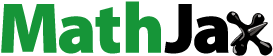
Abstract
Objective: To explore the potential of non-ionic surfactants as novel intranasal absorption enhancers.
Methods: Taking sumatriptan succinate (SMS) as a model drug, influence of different non-ionic surfactants, including laurate sucrose ester (SE), cremophor EL and poloxamer 188, on the intranasal absorption of SMS was investigated using an in situ nasal perfusion technique in rats. Ciliotoxicity of the non-ionic surfactants was evaluated using an in situ toad palate model. In vivo behavior of the selected formulations was studied in rats.
Results: All the non-ionic surfactants investigated increased the intranasal absorption of SMS remarkably but with varied extent and trend. Moreover, it was revealed that at the same concentration, laurate SE had better permeation-enhancing effect than that of cremophor EL and poloxamer 188. The ciliotoxicity results showed that all the non-ionic surfactants were regarded as safe at selected concentrations. Based on the in situ absorption data and ciliotoxicity results, the following three samples, 0.5% laurate SE, 0.1% cremophor EL and 0.5% poloxamer 188 were selected for in vivo absorption studies in rats. Among them, 0.5% laurate SE group presented the highest enhancing effect, followed by 0.1% cremophor EL and 0.5% poloxamer 188 group, with absolute bioavailability 29.99%, 22.64% and 20.90%, respectively.
Conclusions: Laurate SE is a promising intranasal absorption enhancer.
Introduction
Recently, nasal administration has drawn significant attention as an alternative route for systemic delivery of many drugs due to its following advantages, including rapid onset of action comparable to injection, large surface area of nasal mucosa (approximately 150 cm2), lower enzymatic activity compared with gastrointestinal tract and highly vascularized subepithelial layer which passes the drug directly into the systemic circulation, avoiding the first pass metabolism in the liver (Ding et al., Citation2012). Moreover, after nasal administration, part of the drug can directly get into the brain tissue or cerebrospinal fluid through olfactory neurons (Duan & Mao, Citation2010; Yu et al., Citation2011; Ding et al., in press), which could provide better therapy for central nerves system-related diseases.
However, there are two main barriers limiting the intranasal absorption of drugs. One is the low membrane permeability of polar drugs, the other is rapid clearance of administered formulation from nasal cavity owing to mucociliary clearance (Illum, Citation2012). During the past decades, great efforts have been made to overcome the absorption barriers. Among them, searching for safe and effective absorption enhancers is highly desired.
Poloxamer 188, a non-ionic surfactant, has been widely used in the intranasal drug delivery system with different functions. For example, it has been used as the carrier of in situ forming gels, and pharmacokinetic study in animals showed significant improvement in bioavailability of the drug from intranasal gels than oral solution (Jose et al., Citation2013; Ravi et al., Citation2013; Ozbılgın et al., Citation2014). Incorporation of poloxamer 188 was reported to be able to influence the elasticity of nanocubic vehicles for intranasal delivery (Salama et al., Citation2012). Poloxamer: PLGA mixture-based DNA nanoparticles have a potential as carriers for the delivery of DNA across the nasal mucosa (Csaba et al., Citation2006). Our previous study demonstrated that poloxamer 188 played a key role in promoting the intranasal absorption of isosorbide dinitrate (Na et al., Citation2010). Therefore, our assumption is that some other non-ionic surfactants may also be explored as intranasal absorption enhancers with good safety profile. To test this hypothesis, two other non-ionic surfactants, cremophor EL and laurate sucrose ester (SE), were selected in this study. Cremophor EL has been used as excipient in infusions such as Taxol, in oral gelatin capsules such as Ritonavir, as well as in oral solutions (Strickley, Citation2004), it was reported to be able to open tight junctions in Caco-2 cells (Udata et al., Citation2003). Laurate SE is a non-ionic surfactant consisting of sucrose as the hydrophilic group and lauric as the lipophilic group, with HLB 16. It can be used as emulsifier, antibacterial agent and crystallization inhibitor. Its analogue, sucrose cocoate, has been reported as the permeation enhancer of insulin, a rapid and significant increase in plasma insulin level was found after intranasal administration of an insulin formulation containing 0.5% sucrose cocoate, with a concomitant decrease in blood glucose level (Ahsan et al., Citation2003).
