Abstract
The aim of this work was to research the potential functions and the mechanism of absorption of the baicalin (BC)-loaded micelle system that contained Pluronic P123 copolymer (P123) and sodium taurocholate (ST) as carrier materials via oral delivery. Based on the numerous advantages of oral administration, such as cost-effectiveness, flexible and accommodated dosing regimen, and improved compliance for patients, the ST-P123-MMs system would be evaluated as oral delivery vehicle of BC. In this study, X-ray powder diffractometer analysis confirmed the phase change of BC after being incorporated in mixed micelles. The release study in simulated gastric fluid/simulated intestinal fluid exhibited that BC-loaded ST-P123-MMs presented a sustained drug release behavior. Compared with coumarin-6 solution, higher cellar uptake efficiency was achieved for coumarin-6 loaded ST-P123-MMs towards Caco-2 cell lines. The in situ perfusion test in rat indicated that the absorption of BC-loaded ST-P123-MMs in intestinal tract was stronger than BC solution. After oral administration, the Cmax and AUC of BC-loaded ST-P123-MMs were 1.77 times and 1.54 times as high as those of BC suspension in rat, respectively. Promisingly, the formulated BC exhibited a prolonged circulation time with the oral bioavailability increased to 1.54-fold compared with the control group. These results all suggested that P123 and ST mixed micelles could serve as a promising approach to oral administration of BC.
Introduction
Baicalin (BC), one of the major bioactive flavone glucuronides, was extracted from the radix of scutellaria baicalensis. It has extensive pharmacological activities such as anti-inflammatory, anti-fever, anti-bacterial and anti-allergic diseases (Qi et al., Citation1998; Cheng et al., Citation2001; Nakamura et al., Citation2003; Baek et al., Citation2014). However, the application of BC in clinic is still limited due to its poor solubility, low permeability, and minimal oral bioavailability (Shen et al., Citation2003; Tsai & Tsai, Citation2004).
Polymeric micelles with a core–shell structure could provide a hydrophobic core as reservoir to increase the solubility of water-insoluble drugs (Shin et al., Citation2009; Yokoyama, Citation2010; Saxena & Hussain, Citation2012; Sarisozen et al., Citation2012; Riehle et al., Citation2013; Chen et al., Citation2014). P123, a synthetic amphiphilic polymer composed of poly(ethylene oxide) (PEO) and poly(propylene oxide) (PPO) blocks arranged in triblock structure PEO-PPO-PEO, could self-assemble into micelles in aqueous solution. Hydrophobic PPO segments formed inner core is able to realize high drug-loading and entrapment efficiency, and hydrophilic PEO segments formed outer shell can avoid the recognition and elimination by the reticuloendothelial system (Zhang et al., Citation2009, Citation2010). Additionally, bile salts could also self-assemble into micelles in aqueous solution (Bottari et al., Citation1999; Sugioka & Moroi, Citation1999). Based on the rationale, mixed micelles have distinctive advantages over conventional individual micelles by demonstrating increased aqueous solubility, enhanced stability, prolonged circulation time, and improved bioavailability of drugs (Gao et al., Citation2005). Many studies proved that bile salts and other amphipathic molecules (e.g., phospholipid and polyvinyl pyrrolidone) could form the stable mixed micelles system (Yu et al., Citation2010; Dong et al., Citation2013; Zhu et al., Citation2014). Likewise, the mixed micelles containing P123, such as Pluronic L81/P123, Pluronic P123/F127, and Pluronic P123/F68, were also widely investigated for drug delivery (Kulthe et al., Citation2011; Zhang et al., Citation2011; Zhao et al., Citation2012; Saxena & Hussain, Citation2013). Our previous study showed that the ST-P123-MMs system was a promising platform for drug delivery, which could be attributed to nano-size, slow release characteristic, high cytotoxicity, and so on (Zhang et al., Citation2014). Recently, many researches suggested that micelles might be uniquely useful for the oral delivery of water-insoluble drugs due to the suitable particle size which induced excellent potential of intercellular permeability and uptake through membrane internalization (Yano et al., Citation2010). Therefore, this study aimed to research the potential functions and illustrate the absorption mechanism of BC-loaded ST-P123-MMs by the pharmacokinetics, in situ intestine absorption, Caco-2 cells uptake experiments.
