Abstract
Context: Existing medication systems for the treatment of diabetes mellitus (DM) are inconvenient and troublesome for effective and safe delivery of drugs to the specific site. Therefore, investigations are desired to deliver antidiabetics using novel delivery approaches followed by their commercialization.
Objective: The present review aims to provide a compilation on the latest development in the field of novel drug delivery systems (NDDSs) for antidiabetics with special emphasis on particulate, vesicular and miscellaneous systems.
Methods: Review of literature (restricted to English language only) was done using electronic databases like Pubmed® and Scirus, i.e. published during 2005–2013. The CIMS/MIMS India Medical Drug Information eBook was used regarding available marketed formulation of antidiabetic drugs. Keywords used were “nanoparticle”, “microparticle”, “liposomes”, “niosomes”, “transdermal systems”, “insulin”, “antidiabetic drugs” and “novel drug delivery systems”. Single inclusion was made for one article. If in vivo study was not done then article was seldom included in the manuscript.
Results: The curiosity to develop NDDSs of antidiabetic drugs with special attention to the nanoparticulate system followed by microparticulate and lipid-based system is found to emerge gradually to overcome the problems associated with the conventional dosage forms and to win the confidence of end users towards the higher acceptability.
Conclusion: In the current scientific panorama when the area of novel drug delivery system has been recognized for its palpable benefits, unique potential of providing physical stability, sustained and site-specific drug delivery for a scheduled period of time can open new vistas for precise, safe and quality treatment of DM.
Introduction
Diabetes mellitus (DM) has been emerged as an epidemic worldwide in the last few decades and proclaimed as fifth foremost cause of death in most developed and developing countries. According to the latest atlas of International Diabetes Federation (IDF), global dominance of diabetes has touched the figure of 382 million people (5.1 million deaths and 21 million live births were adversely affected by diabetes during pregnancy) which is expected to be 592 million in 2035 (Misra et al., Citation2011; International Diabetes Federation, Citation2013).
Diabetes mellitus is a chronic hyperglycemic condition which is described as a metabolic disorder of multiple etiologies, characterized by improper carbohydrate, fat and protein metabolism. There are two broad categories of DM i.e. type 1 and type 2 (T1DM and T2DM). T1DM is primarily caused by absolute deficiency of insulin however, variable degree of insulin resistance, impaired insulin secretion and increased glucose production are the causes of T2DM. T1DM is further classified into type 1A, i.e. autoimmune destruction of β cells and type 1B, i.e. idiopathic insulin deficiency. Apart from two major types of DM, other types are also acknowledged which are represented in . All types of DM were supposed to be treatable since 1921 when insulin became available. However, better control of T2DM was seen after administering oral hypoglycemics. Approaches, such as pancreas transplantation and gastric bypass surgery have also been attempted with some degree of success. Gestational DM is suggested to manage during and after pregnancy by modifying life style, such as strict control over diet, regular exercise, routine checkup by analyzing blood sugar levels and special care is mandatory after the delivery of baby as mother could be at a higher risk of developing T2DM (Management of Diabetes, Citation2012).
Though several medications are flooding into the market, complete and successful cure of DM still remain untouched because they offer several adverse effects, like gastric irritation, injection phobia, transient nausea and many more. Therefore, these medications eventually result into the patient incompliance and require highly qualified medical expertise (Tuomilehto et al., Citation2001; Ensign et al., Citation2012). Discovering stable/non-invasive drug delivery along with controlled release could prove to be more beneficial. Although precise and safe drug delivery to the specific site for scheduled period of time to get controlled and sustained release remains a touchstone, pharmaceutical researchers have largely endeavored to modify the physical and biological barriers that limit access of drugs to the therapeutic targets.
Novel drug delivery systems (NDDSs) are becoming trendy in recent years because they offer palpable benefits in terms of reduced dosing frequency, increased bioavailability, prevention from degradation specifically against the harsh gastric environment, site specificity and reduced side effects. In vitro, ex vivo and in vivo findings of numerous experimentations worldwide strongly suggested NDDSs as an emerging and promising option to combat major disorders/diseases (Dash & Konkimalla, Citation2011). Literature revealed that the field of drug delivery has marched at a phenomenal pace and a variety of carrier systems have come to the forefront during the last decade. Therefore, the present article portrays up-to-date advancements on delivery of antidiabetics via novel delivery systems and illustrates the worldly trend of research carried out since 2005 onwards.
Problems posed by the antidiabetics
Antidiabetics are effortlessly accessible in the international market (). Most of the oral hypoglycemics are available either in the form of tablets and capsules. However, these dosage forms offer various untoward effects/limitations like gastric irritation, diarrhea, loss of appetite, lactic acidosis in people with abnormal kidney or liver function due to gastrointestinal degradation, insolubility in water and do not comply with the safety and efficacy of the patients (http://www.joslin.org/info/oral_diabetes_medications_summary_chart.html). These adverse effects revealed the limited accessibility of conventional dosage forms at desired site of action, higher systemic toxicity, narrow therapeutic window, complex dosing schedule for long-term treatment (Sutradhar & Sumi, Citation2014). Two major factors required for the drugs to be effective are their optimal concentration at the desired site of action and persistent effective concentration for a longer period of time. After immediate release, oral hypoglycemics get absorb from the biological membranes and move toward the site of action. However, to attain the therapeutic concentration into the blood for whole day, about 2 to 3 doses are required to be administered (Pradhan, Citation2011; CIMS/MIMS India Medical Drug Information eBook, Citation2013). Moreover, insulin and peptide drugs namely GLP-1, GLP1RAs are available primarily as subcutaneous (SC) injections while peptide analogues, i.e. DPP-4 inhibitors (vildagliptin, (Galvus), sitagliptin (Januvia), saxagliptin (Onglyza)) are available in the global market as tablets. They have been approved by EU and USFDA for the treatment of DM in last decade. Liraglutide, Exenatide and Albiglutide act through GLP-1 receptors, which are found in brain, lung, pancreatic islets, stomach, heart, intestine and kidney. Due to the susceptibility to the proteolytic degradation in gastric environment and shorter plasma half-life, they are less frequently used for oral administration (Kalra, 2011). Their injections are available in the market but injections of exenatide cause nausea, vomiting and antibody formation when used twice a day, while liraglutide injections cause mild, transient nausea and vomiting when used once a day (Kalra, Citation2013). Pain at injection site and hence injection phobia is another limitation. Enhancing oral bioavailability of these analogues is a challenge. Therefore, thorough investigation is required in the area of drug delivery which could be possible by examining new and more specific drug delivery carriers (Schmid & Korting, Citation1996).