Sumatriptan succinate (SMS) belongs to biopharmaceutical classification system (BCS) class III with poor permeability. It is a selective 5-HT1 receptor agonist and is widely used in the treatment of migraine. Its oral bioavailability is quite low (15%) due to high first-pass metabolism (Femenia-Font et al., Citation2005), making oral treatment unsatisfactory (Pierce, Citation2010). Moreover, a substantial proportion of patients suffer from severe nausea or vomiting during their migraine attack, thus intranasal delivery seems to be a possible way to solve this problem. At present, although sumatriptan nasal spray formulation is commercially available in the market, the bioavailability is still quite low due to its poor permeability. In order to further increase its intranasal absorption, recently an in situ mucoadhesive gel was reported to improve the nasal retention time of SMS but no in vivo data were presented (Badgujar et al., Citation2010).
Thus, taking SMS as a model drug, the objective of this study was to explore non-ionic surfactants as potential intranasal absorption enhancers, including laurate SE, cremophor EL and poloxamler 188. Influence of the above-mentioned non-ionic surfactants on the intranasal absorption of SMS was investigated using an in situ nasal perfusion technique in rats. Ciliotoxicity of different non-ionic surfactants at selected concentrations were compared using an in situ toad palate model. Moreover, based on the in situ results, the selected intranasal formulations were evaluated in vivo in rats. To the best of our knowledge, this is the first time that laurate SE and cremophor EL were explored as intranasal absorption enhancers systemically.
Materials and methods
Materials
SMS was purchased from Beijing Mediking Biopharm Co., Ltd (Beijing, China). Laurate SE of food grade was purchased from Mitsubishi Kagaku Foods Co. (Japan). Poloxamer 188 and cremophor EL of pharmaceutical grade were gifts from BASF (Germany). Methanol and acetonitrile of chromatographic grade were purchased from Shangdong Yuwang Tech Reagent Company (Shandong, China). All other chemicals were of analytical grade.
Preparation of intranasal formulations
Laurate SE, cremophor EL and poloxamer 188 were dissolved in distilled water to obtain the required concentration as specified in in situ test. SMS was dissolved in the above-mentioned solution (0.1 mgml−1) and pH of the solution was adjusted as required. As for formulations used for in vivo studies, they were the same as that for in situ study, and the dose of SMS was 12 mgkg−1 to rats. Intravenous injection solution was prepared by dissolving SMS (10 mgml−1) in sterile saline solution and adjusting pH to 5.5.
In vitro analytical method of SMS
The concentration of SMS was analyzed by HPLC (Patel et al., Citation2007). HPLC apparatus (LC6-AS liquid chromatograph, Shimadzu, Kyoto, Japan) connected to an ultraviolet variable wavelength detector (Model SPD-6A, Shimadzu, Kyoto, Japan) with a C-18 reversed phase column (4.0 mm internal diameter, 25 cm in length and with a 5 μm particle size) (Agilent Technologies, Palo Alto, CA) and isocratic pump (Model LC6-AS, Shimadzu, Japan) were used. The mobile phase (20% acetonitrile and 80% 0.025 M NH4H2PO4 buffered to pH 5.00 with 0.1% Triethylamine) was delivered at a flow rate of 1.0 mlmin−1, with oven temperature 35 °C. The injection volume was 20 μl and the sample was analyzed at 282 nm. The limits of detection and quantitation of SMS were 0.08 μg ml−1 and 0.25 μg ml−1, respectively. A linear relationship between peak area and concentration was found in the concentration range of 0.5–60 μg ml−1 (A = 5956.7C + 700.08, R = 0.9999, n = 8). The inter-day relative standard deviations were less than 2% (n = 5).
In situ nasal perfusion studies in rats
The animal experiment was carried out in accordance with the Principles of Laboratory Animal Care (NIH Publication No. 86-23, revised 1985). Sprague-Dawley male rats (seven weeks old, 230 ± 20 g) were supplied by the Lab Animal Center of Shenyang Pharmaceutical University (Grade II, Certificate No. SYXK 2006-0064). The experimental protocol was approved by the University Ethics Committee for the use of experimental animals and conformed to the Guide for Care and Use of Laboratory Animals.