Materials and methods
Materials and animals
BC was purchased from D&B (Shanghai, China). ST was purchased from Solarbio (Beijing, China). P123 (Mn ∼ 5800) and coumarin-6 were purchased from Sigma-Aldrich (St. Louis, MO). RPMI 1640 were purchased from Thermo Fisher (Beijing, China). Trypsin-EDTA was purchased from Gibco (Waltham, MA). Fetal bovine serum (FBS) was purchased from Hangzhou Sijiqing Biological Engineering Materials Co., Ltd. (Zhejiang, China). All other chemicals in this study were of analytical grade.
Human colonic cancer cell lines (Caco-2 cells) were donated by Institute of Biochemical and Biotechnological Drug, School of Pharmaceutical Sciences, Shandong University.
Healthy male Wistar rats (200 ± 20 g) were supplied by Laboratory Animals Center of Shandong University, Jinan, China. The animals were used following the guidelines of the Ethical Committee for Animal experiments of Shandong University. All animals were acclimatized at a temperature of 25 ± 2 °C and a relative humidity of 70 ± 5% under natural light/dark conditions for 2 weeks before experiments
Preparation and characterization of the mixed micelles
BC-loaded ST-P123-MMs were prepared by a solvent evaporation method (Abouzeid et al., Citation2014; Zhang et al., Citation2014). ST-P123-MMs as blank micelles were prepared using the same method without adding BC. Besides, coumarin-6 instead of BC as a fluorescence probe was encapsulated into ST-P123-MMs to evaluate the Caco-2 cell uptake of this mixed micelle system.
BC, ST-P123-MMs, and BC-loaded ST-P123-MMs solutions were prepared and lyophilized to obtain corresponding freeze-dried powders. Then BC and ST-P123-MMs freeze-dried powders were blended to obtain BC+ST-P123-MMs physical mixture. The physical state of BC, ST-P123-MMs, BC+ST-P123-MMs physical mixture and BC-loaded ST-P123-MMs was assessed via XRPD analysis with a D/MAX 2500-PC X-ray powder diffractometer (Rigaku, Tokyo, Japan) (Li et al., Citation2011; Moes et al., Citation2011; Li et al., Citation2013; Yue et al., Citation2013). A Cu-Kа source operation (40 kV, 40 mA) was employed. The diffraction patterns were recorded over a 2θ angular range of 9–90° with a step size of 0.4° in 2θ and a 1 s count per step at room temperature.
In vitro drug release
The release tests in vitro of BC from BC-loaded ST-P123-MMs and BC propylene glycol solution were conducted by the dialysis method (Dahmani et al., Citation2012; Guo et al., Citation2012; Zhao et al., Citation2012; Dou et al., Citation2014; Duan et al., Citation2014). Simulated gastric fluid (SGF, pH 1.2) and simulated intestinal fluid (SIF, pH 6.8) were used as the release media. About 400 μL of BC-loaded ST-P123-MMs solution and BC propylene glycol solution as control were added to dialysis bags (molecular weight cutoff 3.5 kDa, Solarbio, Beijing, Japan), which were immersed in 100 mL of release medium at 37 ± 0.5 °C and shaken at a speed of 100 rpm. After 2 h incubation of BC-loaded ST-P123-MMs and BC propylene glycol solution in SGF, the bags were moved into SIF up to 48 h. About 1 mL of solution was taken out and fresh release medium at equal volume was replenished to maintain the constant volume (100 mL) at the predetermined intervals. Then, these samples were filtered with 0.22 μm filters and assayed by the UV–Vis spectrophotometer at 317 nm. All results were obtained in triplicate measurements.