Table 1. Some antidiabetic drugs in conventional formulations.
Probable solutions offered by the novel drug delivery systems
Limitations associated with antidiabetics offered the opportunities for the investigators to come up with such delivery systems having improved therapeutic efficacy. Novel drug delivery carrier systems are developed to deliver antidiabetic drugs safely and more precisely to the specific site for scheduled period of time in a controlled manner for better therapeutic effectiveness and henceforth better control over DM. Additionally, alteration in physicochemical and pharmaceutical properties of antidiabetics has avoided/modified the biological barriers that limit availability of actives to the various therapeutic targets. Significant advantages and major limitations of these systems are summarized in . While an array of investigations have been carried out, strategies to come up with the appropriate carrier systems for antidiabetic delivery are still being made to get widespread recognition in the global market. One such investigation was attempted by one of the authors (Agrawal et al., Citation2014). The proteolytic stability and antidiabetic potential of insulin were improved by developing folic acid functionalized, polymer stabilized multilayered liposomal carrier for oral administration. Liposomes were reasonably stabilized using negatively charged poly(acrylic acid) and positively charged poly(allyl amine) hydrochloride (i.e. layer by layer coating of polyelectrolytes) as coating materials and folic acid appended as ligand. Folic acid has specificity to the folic acid receptors which are usually present in ample amount over the GI tract. The excellent stability of this newly designed liposomal carrier in simulated biological fluid and hypoglycemic potential in diabetic animals for at least 18 h indicated improved therapeutic profile. In future, this investigation may be converted into a clinical reality after scaling up of this technology at large scale. In the current scientific panorama, when the area of NDDS has been recognized for its palpable benefits, the above kind of investigations can open new vistas for precise, safe and quality treatment of DM.
Table 2. Novel drug delivery systems for antidiabetics – advantages and limitations.
Designing and development of novel drug delivery systems
Researchers in the area of drug delivery are constantly working on designing and development to improve the performance of intelligent drug delivery system with the purpose to maximize therapeutic activity and to minimize undesirable side-effects. It could be achieved by altering the formulation variables like process or concentration (independent variables), which eventually escalate end-user confidence (Pund et al., Citation2014). Since each delivery system has its own unique pharmaceutical and physiological characteristics; therefore, appropriate consideration of independent variables is very much required in the designing of final product. Acquaintance with physical, chemical and pharmaceutical characteristics of each excipient is also compulsory (Allen, Citation2008). Factors which are attempted to improve the insulin delivery are oral administration, proteolytic and physical stabilities in harsh biological environment. In this regard, effect of various independent variables, such as polymer/cross-linker concentration, sonication time, stirring speed, cross-linking time has been investigated over the obligatory responses (Cui et al., Citation2006; Graf et al., Citation2009). Furthermore, native structure of proteins/peptides, like Glucagon-like peptide 1 (GLP-1), GLP-1 receptors agonist (GLP1RAs) get modified when administered orally, therefore modification of its surface becomes the obligatory step in delivering these drugs before administering (Qi et al., Citation2013). Lipophilicity and poor water solubility of some oral hypoglycemics, like glibenclimide offered other challenges to the formulation scientist, viz. enhancement of dissolution rate which was attempted to improve solubilization of these drugs in simulated biological fluids by altering various process variables (Nnamani et al., Citation2010). Similarly, controlled release, consistent drug plasma concentration, improved permeation are also attempted for antidiabetics with shorter biological half-life, variable drug plasma concentration and higher first-pass metabolism. depicts some of the major novel delivery systems particularly employed for DM.
Mechanism of transport
Depending on various size, shape and integrity, each carrier system has its own mechanism of transport through the biological membranes. Moreover, altering the route of administration may offer other transport mechanism to the carrier systems. A diagrammatic representation of mechanism of drug (fabricated/encapsulated in various novel delivery systems) transport through different biological membranes is shown in , revealing mechanism specificity of individual delivery system from various routes of administration, i.e. topical, transdermal, oral, etc. The major transport pathways of particulate system when administered orally are transcellular and paracellular (Cremaschi et al., Citation1996; Brooking et al., Citation2001; Illum, Citation2007). Nanosized particles, i.e. up to 10 nm often pass through the paracellular transport in a restricted quantity because the diameter of the tight junctions is 3.9–8.4 A° while larger particles, i.e. 100–500 nm, follow one of the transcellular transports, such as endocytosis and carrier- or receptor-mediated. Drug available around absorption site (previously released from NDDS) is transported via carrier-, receptor- and/or absorption-mediated transcytosis. Lipoidal carriers get integrated with the cell wall and release the drug inside the cell. Transport of particulate delivery system through skin may follow transcellular, intercellular or transappendageal pathway, whereas vesicular delivery system may follow integration of its lipid bilayer with cell wall or transappendageal pathway (Desai et al., Citation2010). Most of the transdermal delivery systems are assisted with physical technique, such as microporation, iontophoresis, electroporation, sonophoresis and microneedles, which have been proved to modify the barrier properties of skin. Therefore, it could be inferred that drug may follow more than one transport mechanism.
Recent advances in antidiabetic drug delivery
Exhaustive literature survey published from January 2005 to December 2013 () indicated keen interest of the researchers to develop NDDSs using antidiabetic drugs which is found to be intensifying gradually. Special attention is being given to nanoparticulate carrier systems followed by microparticulate and lipid-based carriers. Various clinical trials were retrieved from the clinical trials registry of the U.S. National Library of Medicine (www.clinicaltrials.gov; accessed on 4 July 2013) using the key terms “Type 1 Diabetes” and “Type 2 diabetes oral insulin”. Some of them, like inhaled insulin; exubera (Pfizer) gained FDA approval in 2006 but withdrawn from the market early 2007 due to a lack of trade and various safety concerns. Moreover, it was also approved in Europe (EMA) in 2006 but never launched due to multiple concerns and highly restricted indications. Subsequently, in the following months all the companies (Lilly, Novo Nordisk, Sanofi, etc.) have retarded the efforts and currently only Mankind is endeavoring to get the FDA approval with several ambiguous issues (safety, optimal formulation). Furthermore, microporation (a needle-free and pain-free) technique designed to deliver water-soluble drugs, proteins and peptides through skin by creating aqueous micro channels in the stratum corneum is under clinical trial phase II conducted by Altea Therapeutics. Various representative examples of antidiabetic drug-based novel delivery systems are summarized in the following section under the major heading of particulate, vesicular and miscellaneous systems.