For the nasal absorption studies, Sprague-Dawley male rats weighing 180–220 g were used and allowed free access to food and water. Before experimentation, the rats were anaesthetized with urethane at a dose of 0.1 mgml−1 and were placed in a supine position on a working surface. Then, the rats were surgically treated by cutting trachea and the trachea was cannulated with a polyethylene tube to aid breathing (Mei et al., Citation2008). Another tube was inserted through the esophagus into the posterior part of the nasal cavity. The nasopalatine duct was closed with an adhesive agent to prevent drainage of the solution from nasal cavity into mouth. The tube inserted into the esophagus was connected to a reservoir containing 5 ml of drug solution under magnetic stirring and immersed in a water bath at 37 °C. The solution was circulated, by means of a peristaltic pump from the reservoir through the nasal cavity and out of the nostrils back into the reservoir. Flow rate was set at 2.0 mlmin−1. Aliquots (100 μl) were sampled at various time intervals up to 2 h and replenished with an equal volume of saline. SMS contents were analyzed by HPLC assay as described in the section “in vitro analytical method of SMS”.
Nasal ciliotoxicity of different absorption enhancers
Nasal ciliotoxicity studies were carried out using an in situ toad palate model (Jiang et al., Citation1995). In brief, the upper palate of the toads (30–40 g, male and female) was exposed and treated with 0.5 ml of 0.5% laurate SE, 0.1% cremophor EL and 0.5% poloxamer 188 saline solutions for 0.5 h, respectively. Thereafter, the test formulation was removed by washing the palate with saline, and then approximately 5 mm × 3 mm of the palate was dissected and the mucocilia was examined with a Motic DMBA450 light microscopy (Motic China Group Co. Ltd., Beijing, China) and Camera (Nikon Fx-35A, Tokyo, Japan) at enlargement of 400× at regular intervals. The time from test solution administration to the stop of cilia movement was recorded and noted as the lasting time of cilia movement. Saline, ephedrine hydrochloride (1%, w/v solution) and sodium deoxycholate (one of the agents with serious nasal ciliotoxicity, 1%, w/v solution) were used as the blank, negative and positive controls, respectively. The relative inhibition rate of different absorption enhancers was calculated according to the following equation:
In vivo studies in rats
Twenty-five healthy male SD rats weighing 200 ± 20 g were divided into five groups, 0.1% cremophor EL group, 0.5% poloxamer 188 group, 0.5% laurate SE group, the saline control group and intravenous administration group. The nasal formulations were administered using a microsyringe (Hamilton Bonaduz AG, Switzerland) attached via a needle to a short polyethylene tube inserted approximately 0.7 cm into one nostril (15 mgkg−1) (Mao et al., Citation2006). For intravenous administration, a bolus injection was administered via the tail vein. For all the groups tested, at 3, 5, 10, 15, 20, 30, 45, 60 and 120 min after administration, blood samples of 0.3 ml were withdrawn and were harvested immediately by centrifugation at 4000 rpm for 15 min. Then, plasma samples were collected and stored at −20 °C until analysis. Plasma samples were processed with the following steps: 200 μl of methanol and 10 μl of internal standard (50 μg/ml carbamazepine in methanol) were added into 100 μl of serum samples. The mixture was vortexed for 3 min and centrifuged at 4000 rpm for 10 min. The supernatant was used for HPLC assay. Each point represents the mean of five rats.
Statistical and pharmacokinetic data analysis
The peak plasma concentration (Cmax) and the time to reach the peak concentration (Tmax) were obtained directly from the plasma concentration–time curves. The absolute bioavailability (F) was calculated according to the following equation:
The pharmacokinetic parameters were calculated using DAS 2.1.1 program (Mathematical Pharmacology Professional Committee, Shanghai, China). Data are presented as means ± standard deviations of five experiments. Probability values p < 0.05 were considered significant.
Results
Since preliminary experiments demonstrated that SMS was very stable in the nasal perfusate for over 2 h, therefore any loss of the drug from the perfusate was regarded as its absorption across the nasal mucosa.
Effect of pH on the intranasal absorption of SMS
Keeping drug concentration at 100 μg ml−1, intranasal absorption of SMS at pH 5.50, 6.00 and 6.50 was investigated, respectively. As shown in , drug absorption was very fast in the initial 40 min and almost leveled off thereafter. No remarkable difference in absorption was found in the pH range of 5.50–6.50 (p > 0.05). Since pH of normal human nasal mucosa is in the range of 5.5–6.5 (Mei et al., Citation2008), and the pH of commercially available preparation is 5.50, therefore, pH 5.50 was selected for the following studies.