In situ intestine absorption experiments in rats
Intestine absorption experiment was conducted by single-pass intestinal perfusion technique (Jain et al., Citation2007; Li et al., Citation2009; Luo et al., Citation2013). Kerbs–Ringer buffer solution as the intestinal perfusion solution was prepared by dissolving NaCl (7.8 g), CaCl2 (0.37 g), KCl (0.35 g), MgCl2 (0.02 g), NaH2PO4 (0.22 g), and glucose (1.4 g) in purified water (1000 mL) (Li et al., Citation2009). BC and BC-loaded ST-P123-MMs were diluted by the Kerbs–Ringer buffer as the control group and the experimental group at three concentrations of low (27 μg/mL), middle (54 μg/mL), and high concentration (108 μg/mL) of BC. The study was conducted in 18 male Wistar rats treated with BC solution and BC-loaded ST-P123-MMs at three different drug concentrations. These rats were fasted for 12 h prior to experiment but allowed freely to water. After weighing, rats were anaesthetized and kept in the whole course of the experiment. A midline longitudinal incision was made at the abdomen and the whole intestinal segment was selected. The intestinal segment was cannulated in both ends and linked by a constant-current pump (HL-2 constant-current pump, Jiapeng, Shanghai, China) and perfusion fluid to form a loop pathway, the perfusion fluid being stored in graduated cylinder. The animals were maintained constant body temperature by a light of an infrared lamp to maintain at 37 °C and the incision was covered with gauze filled with isotonic saline. The intestinal segment was rinsed with saline solution until the outlet solution was visually clear and then perfused at a constant flow rate of 1.0 mL/min. Samples were taken out from perfusion fluid at 0, 15, 30, 45, 60, 120, 180, 240, and 360 min into EP tubes and survival volumes were recorded. Equal amount of fresh liquid was added to maintain constant volume of perfusion fluid. Finally, the animals were euthanized with a cardiac injection of saturated potassium chloride solution. Samples were filtered with 0.22 μm filters and determined via the UV–Vis spectrophotometer at 317 nm.
Uptake of coumarin-6-loaded ST-P123-MMs by Caco-2 cells
Caco-2 cell lines were used in cell uptake study to simulate intestinal absorption in vivo for oral chemotherapy (Feng et al., Citation2009). The cell lines were incubated in tissue culture flasks and placed in incubator with a humidified environment of 5% CO2 at 37 °C. The mixture of 80% RPMI 1640 and 20% fetal bovine serum was used as the nutrient medium and replaced every 3 d. After reaching confluence, the cells were digested by Trypsin-EDTA and collected. The Caco-2 cells were cultured in 12-well plates at a density of 5 × 103 cells per well and reached to appropriate confluence. Then the cells were divided into two groups and incubated in nutrient mediums containing coumarin-6 and coumarin-6-loaded ST-P123-MMs, the concentration of coumarin-6 being 10 μg/mL. Then the 12-well plates were put back into an incubator. After 2 h, the medium was removed and the cells were washed three times with cold PBS (pH 7.4) to eliminate the residual coumarin-6 outside the cells. The uptake of coumarin-6-loaded ST-P123-MMs and coumarin-6 solution by Caco-2 cells was observed under fluorescence microscope (FLM, Olympus, Tokyo, Japan).
In vivo pharmacokinetics (PK) studies
All rats were divided into two groups randomly as the control group and the experimental group in PK studies (Wu et al., Citation1999; Yin et al., Citation2009; Hao et al., Citation2012; Khatun et al., Citation2013). The control group received intragastric administration of BC suspended in 0.5% (w/v) sodium carboxyl methyl cellulose (CMC-Na) solution (Zhu et al., Citation2014), and the experimental group received intragastric administration of BC-loaded ST-P123-MMs solution. Blood samples were drawn from subclavian vein at 0.5, 1, 2, 4, 6, 8, 10, 12, 24, and 48 h into collecting tubes containing heparin.