Figure 4. Current trend of antidiabetic drug delivery via NDDSs. A graphical representation of number of published articles on anti-diabetic drugs between January 2005–December 2013.
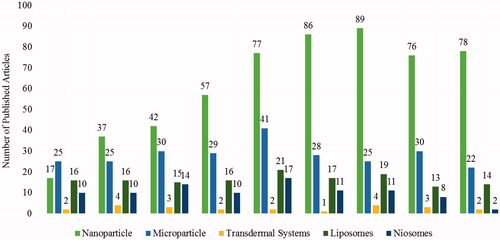
Particulate system
Owing to the revolutionary participation of NDDS in drug delivery, particulate systems are developed periodically by the investigators because of their immense utility. The miniature particles offer even intracellular transport of drugs and attachment of specific ligands make them accessible to the specific receptor/cell/organ. Hence, these systems are considered as most preferred carriers for the delivery of antidiabetic drugs (Liu et al., Citation2008b). These carriers are categorized into two major classes, i.e. microsized and nanosized. A range of nano-scale drug delivery technologies, such as nanovesicles, nanotubes, micelles, dendrimers, nanoshells and many more have been investigated using variety of materials for instance biologics (lipids, peptides, nucleic acids, polysaccharides), polymers (poly(alkylcyanoacrylate), poly(3-hydroxybutanoic acid), poly(organophosphazene)), silicon, carbon and metal (Hughes, Citation2005). These systems are fabricated either via matrixing or by encapsulation which control the release by means of diffusion and erosion of drugs from particulate systems (Dash & Konkimalla, Citation2011).
Microparticulate systems
Microparticle-based therapy allows tailored drug release to the specific treatment site and formulation of various drug–polymer combination. Microparticulate system help in maintaining the therapeutic concentration of drugs (shorter half-life) in plasma for longer period of time by controlling their release. Being small in size, microparticles have larger surface-to-volume ratios and can be developed for the improvement of dissolution rate of practically insoluble drugs. Moreover, to attain optimal therapeutic concentration of drug in systemic circulation, variables, such as dose and release kinetic are manipulated time to time as per the requirement by employing microencapsulation methods, varying drug–polymer ratio etc. (Sinha et al., Citation2004). Microparticulate systems have been developed for oral (Rekha & Sharma, Citation2009), topical (Zhao et al., Citation2010), parenteral (Mesiha et al., Citation2005) and nasal (Zhang et al., Citation2008) routes. Literature abounds with the studies that report various type of microparticulate systems, such as magnetic microparticles, solid lipid microparticles, biological microparticles, metal based and polymeric microparticles () (Pereswetoff-Morath, Citation1998; Sinha et al., Citation2004; Bilati et al., Citation2005; Hughes, Citation2005; Dash & Konkimalla, Citation2011). Concept of microencapsulation has been used to modify drug release pattern and to enhance in vivo hypoglycemic effect of Glipizide and Gliclazide (Madhusudhan et al., Citation2010; Barakat et al., Citation2013). To lengthen the life span of insulin via oral route, Furtado et al. (Citation2008) have developed poly(fumaric-co-sebaceous) anhydride microspheres. In double dose experiment it was found that insulin containing microspheres are capable enough to maintain constant plasma glucose level (about 100 mmol/L) during the entire study for at least 15 h. The mechanisms of release of microencapsulated drugs is reported to be erosion or diffusion for matrix type and diffusion for reservoir type (Soppimath et al., Citation2001; Hughes, Citation2005). Literature unfolded that, the major transport mechanism of microparticulate systems is transcellular (through endocytosis and carrier- or receptor-mediated transport). It was suggested by Illum (Citation2007) that hydrophilic/hydrophobic nature and size of particles are the limiting factor in the transport through biological membranes. Microsized particles are barely able to cross the mucosal membrane by the paracellular route because normal diameter of the tight junctions is 3.9–8.4 A°. Hence, microparticles cross biological membrane normally via transcellular pathway, i.e. endocytosis (carrier- or receptor-mediated).
Table 3. Recent reports on various microparticulate systems for the treatment of diabetes (Year 2005 onward).
Nanoparticulate systems
Relatively higher intracellular uptake of nanoparticulate systems makes them the carriers of choice in delivering antidiabetic drugs (). Moreover, they have wide size range (1–1000 nm), covering a variety of structures, such as nanospheres, nanocapsules, nanodiamonds and nanofibers (Hamidi et al., Citation2008; Dash & Konkimalla, Citation2011). They can be categorized as polymeric NPs, metal-based NPs, lipid-based NPs and biological NPs. Methods adopted to develop these nanostructures are emulsion solvent evaporation, solvent displacement, diffusion solvent evaporation, ionotropic polyelectrolyte pre-gelation, polyelectrolyte complexation, dispersion polymerization, interfacial polymerization of microemulsion, ionic gelation, free-radical precipitation/dispersion, etc. (Cui et al., Citation2006; Battaglia et al., Citation2007; Zhang et al., Citation2008; Graf et al., Citation2009; Rekha & Sharma, Citation2009; Woitiski et al., Citation2010; Dash & Konkimalla, Citation2011). They have undoubtedly improved the blood glucose lowering potential of drug-like insulin even up to 22 days (), protected actives from harsh gastric pH, enhanced bioavailability and reduced dosing frequency (Finotelli et al., Citation2010; Peng et al., Citation2012). Liu et al. (Citation2008a) have reflected the potential advantages of nanoparticulate systems, i.e. mainly attributed to their minute size and explicit morphology which makes them able to pass through very fine blood capillaries and protect peptide/peptide surrogate or other contents from deteriorator enzymes. In one of the reports, Soppimath et al. (Citation2001) have published that, the rate of drug release and pattern of NPs degradation depend on desorption of drug molecules adsorbed on surface, matrix diffusion of NP, drug diffusion from polymer shell, matrix erosion of nanoparticles and combination of erosion as well as diffusion. As far as their transport from biological membrane is concerned, authors declared that nanoparticles are transported via both cellular uptake processes, such as transcellular (clathrin- and caveoli-mediated endocytosis, pinocytosis, and less commonly known, phagocytosis) and paracellular pathway (Brooking et al., Citation2001; Illum, Citation2007; Jong & Borm, Citation2008). Furthermore, increased mucoadhesion and retention of nanoparticles in the gastrointestinal tract is due to electrostatic interactions between the positively charged nanoparticles and the negatively charged mucus and endothelial layer, or through a physical capture of the nanoparticle by the mucus layer. These systems have improved oral delivery of poorly adsorbed drugs and proteins by increasing time of retention and extent of interaction with the mucus layer of the intestine. Mucoadhesive polymers, such as Eudragit (Evonik, Essen, Germany), poly(acrylic acid), sodium alginate and chitosan help in mucoadhesiveness, which ensures increased mucosal interaction. Quick exit of nanoparticles can be seen if they are loosely attached to the mucus layer. Therefore, it is preferred to achieve adherence in the deeper mucus layer, which is shed less often and provides a longer interaction with the GI tract. Site specificity and controlled release nature of these systems was also affirmed owing to their biodegradability, pH specificity, ionic and/or temperature sensibility, well-organized drug distribution pattern along with drug protection and lower onset of toxicity (Soppimath et al., Citation2001; Koo et al., Citation2005; Hamidi et al., Citation2008; Jong & Borm, Citation2008; Faraji & Wipf, Citation2009).