Effect of drug concentration on the intranasal absorption of SMS
Keeping solution pH at 5.5, the effect of drug concentration on the intranasal absorption of SMS was studied at three concentration levels, 50, 100 and 150 μg ml−1, respectively. As shown in , irrespective of the initial concentration, the amount absorbed increased linearly with time in the initial 20 min, and the absorption rate constants were 1.3141, 2.2975 and 2.4972 μg min−1 for 50, 100 and 150 μg ml−1of drug solutions, respectively. Thereafter, the absorption rate slowed down and almost leveled off after 40 min. These results demonstrated that permeation of SMS across nasal epithelium was dose dependent. 100 μg ml−1 was selected for the following studies to investigate the influence of different absorption enhancers.
Effect of poloxamer 188
So far, poloxamer 188 has not been investigated as the intranasal absorption enhancer of hydrophilic drugs in animal models (Lin et al., Citation2007). In the present study, influence of poloxamer 188 concentration on the intranasal absorption of BCS III drug, SMS, was investigated and the results are shown in . Compared with the control group, all the poloxamer groups investigated increased the absorption of SMS significantly (p < 0.05) and the absorption-enhancing effect was poloxamer 188 concentration dependent. No difference in absorption was found between 0.3% and 0.5% poloxamer 188 group (p > 0.05), and further increasing poloxamer 188 concentration to 1% caused a decrease in absorption, and no difference in absorption was found between 1% and 2% poloxamer 188 group in the initial 40 min (p > 0.05), and further absorption decrease was observed in 2% poloxamer 188 group thereafter. Similar results were found when poloxamer 188 was used as the absorption enhancer of isosorbide dinitrate (Na et al., Citation2010).
Effect of cremophor EL
The effect of cremophor EL concentration on the intranasal absorption of SMS is shown in . Even when cremophor EL concentration was as low as 0.05% (w/v), the absorption-enhancing effect was remarkable as early as 5 min compared with the control group, further increasing cremophor EL concentration to 0.1% caused a considerable increase in absorption after 10 min (p < 0.05). However, increasing cremophor EL concentration further to 0.5% caused a decrease in drug absorption compared with that of cremophor EL 0.1% (w/v) group although it is higher than that of 0.05% (w/v) group. Moreover, it was noted that in the cremophor EL containing groups, drug absorption increased almost linearly with time after 20 min, and the saturation phenomenon as observed in the control group disappeared.
Effect of laurate SE
It has been reported that laurate SE can be used as an absorption enhancer for intestinal absorption of water-soluble high molecular weight compounds (Onishi et al., Citation2012). Therefore, it is potential as an intranasal absorption enhancer was investigated. The effect of laurate SE concentration on the intranasal absorption of SMS was studied in the concentration range of 0.05–0.5% and the results are shown in . It was noted that the intranasal absorption-enhancing effect of laurate SE increased with time and concentration. Compared with the control group, only after 20 min 0.05% (w/v) laurate SE group exhibited significant absorption promoting effect (p < 0.05). Moreover, the absorption saturation time was prolonged to 90 min compared with 40 min in the control group. In contrast, laurate SE 0.1%, 0.5% groups increased the absorption of SMS remarkably (p < 0.05) at all the time points starting as early as 5 min, and no saturation occurred in 2 h. Drug absorption increased with time and laurate SE concentration, with absorption rate constants 3.75, 5.65, 6.66 μg min−1 for 0.05%, 0.1% and 0.5% laurate SE groups, respectively.
Comparison of different absorption enhancers and safety assessment
Based on the above results, by selecting the concentration with the best absorption-enhancing effect, intranasal permeation capacity of the three non-ionic surfactants was compared. As shown in , the absorption-enhancing effect followed the order: 0.5% laurate SE >0.1% cremophor EL >0.5% poloxamer 188. Moreover, 0.5% laurate SE group exhibited the fastest onset of action although no statistical difference in absorption was found among the three groups in the initial 30 min (p > 0.05). Thereafter, drug permeation amount in 0.1% cremophor EL group increased linearly at a rate of 1.0899 μg min−1. 0.5% laurate SE group had the best absorption-enhancing effect in the first 10 min than that of 0.1% cremophor EL group and 0.5% poloxamer 188 group (p < 0.05), and the permeation amount increased linearly with time after 30 min at a rate of 1.2657 μg min−1. In contrast, a plateau occurred at 90 min in 0.5% poloxamer 188 group.
