):188–90