Table 4. Recent reports on different nanoparticulate approaches used for antidiabetics (Year 2005 onward).
Recently, nanomedicine-based oral drug delivery systems for antidiabetics have gained significant attention because of their nano size distribution, site specificity and excellent protection to peptides by maintaining their proteolytic stability in physiological fluids.
Vesicular system
Vesicular systems have immense utility in drug delivery due to the presence of lipid bilayer like arrangement similar to the cell membrane. Delivery of bioactive molecules has been improved by means of an ultra-deformable vesicular carrier. The physicochemical characteristics of drugs, like size, charge, lamellarity, elasticity (Benson, Citation2006), solubility and thermodynamics get affected (Vinod et al., Citation2012) by the composition of these carriers. They are reported to deliver drugs, like minoxidil, enoxacin, aceclofenac and estradiol via transdermal route and as a carrier of insulin which improved the proteolytic stability in gastrointestinal tract. These carriers have exhibited safe and sustained delivery of medicaments for longer period of time (Karathanasis et al., Citation2006; Kumar & Rajeshwarrao, Citation2011). Their biodegradable nature and uptake via phagocytes (Gupta et al., Citation2012) provide an opportunity to the formulation scientist to target drugs even inside the cells. Foremost significance of these systems, are to deliver very less soluble drug, improve stability and therapeutic concentration in plasma and reduce dose-dependent toxicity (Huang & Wang, Citation2006; Karathanasis et al., Citation2006). On account of which, a variety of vesicular carriers, such as liposomes, niosomes and pharmacosomes has been attempted periodically () which are offering various opportunities and challenges for formulation scientists to come up with the improved therapy.
Table 5. Vesicular systems developed for antidiabetic drugs.
Liposomes
Liposomes are artificially prepared vesicular systems chiefly composed of a lipid bilayer. Literature unfolds various types of traditional liposomes, such as uni-lamellar (ULV) or multi-lamellar vesicular systems (MLV) (Ye et al., Citation2000; Karathanasis et al., Citation2006). Drugs/actives like cytotoxic, antidiabetics, anti-cancerous, proteinaceous and genetic material have been encapsulated in liposomal systems and it has been proved as a carrier of choice for the drug accumulation at the desired site of action. Several formulation strategies, namely thin film hydration and membrane dialyzing method (Huang & Wang, Citation2006), two-step double emulsification (Pardakhty et al., Citation2007), modified reverse phase evaporation (Pardakhty et al., Citation2007; Misra et al., Citation2009), etc., are made to develop such liposomes using a variety of polymers. Ye and his colleagues (Citation2000) have developed sustained release liposomal carrier system of insulin and peptides, such as leuprolide, enkephalin and octreotide using DepoFoam technology and revealed higher degree of drug loading and very narrow particle (spherical shape) size distribution. In vitro release and in vivo pharmacodynamic studies suggested their sustained release for several weeks. Another approach like triggered release of insulin by means of liposomal delivery to the lungs has also been attempted (Bi et al., Citation2008), which ensured extended hypoglycemic effect, i.e. up to 72 h (Pardakhty et al., Citation2007) with a reduced degree of side effects and improved efficacy. Karathanasis et al. (Citation2006) have developed the inhaler of insulin fabricated in multifunctional liposomal delivery system for its environment-sensitive release after inhaling. Cross-linking of liposomes was made by chemical bridging which was cleavable by cysteine. A rapid release of encapsulated insulin into deep lung took place after the contact of liposomal carrier with cysteine. Today, liposomal technologies have been explored extensively and many of the formulations for antidiabetic drugs are being developed by multinational companies. Some of the formulations have been approved by FDA (Siekmeier & Scheuch, Citation2008). However, introduction of oral formulation in global market is still awaited.
Niosomes
Niosomes, a non-ionic surfactant vesicles having lamellar structure formed by self-assembly of surfactant molecules. Niosomal vesicular systems are developed to improve the oral bioavailability of poorly water soluble drugs, like Glibenclamide, to reduce dosing frequency and dose-dependent toxicity of the drugs with smaller half-life like Metformin. Hydrophilic -lipophilic balance of surfactants, chemical structure of ingredients and packaging parameters are the key factors behind the formation of bilayer vesicles. A variety of properties of non-ionic surfactant vesicular system are summarized (Bi et al., Citation2008; Kumar & Rajeshwarrao, Citation2011). Niosomes have been placed as a system to enhance the bioavailability of drugs having very low aqueous solubility and to make active drug available at the desired site of action in a controlled manner for a longer duration. Insulin-loaded niosomes were also found to have good stability in the presence of proteolytic enzymes of GI tract when sodium deoxycholate was used as a surfactant. Encapsulation of active ingredients in niosomes could prove to be quite appropriate in the reduction of toxicity of antidiabetic drugs, increase absorption, stability and drug action (Pardakhty et al., Citation2007).