In addition to the absorption-enhancing effect, safety of the absorption enhancers is of special importance for clinical application. Therefore, ciliotoxicity of different enhancers at the selected concentration was investigated using an in situ toad palate model and the results are shown in . The time of cilia movement for the saline control group was 705 min. The relative inhibition rates of laurate SE (0.5%, w/v), cremophor EL (0.1%, w/v) and poloxamer 188 (0.5%, w/v) were 75.6%, 80.2% and 86.6%, respectively, all higher than 70%, indicating that the non-ionic type absorption enhancers selected in this study have good safety profiles to the cilia in the nasal cavity.
In vivo absorption studies
Based on the in situ absorption data and ciliotoxicity results, the following three samples, 0.5% laurate SE, 0.1% cremophor EL and 0.5% poloxamer 188 were selected for in vivo absorption studies in rats. Meanwhile, intranasal absorption of SMS without any enhancers was studied as control and the absorption of SMS after intravenous injection was also studied. As shown in , drug concentration was measurable 2 min after intranasal administration of all the solutions. SMS was absorbed into the blood quickly with Tmax of approximately 5 min for all the three tested groups, compared with 10 min for the control group, indicating that SMS was rapidly absorbed via intranasal route compared with that of 60 min per oral in rabbits (Sheshala et al., Citation2011). The pharmacokinetic profiles can be described with a two-compartment model. The main pharmacokinetic parameters are listed in . Compared with the control group, all the absorption enhancers investigated increased the Cmax and AUC of SMS significantly (p < 0.05). Among them, 0.5% laurate SE group presented the highest enhancing effect, followed by 0.1% cremophor EL and 0.5% poloxamer 188 group, with absolute bioavailability 29.99%, 22.64% and 20.90%, respectively. This is in good agreement with the in situ absorption data.
Figure 8. Mean plasma concentration–time profiles after intranasal application of SMS containing 0.5% laurate SE, 0.5% poloxamer 188 and 0.1% cremophor EL, respectively, in rats, compared with the intranasal control group (15 mgkg−1) and intravenous injection group (3 mgkg−1). Indicated values are the mean of five experiments (n = 5).
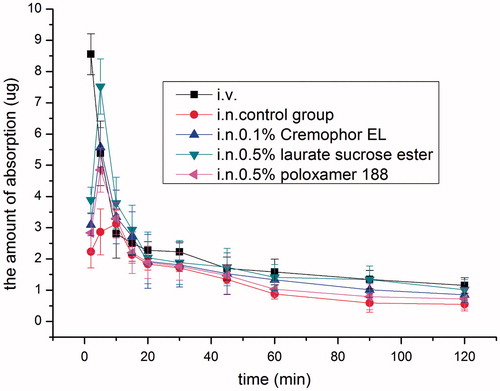
Table 1. Main pharmacokinatic parameters after intranasal (i.n.) administration of SMS (15 mg kg−1) with 0.5% laurate SE, 0.5% poloxamer 188 and 0.1% cremophor EL, the control group and intravenous injection group (i.v.) (mean ± SD, n = 5).
Discussion
The absorption of SMS via intranasal route was found to be pH independent. The pKa of SMS is 9.5, when the system pH changed from 5.5 to 6.5, SMS exists in the same form; therefore, pH has no significant effect on the intranasal absorption of SMS in this range.
The intranasal absorption of SMS was concentration dependent and saturable. Irrespective of the initial concentration, the amount absorbed increased linearly with time in the initial 20 min, and then the absorption rate slowed down and almost leveled off after 40 min. Similarly, a saturation phenomenon in in situ test has been reported with another hydrophilic drug analgin (Mao et al., Citation1997). It has been hypothesized that the main transport mechanism of hydrophilic drug across nasal mucosa is by aqueous pores (Chavanpatil & Vavia, Citation2005). This saturation phenomenon can probably be explained by the fact that the number of aqueous pores in the nasal epithelium is limited and can be saturated. Thus, after a period of time intranasal absorption of SMS was impeded.
From absorption-enhancing point of view, both ionic and non-ionic surfactants can be used as intranasal absorption enhancers. However, taking ciliototoxicity into account, non-ionic surfactants are more popular. Although poloxamer 188 has been regarded as one of the safest excipients in the field of pharmacy and can be used as emulsifier in the preparation of submicron emulsion for intravenous injection, only limited information is available regarding its potential as an intranasal absorption enhancer. Our previous study demonstrated that poloxamer 188 enhanced the intranasal absorption of a hydrophobic drug isosorbide dinitrate (Na et al., Citation2010). However, its capacity to enhance the intranasal absorption of hydrophilic drugs is still in doubt since it was reported that poloxamer 188 was ineffective as a permeating enhancer of hydrophilic compounds in nasal epithelial cells (Lin et al., Citation2007). Recent oral bioavailability study of ketoprofen also indicated that poloxamer 188 presented no apparent intestinal absorption-enhancing effect (Fischer et al., Citation2012). Here, our present study demonstrated for the first time, using both in situ and in vivo data, that poloxamer 188 could enhance the intranasal absorption of BCS class III drug, implying that poloxamer 188 has good absorption promoting effect for both hydrophilic and hydrophobic drugs. Probably, poloxamer may insert into lipid membranes, changing the lateral packing density of membranes therefore enhanced drug absorption (Seelig & Gerebtzoff, Citation2006). Moreover, it was disclosed that the absorption-enhancing effect of poloxamer 188 was concentration dependent and 0.3–0.5% poloxamer 188 was much more effective than that of 1% poloxamer 188. The same phenomenon was observed when poloxamer 188 was used as the absorption enhancer of isosorbide dinitrate (Na et al., Citation2010).
It has been demonstrated previously that cremophor EL (2.5 ml/kg) given simultaneously with Dox (20 mg/kg, i.p.) increased Dox levels in plasma, heart, liver and kidneys of healthy mice as a result of enhancing its bioavailability (Badary et al., Citation1998). Its potential as intranasal absorption enhancer has not been reported so far and was investigated in the present study. It was noted that initially, the permeation effect of cremophor EL increased with concentration, the best absorption-enhancing effect was observed at concentration 0.1% and further increasing cremophor EL concentration to 0.5% caused a decrease in drug absorption. The absorption-enhancing effect of cremophor EL can probably be explained by its capacity to open the tight junctions between cells (Batrakova et al., Citation1998). Levente Kürti found that both 1 and 10 mg/ml cremophor EL could lead to a significant and transient transepithelial electrical resistance (TEER) decrease (Kurti et al., Citation2012). Moreover, Bhagwant D. Rege proved that cremophor EL was able to influence the fluidity of cell membrane (Rege et al., Citation2002). Perhaps, the increased cellular and paracellular transport explained the significant drug absorption in cremophor EL containing groups, with disappearance of the saturation phenomenon as observed in the control group. Based on the best of knowledge, this is the first time that cremophor EL was reported as an intranasal absorption enhancer. Although no other safety data about cremophor EL were reported, Levente Kürti confirmed cremophor RH40 is non-toxic and could be used at 5 mg/ml concentration for 1 h in epithelial cells without cellular damage (Kurti et al., Citation2012), and both of them are already included in USP/NF and Ph. Eur. In our study, the concentration of cremophor EL used was 0.1% (w/v), and it was regarded as safe based on cytotoxicity data.
SEs have been reported as absorption enhancers in the field of oral, transdermal, even nasal and ocular (Pillion et al., Citation2002; Szuts & Szabo-Revesz, Citation2012). Here, laurate SE was selected in this study since among different SEs, laurate SE showed the most obvious effect on the oral absorption of lidocaine hydrochloride (Ganem-Quintanar et al., Citation1998). Thus, the potential of laurate SE as an intranasal absorption enhancer was investigated. The intranasal absorption-enhancing effect of laurate SE was found to be time and concentration dependent, and the absorbed amount increased with time and laurate SE concentration. Similar phenomenon was reported by Hiraku Onishi, showing that when the concentration of laurate SE increased from 2.5% to 29%, the AUC of FD-4 increased from 234 ± 100 to 2294 ± 807 μg·min/ml (Onishi et al., Citation2012). Notably, laurate SE 0.5% group increased the absorption of SMS significantly at all the time points investigated and no saturation occurred until 2 h. As to its absorption-enhancing mechanism, laurate sucrose might increase the intranasal absorption of sumatriptan via a transcellular and a paracellular pathway. It has been demonstrated that sugar esters increased membrane fluidity of lipid layers of the intestinal brush border membranes and decreased the TEER of Caco-2 cells (Yamamoto et al., Citation2014).