Miscellaneous systems
Novel drug delivery systems are becoming the revolutionary approach in delivering antidiabetic drugs. Numerous efforts have been made till date by the formulation scientists. A report on electroporative delivery of insulin, i.e. encapsulated in the polymer vesicles suggested the usefulness of electroporation in the enhancement of insulin deposition over the skin by measuring the flux in vitro (Rastogi et al., Citation2010b). Confocal microscopy demonstrated the presence of nanoparticles in the deeper layers of epidermis. In vivo comparison of electroporation of insulin solution and nanoparticles into the skin showed hypoglycemic effect for at least 24 and 36 h, respectively. Therefore, this approach was considered as an alternative to the injectable insulin formulation. Another method of transdermal delivery is microporation, which mechanically creates micropores into the skin by means of thermal, radiofrequency and laser ablation (Banga, Citation2009). It has been proved as a needle-free and pain-free process to deliver water-soluble drugs, proteins, and peptides through stratum corneum and termed as the PassPort System (Alan & Eric, Citation2008). Since oral hypoglycemics, like glipizide causes severe hypoglycemia and gastric disturbances in the normal doses and intended to be taken for a longer period of time, so the patient compliance is an important concern. To avoid such limitations, Mutalik et al. (Citation2006) have developed Glipizide containing matrix type transdermal systems (TDDSs) using ethyl cellulose (hydrophobic), polyvinylpyrrolidone (hydrophilic) and Eudragit RL-100 (hydrophilic)/Eudragit RS-100 as polymers. These systems can also be used when immediate termination of the therapy is required. Representative miscellaneous systems are summarized in . One of such carriers is porous floating granular delivery system of repaglinide (fast and short acting meglitinide analog), which was reported to demonstrate controlled release and augmented gastric retention time of drug (Jain et al., Citation2007b). Furthermore, a report on nanodiamonds of insulin was published in 2009 (Shimkunas et al., Citation2009) using pH-dependent polymer. In this investigation, bovine insulin was non-covalently bound to detonated nanodiamonds via physical adsorption in an aqueous solution, which demonstrated pH-dependent desorption and significant insulin release in alkaline environments of sodium hydroxide. In another investigation (Chen et al., Citation2011), controlled release, glucose responsive, self-assembled multilayer films of insulin have developed for installation beneath the skin. This multilayer film showed continuous release of insulin in vivo after being subcutaneously implanted in streptozotocin-induced diabetic rats and reduced blood glucose level for at least two weeks. It was affirmed that, this system have potential and great future in glucose-responsive delivery of medicaments due to its distinct mechanism.
Table 6. Miscellaneous noteworthy novel carriers for antidiabetics.
Future prospects
Industries and various leading research institutes are working to develop new ways of administering antidiabetic drugs, from pills to nano and micro structures NDDS-based system. For example, Microcapsule of Octreotide Acetate was prepared by Novartis and clinical trial on human has been completed in 2011 and presently, it is flooding into the market with a brand name SandostatinLAR. This type of practice provides a healthy environment for new NDDSs systems to get into the clinical pipelines. As a consequence, it opens the doors for several other novel systems which are under development. NDDSs of some other drugs, such as Albiglutide nanoparticle (GlaxoSmithKline), Insulin liposome (Phosphagenics, Ltd. Biotek, Andhra Pradesh, India), Transdermal basal insulin patch for the treatment of T1DM (Altea Therapeutics, Clinical Research Center, Atlanta, GA), etc., are in phase II and III of clinical trial and are appeared as a promising option for the delivery of more sensitive drugs. However, progression of NDDS (in case of DM) critically depends on cost, registration, approval procedures. Fortunately, government is highly influenced by the probable outcome from NDDS but little concerned regarding its overall economic impact. Hence, some developed and developing countries decided to provide funds to support the cost of research. Wherever possible, the collaboration amid two or more organizations with multidisciplinary expertise should also be made at global level. Moreover, the protection of intellectual property rights is very important, which offer a huge commercial success of the product after being transferred to the pharmaceutical industry. Furthermore, there is a need to reassess the testing parameters for the evaluation of NDDSs-based products. Toxicological risks, i.e. unique to novel systems, demands amendment in the guidelines in near future. FDA has documented the guideline for “alternative delivery systems” whereas to get the NDDSs-based quality product, specific guidelines are needed in the future. Finally, it could be expected that the success of several over-engineered delivery systems will boost reluctant people to look at NDDSs more closely.
Conclusion
Diabetes mellitus is accounted for extensive health and economic burden annually, however, the development in the field of drug delivery and disease management is stagnant. Simultaneously, the limitations associated with the current marketed formulations demand for easy and non-invasive systems with long lasting hypoglycemic effect and patient compliance. Cognizance of NDDS pertaining to diabetes treatment has risen gradually that is evidenced from nanoparticulate systems in which noteworthy results were found. Moreover, the pharmacoeconomic analysis indicates lower cost of treatment by means of NDDSs. But the commercial, societal and regulatory aspects related with these systems are still ambiguous even when massive research has been done. Therefore, to make these systems available in the global market, their safety profile, safety pharmacology, environmental effects during formulation and their potential effects on the health are to be addressed properly. Furthermore, the developed formulations need to be extensively optimized in different animal species to minimize the incidences of failure at clinical level in different human subjects.
Declaration of interest
The authors declare no conflicts of interest.
References
- Agrawal AK, Harde H, Thanki K, Jain S. (2014). improved stability and antidiabetic potential of insulin containing folic acid functionalized polymer stabilized multilayered liposomes following oral administration. Biomacromolecules 15:350–60
- Ahmed OA, Afouna MI, El-Say KM, et al. (2014). Optimization of self-nanoemulsifying systems for the enhancement of in vivo hypoglycemic efficacy of glimepiride transdermal patches. Expert Opin Drug Deliv 11:1005–13
- Alan S, Eric T. (2008). The PassPort System: a new transdermal patch for water-soluble drugs, proteins, and carbohydrates. Modified-Release Drug Delivery Technology 2:417–26
- Allen LV. (2008). Dosage form design and development. Clin Ther 30:2102–11
- Al-Kassas RS, Al-Gohary OMN, Al-Faadhel MM. (2007). Controlling of systemic absorption of gliclazide through incorporation into alginate beads. Int J Pharm 341:230–7
- Banga AK. (2009). Microporation applications for enhancing drug delivery. Expert Opin Drug Del 6:343–54
- Barakat NS, Almurshedi AS. (2011). Design and development of gliclazide-loaded chitosan microparticles for oral sustained drug delivery: in-vitro/in-vivo evaluation. J Pharm Pharmacol 63:169–78
- Barakat NS, Shazly GA, Almedany AH. (2013). Influence of polymer blends on the characterization of gliclazide–encapsulated into poly(ɛ-caprolactone) microparticles. Drug Dev Ind Pharm 39:352–62
- Battaglia L, Trotta M, Gallarate M, et al. (2007). Solid lipid nanoparticles formed by solvent-in-water emulsion-diffusion technique: development and influence on insulin stability. J Microencapsul 24:660–72
- Bayat A, Dorkoosh FA, Dehpour AR, et al. (2008). Nanoparticles of quaternized chitosan derivatives as a carrier for colon delivery of insulin: ex vivo and in vivo studies. Int J Pharm 356:259–66
- Benson HA. (2006). Transfersomes for transdermal drug delivery. Expert Opin Drug Del 3:727–37
- Bi R, Shao W, Wang Q, Zhang N. (2008). Spray-freeze-dried dry powder inhalation of insulin-loaded liposomes for enhanced pulmonary delivery. J Drug Targ 16:639–48
- Bilati U, Allemann E, Doelker E. (2005). Strategic approaches for overcoming peptide and protein instability within biodegradable nano- and microparticles. Eur J Pharm Biopharm 59:375–88
- Bowey K, Swift BE, Flynn LE, Neufeld RJ. (2013). Characterization of biologically active insulin-loaded alginate microparticles prepared by spray drying. Drug Dev Ind Pharm 39:457–65
- Brooking J, Davis SS, Illum L. (2001). Transport of nanoparticles across the rat nasal mucosa. J Drug Tar 9:267–79
- Cetin M, Atila A, Sahin S, Vural I. (2013). Preparation and characterization of metformin hydrochloride loaded-Eudragit((R))RSPO and Eudragit((R))RSPO/PLGA nanoparticles. Pharm Dev Technol 18:570–6
- Cevc G. (2003). Transdermal drug delivery of insulin with ultradeformable carriers. Clin Pharmacokin 42:461–74
- Chalasani KB, Russell-Jones GJ, Yandrapu Sk, et al. (2007). A novel vitamin B12-nanosphere conjugates carrier system for peroral delivery of insulin. J Control Release 117:421–9
- Chen X, Wu W, Guo Z, et al. (2011). Controlled insulin release from glucose-sensitive self-assembled multilayer films based on 21-arm star polymer. Biomaterials 32:1759–66
- CIMS/MIMS India Medical Drug Information eBook in pdf format. Available from: http://wwwmedicalgeekcom/pharmacology/23913-download-cims-india-medical-drug-information-ebookhtml
- Coelho JF, Ferreira PC, Alves P, et al. (2010). Drug delivery systems: advanced technologies potentially applicable in personalized treatments. EPMA J 1:164–209
- Cremaschi D, Porta C, Ghirardelli R. (1996). The active transport of polypeptides in the rabbit nasal mucosa is supported by a specific vesicular transport inhibited by cytochalasin D. Biochim Biophys Acta 1283:101–5
- Cui F, Shi K, Zhang L, et al. (2006). Biodegradable nanoparticles loaded with insulin-phospholipid complex for oral delivery: preparation, in vitro characterization and in vivo evaluation. J Control Release 114:242–50
- Damge C, Maincent P, Ubrich N. (2007). Oral delivery of insulin associated to polymeric nanoparticles in diabetic rats. J Control Release 117:163–70
- Das S, Roy P, Pal R, et al. (2014). Engineered silybin nanoparticles educe efficient control in experimental diabetes. PLoS One 9:1–13
- Dash TK, Konkimalla VB. (2011). Poly-є-caprolactone based formulations for drug delivery and tissue engineering: a review. J Control Release 58:15–33
- Desai P, Patlolla RR, Singh M. (2010). Interaction of nanoparticles and cell-penetrating peptides with skin for transdermal drug delivery. Mol Mem Bio 27:247–59
- Ensign LM, Cone R, Hanes J. (2012). Oral drug delivery with polymeric nanoparticles: the gastrointestinal mucus barriers. Adv Drug Deliver Rev 64:557–70
- Faraji AH, Wipf P. (2009). Nanoparticles in cellular drug delivery. Bioorgan Med Chem 17:2950–62
- Finotelli PV, Da Silva D, Sola-Penna M, et al. (2010). Microcapsules of alginate/chitosan containing magnetic nanoparticles for controlled release of insulin. Colloids Surf B 81:206–11
- Furtado S, Abramson D, Burrill R, et al. (2008). Oral delivery of insulin loaded poly(fumaric-co-sebacic) anhydride microspheres. Int J Pharm 347:149–55
- Graf A, Rades T, Hook SM. (2009). Oral insulin delivery using nanoparticles based on microemulsions with different structure-types: optimisation and in vivo evaluation. Eur J Pharm Sci 37:53–61
- Gupta M, Agrawal U, Vyas SP. (2012). Nanocarrier-based topical drug delivery for the treatment of skin diseases. Expert Opin Drug Del 9:783–804
- Guo Q, Wu Z, Zhang X, et al. (2014). Phenylboronate-diol crosslinked glycopolymeric nanocarriers for insulin delivery at physiological pH. Soft Matter 10:911–20
- Gwinn MR, Vallyathan V. (2006). Nanoparticles: health effects—pros and cons. Environ Health Perspect 114:1818–25
- Hamidi M, Azadi A, Rafiei P. (2008). Hydrogel nanoparticles in drug delivery. Adv Drug Deliver Rev 60:1638–49
- Hasan AA, Madkor H, Wageh S. (2013). Formulation and evaluation of metformin hydrochloride-loaded niosomes as controlled release drug delivery system. Drug Deliv 20:120–6