Compared with poloxamer 188 and cremophor EL, laurate SE was the most effective absorption enhancer both in situ and in vivo, implying its potential as promising intranasal absorption enhancer. Moreover, the safety of laurate SE has not only been demonstrated by the ciliototoxicity test in this study but also by many previous studies. In fact, SEs based on palmitic/stearic acid or lauric acid have been regarded as safe and registered in the Japanese Standards of Cosmetic Ingredients (Szuts & Szabo-Revesz, Citation2012). Recently, Drummond studied the toxicity of SEs in animal models and in humans (Drummond et al., Citation2003). Acute toxicity studies in animals demonstrated that no significant toxic events were associated with the oral ingestion of SEs, except when SEs were administered at a high dose (10 g/kg) to rats. By using a non-loop method, Hiraku Onishi studied the safety when laurate SE (10%, w/v) was used as an intestinal absorption enhancer, no intestinal damage was observed with the addition of laurate SE at 2.5–20% (w/v) (Onishi et al., Citation2012). Moreover, Yamamoto et al. evaluated the intestinal membrane damage with or without various sugar esters. The sugar esters (0.5%, w/v) did not increase the activities of lactate dehydrogenase, suggesting that these sugar esters did not cause serious membrane damage to the intestinal epithelium (Yamamoto et al., Citation2014).
Conclusions
In this work, taking SMS as a model drug, the potential of three non-ionic surfactants, laurate SE, cremophor EL and poloxamer 188, as intranasal absorption enhancers was studied both in situ and in vivo in rats. All the surfactants tested are quite effective in enhancing the intranasal absorption of SMS. Among them, laurate SE exhibited the most efficient absorption-enhancing effect. In conclusion, this study demonstrated that non-ionic surfactants can be applied as novel intranasal absorption enhancers for drugs with poor permeability.
Declaration of interest
The authors report no conflicts of interest.
References
- Ahsan F, Arnold JJ, Meezan E, et al. (2003). Sucrose cocoate, a component of cosmetic preparations, enhances nasal and ocular peptide absorption. Int J Pharm 251:195–203
- Badary OA, Al-Shabanah OA, Al-Gharably NM, et al. (1998). Effect of cremophor EL on the pharmacokinetics, antitumor activity and toxicity of doxorubicin in mice. Anticancer Drugs 9:809–15
- Badgujar SD, Sontakke M, Narute D, et al. (2010). Formulation and evaluation of sumatriptan succinate nasal in-situ gel using fulvic acid as novel permeation enhancer. Int J Pharm Res Dev 2:1–8
- Batrakova EV, Han HY, Alakhov V, et al. (1998). Effects of pluronic block copolymers on drug absorption in Caco-2 cell monolayers. Pharm Res 15:850–5
- Chavanpatil MD, Vavia PR. (2005). Nasal drug delivery of sumatriptan succinate. Pharmazie 60:347–9
- Csaba N, Sánchez A, Alonso MJ. (2006). PLGA:poloxamer and PLGA:poloxamine blend nanostructures as carriers for nasal gene delivery. J Control Release 113:164–72
- Ding J, Li J, Mao S. (in press). Development and evaluation of vinpocetine inclusion complex for brain targeting. Asian J Pharm Sci http://dx.doi.org/10.1016/j.ajps.2014.08.008
- Ding J, Na L, Mao S. (2012). Chitosan and its derivatives as the carrier for intranasal drug delivery. Asian J Pharm Sci 7:349–61
- Duan X, Mao S. (2010). New strategies to improve the intranasal absorption of insulin. Drug Discov Today 15:416–27
- Drummond CJ, Fong C, Krodkiewska I, et al. (2003). Sugar fatty acid esters. In: Novel surfactants, surfactant science series, Holmberg K (eds). CRC Press, Taylor & Francis, Inc: London, UK, Vol. 114, 95–128
- Femenia-Font A, Balaguer-Fernandez C, Merino V, et al. (2005). Effect of chemical enhancers on the in vitro percutaneous absorption of sumatriptan succinate. Eur J Pharm Biopharm 61:50–5
- Fischer SM, Parmentier J, Buckley ST, et al. (2012). Oral bioavailability of ketoprofen in suspension and solution formulations in rats: the influence of poloxamer 188. J Pharm Pharmacol 64:1631–7