- Hinds KD, Campbell KM, Holland KM, et al. (2005). PEGylated insulin in PLGA microparticles. In vivo and in vitro analysis. J Control Release 104:447–60
- Huang X, Du YZ, Yuan H, Hu FQ. (2009). Preparation and pharmacodynamics of low-molecular-weight chitosan nanoparticles containing insulin. Carbohyd Polym 76:368–73
- Huang YY, Wang CH. (2006). Pulmonary delivery of insulin by liposomal carriers. J Control Release 113:9–14
- Hughes GA. (2005). Nanostructure-mediated drug delivery. Nanomed Nanotechnol 1:22–30
- Ilić I, Dreu R, Burjak M, et al. (2009). Microparticle size control and glimepiride microencapsulation using spray congealing technology. Int J Pharm 381:176–83
- Illum L. (2007). Nanoparticulate systems for nasal delivery of drugs: a real improvement over simple systems. J Pharm Sci 96:473–83
- International Diabetes Federation. (2013). IDF diabetes atlas. 6th ed. Brussels, Belgium: International Diabetes Federation
- Jain AK, Chalasani KB, Khar RK, et al. (2007a). Muco-adhesive multivesicular liposomes as an effective carrier for transmucosal insulin delivery. J Drug Targ 15:417–27
- Jain AK, Khar RK, Ahmed FJ, Diwan PV. (2008). Effective insulin delivery using starch nanoparticles as a potential trans-nasal mucoadhesive carrier. Eur J Pharm Biopharm 69:426–35
- Jain S, Saraf S. (2009). Influence of processing variables and in vitro characterization of glipizide loaded biodegradable nanoparticles. Diabetes Metab Syndrome Clin Res Rev 3:113–17
- Jain SK, Agrawal GP, Jain NK. (2007b). Porous carrier based floating granular delivery system of repaglinide. Drug Dev Ind Pharm 33:381–91
- Jain SK, Awasthi AM, Jain NK, Agrawal GP. (2005). Calcium silicate based microspheres of repaglinide for gastroretentive floating drug delivery: preparation and in vitro characterization. J Control Release 107:300–9
- Jong WHD, Borm PGA. (2008). Drug delivery and nanoparticles: application and hazards. Int J Nanomed 3:133–49
- Jose P, Sundar K, Anjali CH, Ravindran A. (2014). Metformin-loaded BSA nanoparticles in cancer therapy: a new perspective for an old antidiabetic drug. Cell Biochem Biophys
- Jose S, Fangueiro JF, Smitha J, et al. (2012). Cross-linked chitosan microspheres for oral delivery of insulin: Taguchi design and in vivo testing. Colloid Surf B 92:175–9
- Kalra S. (2013). Glucagon-like peptide-1 receptors agonists (GLP1 RA). J Pak Med Assoc 63:1312–15
- Karathanasis E, Bhavane R, Annapragada AV. (2006). Triggered release of inhaled insulin from the agglomerated vesicles: pharmacodynamic studies in rats. J Control Release 113:117–27
- Kim JY, Lee H, Oh KS, et al. (2013). Multilayer nanoparticles for sustained delivery of exenatide to treat type 2 diabetes mellitus. Biomaterials 34:8444–9
- Kofuji K, Murata Y, Kawashima S. (2005). Sustained insulin release with biodegradation of chitosan gel beads prepared by copper ions. Int J Pharm 303:95–103
- Koo OM, Rubinstein I, Onyuksel H. (2005). Role of nanotechnology in targeted drug delivery and imaging: a concise review. Nanomed Nanotechnol 1:193–212
- Kumar GP, Rajeshwarrao P. (2011). Nonionic surfactant vesicular systems for effective drug delivery: an overview. Acta Pharma Sin B 1:208–19
- Lekshmi UMD, Poovi G, Kishore N, Reddy PN. (2010). In vitro characterization and in vivo toxicity study of repaglinide loaded poly(methyl methacrylate) nanoparticles. Int J Pharm 396:194–203
- Leong KH, Chung LY, Noordin MI, et al. (2011). Lectin-functionalized carboxymethylated kappa-carrageenan microparticles for oral insulin delivery. Carbohyd Polym 86:555–65
- Liu B, Dong Q, Wang M, et al. (2010). Preparation, characterization, and pharmacodynamics of exenatide-loaded poly(DL-lactic-co-glycolic acid) microspheres. Chem Pharm Bull 58:1474–9
- Liu H, Gong T, Fu HL, et al. (2008a). Solid lipid nanoparticles for pulmonary delivery of insulin. Int J Pharm 356:333–44
- Liu Z, Jiao Y, Wang Y, et al. (2008b). Polysaccharides-based nanoparticles as drug delivery systems. Adv Drug Deliver Rev 60:1650–62
- Ma Z, Lim TM, Lim LY. (2005). Pharmacological activity of peroral chitosan-insulin nanoparticles in diabetic rats. Int J Pharm 293:271–80
- Madhusudhan S, Panda AK, Parimalakrishnan S, et al. (2010). Design, in vitro and in vivo evaluation of glipizide Eudragit microparticles. J Microencapsul 27:281–91
- Maiti S, Ranjit S, Mondol R, et al. (2011). Al+3 ion cross-linked and acetalated gellan hydrogel network beads for prolonged release of glipizide. Carbohyd Polym 85:164–72
- Management of Diabetes. (2012). Federal Bureau of Prisons Clinical Practice Guidelines, 4–18. https://doi.org/http://www.bop.gov/resources/pdfs/diabetes.pdf
- Mesiha MS, Sidhom MB, Fasipe B. (2005). Oral and subcutaneous absorption of insulin poly(isobutylcyanoacrylate) nanoparticles. Int J Pharm 288:289–93
- Misra GP, Singh RS, Aleman TS, et al. (2009). Subconjunctivally implantable hydrogels with degradable and thermoresponsive properties for sustained release of insulin to the retina. Biomaterials 30:6541–7
- Misra P, Upadhyay RP, Misra A, Anand K. (2011). A review of the epidemiology of diabetes in rural India. Diabetes Res Clin Pr 92:303–11
- Mutalik S, Udupa N, Kumar S, et al. (2006). Glipizide matrix transdermal systems for diabetes mellitus: preparation, in vitro and preclinical studies. Life Sci 79:1568–77
- Ning M, Guo Y, Pan H, et al. (2005). Niosomes with Sorbitan Monoester as a carrier for vaginal delivery of insulin: studies in rats. Drug Deliv 12:399–407
- Nishimura A, Hayakawa T, Yamamoto Y, et al. (2012). Controlled release of insulin from self-assembling nanofiber hydrogel, PuraMatrix™: application for the subcutaneous injection in rats. Eur J Pharm Sci 45:1–7
- Nnamani PO, Attama AA, Ibezim EC, Adikwu MU. (2010). SRMS142-based solid lipid microparticles: Application in oral delivery of glibenclamide to diabetic rats. Eur J Pharm Biopharm 76:68–74
- Pal D, Nayak AK. (2012). Novel tamarind seed polysaccharide-alginate mucoadhesive microspheres for oral gliclazide delivery: in vitro-in vivo evaluation. Drug Deliv 19:123–31
- Pardakhty A, Varshosaz J, Rouholamini A. (2007). In vitro study of polyoxyethylene alkyl ether niosomes for delivery of insulin. Int J Pharm 328:130–41
- Peng Q, Zhang ZR, Gong T, et al. (2012). A rapid-acting, long-acting insulin formulation based on a phospholipid complex loaded PHBHHx nanoparticles. Biomaterials 33:1583–8
- Pereswetoff-Morath L. (1998). Microspheres as nasal drug delivery systems. Adv Drug Deliver Rev 29:185–94
- Pradhan SK. (2011). Microsponges as the versatile tool for drug delivery system. Int J Res Pharm Chem 1:243–58
- Pund S, Shete Y, Jagadale S. (2014). Multivariate analysis of physicochemical characteristics of lipid based nanoemulsifying cilostazol by quality by design. Coll and Surf B 115:29–36
- Qi F, Wu J, Fan Q, et al. (2013). Preparation of uniform-sized exenatide-loaded PLGA microspheres as long-effective release system with high encapsulation efficiency and bio-stability. Colloids Surfaces B 112:492–8
- Rastogi R, Anand S, Dinda AK, Koul V. (2010a). Investigation on the synergistic effect of a combination of chemical enhancers and modulated iontophoresis for transdermal delivery of insulin. Drug Dev Ind Pharm 36:993–1004
- Rastogi R, Anand S, Koul V. (2010b). Electroporation of polymeric nanoparticles: an alternative technique for transdermal delivery of insulin. Drug Dev Ind Pharm 36:1303–11
- Reis CP, Ribeiro AJ, Houng S, et al. (2007). Nanoparticulate delivery system for insulin: design, characterization and in vitro/in vivo bioactivity. Euro J Pharm Sci 30:392–7
- Rekha MR, Sharma CP. (2009). Synthesis and evaluation of lauryl succinyl chitosan particles towards oral insulin delivery and absorption. J Control Release 135:144–51
- Ryan GJ, Moniri NH, Smiley DD. (2013). Clinical effects of once-weekly exenatide for the treatment of type 2 diabetes mellitus. Am J Health Syst Pharm 70:1123–31
- Salmaso S, Bersani S, Elvassore N, et al. (2009). Biopharmaceutical characterisation of insulin and recombinant human growth hormone loaded lipid submicron particles produced by supercritical gas micro-atomisation. Int J Pharm 379:51–8
- Schmid MH, Korting HC. (1996). Therapeutic progress with topical liposome drugs for skin disease. Adv Drug Deliver Rev 18:335–42
- Shimkunas RA, Robinson E, Lam R, et al. (2009). Nanodiamond–insulin complexes as pH-dependent protein delivery vehicles. Biomaterials 30:5720–8
- Siekmeier R, Scheuch G. (2008). Inhaled insulin – does it become reality? J Physiol Pharmacol 59:81–113
- Silva CM RA, Figueiredo SV, Goncalves AR, Veiga F. (2006). Alginate microspheres prepared by internal gelation: development and effect on insulin stability. Int J Pharm 311:1–10
- Sinha VR, Singla AK, Wadhawan S, et al. (2004). Chitosan microspheres as a potential carrier for drugs. Int J Pharm 274:1–33
- Sonaje K, Lin YH, Juang JH, et al. (2009). In vivo evaluation of safety and efficacy of self-assembled nanoparticles for oral insulin delivery. Biomaterials 30:2329–39
- Soppimath KS, Aminabhavi TM, Kulkarni AR, Rudzinski WE. (2001). Biodegradable polymeric nanoparticles as drug delivery devices. J Control Release 70:1–20
- Sun S, Liang N, Piao H, et al. (2010). Insulin-S.O (sodium oleate) complex-loaded PLGA nanoparticles: formulation, characterization and in vivo evaluation. J Microencapsul 27:471–8
- Sutradhar KB, Sumi CD. (2014). Implantable microchip: the futuristic controlled drug delivery system. Drug Deliv [early online]
- Tozuka Y, Sugiyama E, Takeuchi H. (2010). Release profile of insulin entrapped on mesoporous materials by freeze-thaw method. Int J Pharm 386:172–7
- Tuomilehto J, Lindström J, Eriksson JG, et al. (2001). Prevention of type 2 diabetes mellitus by changes in lifestyle among subjects with impaired glucose tolerance. New Engl J Med 344:1343–50
- Vinod KR, Kumar MS, Anbazhagan S, et al. (2012). Critical issues related to transfersomes – novel vesicular system. Acta Sci Pol Technol Aliment 11:67–82
- Wang J, Tabata Y, Morimoto K. (2006). Aminated gelatin microspheres as a nasal delivery system for peptide drugs: evaluation of in vitro release and in vivo insulin absorption in rats. J Control Release 113:31–7
- Wei W, Ma GH, Wang LY, et al. (2010). Hollow quaternized chitosan microspheres increase the therapeutic effect of orally administered insulin. Acta Biomater 6:205–9
- Woitiski CB, Neufeld RJ, Veiga F, et al. (2010). Pharmacological effect of orally delivered insulin facilitated by multilayered stable nanoparticles. Eur J Pharm Sci 41:556–63
- Xuan J, Lin Y, Huang J, et al. (2013). Exenatide-loaded PLGA microspheres with improved glycemic control: in vitro bioactivity and in vivo pharmacokinetic profiles after subcutaneous administration to SD rats. Peptides 46:172–9
- Ye Q, Asherman J, Stevenson M, et al. (2000). DepoFoam™ technology: a vehicle for controlled delivery of protein and peptide drugs. J Control Release 64:155–66
- Yin L, Ding J, He C, et al. (2009). Drug permeability and mucoadhesion properties of thiolated trimethyl chitosan nanoparticles in oral insulin delivery. Biomaterials 30:5691–700
- Yu L, Li C, Le Y, et al. (2011). Stabilized amorphous glibenclamide nanoparticles by high-gravity technique. Mater Chem Phys 130:361–6
- Zhang XG, Zhang HJ, Wu ZM, et al. (2008). Nasal absorption enhancement of insulin using PEG-grafted chitosan nanoparticles. Eur J Pharm Biopharm 68:526–34
- Zhang Y, Wei W, Lv P, et al. (2011). Preparation and evaluation of alginate-chitosan microspheres for oral delivery of insulin. Eur J Pharm Biopharm 77:11–19
- Zhao X, Zu Y, Zu SC, et al. (2010). Insulin nanoparticles for transdermal delivery: preparation and physicochemical characterization and in vitro evaluation. Drug Dev Ind Pharm 36:1177–85
- Zheng J, Yue X, Dai Z, et al. (2009). Novel iron–polysaccharide multilayered microcapsules for controlled insulin release. Acta biomaterialia 5:1499–507
- Zu Y, Zhang Y, Zhao X, et al. (2012). Preparation and characterization of chitosan-polyvinyl alcohol blend hydrogels for the controlled release of nano-insulin. Int J Bio Macromol 50:82–7