- Ganem-Quintanar A, Quintanar-Guerrero D, Falson-Rieg F, et al. (1998). Ex vivo oral mucosal permeation of lidocaine hydrochloride with sucrose fatty acid esters as absorption enhancers. Int J Pharm 173:203–10
- Illum L. (2012). Nasal drug delivery-recent developments and future prospects. J Control Release 161:254–63
- Jiang XG, Cui JB, Fang XL, et al. (1995). Toxicity of drugs on nasal mucocilia and the method of its evaluation. Acta Pharm Sin 30:848–53
- Jose S, Ansa CR, Cinu TA, et al. (2013). Thermo-sensitive gels containing lorazepam microspheres for intranasal brain targeting. Int J Pharm 441:516–26
- Kurti L, Veszelka S, Bocsik A, et al. (2012). The effect of sucrose esters on a culture model of the nasal barrier. Toxicol In Vitro 26:445–54
- Lin H, Gebhardt M, Bian S, et al. (2007). Enhancing effect of surfactants on fexofenadine HCl transport across the human nasal epithelial cell monolayer. Int J Pharm 330:23–31
- Mao S, Shi Z, Bi D. (1997). Analgin nasal absorption. Chinese Pharm J 32:87–91
- Mao S, Yang S, Bi D. (2006). Pharmacodynamics and potential toxicity of intranasally administrated dipyrone. Biol Pharm Bull 29:1355–9
- Mei D, Mao S, Sun W, et al. (2008). Effect of chitosan structure properties and molecular weight on the intranasal absorption of tetramethylpyrazine phosphate in rats. Eur J Pharm Biopharm 70:874–81
- Na L, Mao S, Wang J, et al. (2010). Comparison of different absorption enhancers on the intranasal absorption of isosorbide dinitrate in rats. Int J Pharm 397:59–66
- Onishi H, Imura Y, Uchida M, et al. (2012). Enhancement potential of sucrose laurate (L-1695) on intestinal absorption of water-soluble high molecular weight compounds. Curr Drug Deliv 9:487–94
- Ozbılgın ND, Saka OM, Bozkır A. (2014). Preparation and in vitro/in vivo evaluation of mucosal adjuvant in situ forming gels with diphtheria toxoid. Drug Deliv 21:140–7
- Patel SR, Zhong H, Sharma A, et al. (2007). In vitro and in vivo evaluation of the transdermal iontophoretic delivery of sumatriptan succinate. Eur J Pharm Biopharm 66:296–301
- Pierce MW. (2010). Transdermal delivery of sumatriptan for the treatment of acute migraine. Neurotherapeutics 7:159–63
- Pillion DJ, Ahsan F, Arnold JJ, et al. (2002). Synthetic long-chain alkyl maltosides and alkyl sucrose esters as enhancers of nasal insulin absorption. J Pharm Sci 91:1456–62
- Ravi PR, Aditya N, Patil S, et al. (2013). Nasal in-situ gels for delivery of rasagiline mesylate: improvement in bioavailability and brain localization. Drug Deliv Nov 29. [Epub ahead of print]
- Rege BD, Kao JPY, Polli JE. (2002). Effects of nonionic surfactants on membrane transporters in Caco-2 cell monolayers. Eur J Pharm Sci 16:237–46
- Salama HA, Mahmoud AA, Kamel AO, et al. (2012). Phospholipid based colloidal poloxamer-nanocubic vesicles for brain targeting via the nasal route. Colloids Surf B Biointerfaces 100:146–54
- Seelig A, Gerebtzoff G. (2006). Enhancement of drug absorption by noncharged detergents through membrane and P-glycoprotein binding. Expert Opin Drug Metab Toxicol 2:733–52
- Sheshala R, Khan N, Darwis Y. (2011). Formulation and optimization of orally disintegrating tablets of sumatriptan succinate. Chem Pharm Bull 59:920–8
- Strickley RG. (2004). Solubilizing excipients in oral and injectable formulations. Pharm Res 21:201–30
- Szuts A, Szabo-Revesz P. (2012). Sucrose esters as natural surfactants in drug delivery systems – a mini-review. Int J Pharm 433:1–9
- Udata C, Patel J, Pal D, et al. (2003). Enhanced transport of a novel anti-HIV agent – cosalane and its congeners across human intestinal epithelial (Caco-2) cell monolayers. Int J Pharm 250:157–68
- Yamamoto A, Katsumi H, Kusamori K, et al. (2014). Improvement of intestinal absorption of poorly absorbable drugs by various sugar esters. Yakugaku Zasshi 134:47–53
- Yu C, Gu P, Zhang W, et al. (2011). Evaluation of submicron emulsion as vehicles for rapid-onset intranasal delivery and improvement in brain targeting of zolmitriptan. Drug Deliv 18:578–85