Plasma samples were obtained by centrifugation of blood at 12 000 rpm for 10 min and stored at −20 °C before HPLC analysis. BC in the plasma samples was extracted into 1 mL of acetonitrile and the acetonitrile was evaporated under nitrogen atmosphere. Then 100 μL of methanol was added to redissolve BC and centrifuged at 12 000 rpm for 10 min. About 20 μL of the supernatant was injected into the column of HPLC. The HPLC system (Agilent 1200 liquid chromatograph, Agilent Technologies, Santa Clara, CA) was equipped with a UV detector (Agilent G1314-60100, Agilent Technologies, Santa Clara, CA) and reversed phase column (Elite ODS C-18, 4.6 mm × 250 mm, Dalian Elite Analytical Instruments Co., Ltd., Dalian, China). The wavelength of the UV detector was set at 278 nm with a flow rate of 0.8 mL/min. A degassed mixed solution of chromatographic methanol and water (50/50, v/v) with 0.2% phosphoric acid was used as the mobile phase for the determination of plasma drug concentration.
Statistical analysis
The statistical significance of differences among more than two groups was determined by one-way ANOVA. A value of p < 0.05 was considered to be significant.
Results and discussion
Characterization of BC-loaded ST-P123-MMs
As reported in our previous work (Zhang et al., Citation2014), the prepared BC-loaded ST-P123-MMs exhibited the average size (15.60 nm), zeta potential (−5.26 mV), drug loading (DL, 16.94%), and entrapment efficiency (EE, 90.67%). The capacity of the polymer micelles to increase aqueous solubility of hydrophobic molecules is due to their hydrophobic core providing a suitable microenvironment for a hydrophobic solute (Bromberg, Citation2001, Citation2002). Phase states of BC, ST-P123-MMs, BC+ST-P123-MMs physical mixture, and BC-loaded ST-P123-MMs lyophilized powders were analyzed by XRPD (Zhang et al., Citation2014). In , several clear crystal diffraction peaks at the 2θ angle position of 12.4°, 14.6°, 16.9°, 20.6°, 27.9°, and 29.4° demonstrated the presence of the crystal phase of BC. The same diffraction peaks in the BC+ST-P123-MMs physical mixture illustrated that the crystal state of the drug still existed. ST-P123-MMs group appeared no characteristic diffraction peaks because of the absence of BC. The disappearance of the characteristic peaks of BC in BC-loaded ST-P123-MMs suggested that the phase state of BC had changed and the drug was highly dispersed in the carrier materials (Saxena & Hussain, Citation2013).
In vitro release of BC from mixed micelles
The in vitro release experiment of BC was conducted in different pH conditions (SGF, pH 1.2; SIF, pH 6.8) to simulate gastrointestinal tract in vivo. exhibited the release profiles of BC from BC-loaded ST-P123-MMs and BC-propylene glycol in SGF/SIF (Guo et al., Citation2012; Zhao et al., Citation2012; Duan et al., Citation2014). As shown in this figure, 33.92% of BC was released from propylene glycol in SGF within 2 h and then 79.34% in SIF within 48 h. The release rate of BC from BC-loaded ST-P123-MMs was 14.12% in SGF within 2 h and then 54.28% in SIF within 48 h, which showed sustained release. During the process of drug release, the micelles system may experience three stages including swelling/erosion, diffusion, and the degradation of carrier materials (Mu et al., Citation2005). The sustained release behavior of BC-loaded ST-P123-MMs might be attributed to the fact that the drug inside the hydrophobic core of micelles was protected from the direct impact of external environment. However, because simulative physiological condition cannot absolutely simulate real environment in vivo, it is unknown whether the release in vitro was consistent with characteristic of micelles in vivo after oral administration. Therefore, the BC-loaded mixed micelles should be further examined and evaluated in vivo (Pal & Nayak, Citation2012; Ukawala et al., Citation2012; Akhtar et al., Citation2014).
In situ intestine absorption experiments in rats
Intestinal perfusion experiment in situ was carried out to explore the intestinal absorption of BC-loaded mixed micelles. To study the effect of drug concentration on the intestinal absorption, low (27 μg/mL), middle (54 μg/mL), and high (108 μg/mL) concentrations were set in this study. The drug residual amount was changed along with the intestinal absorption. Recording directly the volume method was applied to calculate the remaining drug amount (Stewart et al., Citation1997; Liao et al., Citation2005). According to the formula of lnX = lnX0 − Ka × t (Ka are absorption rate constants, t is the perfusing time, X is the residual drug amount, and X0 is the initial drug amount), the intestinal absorption kinetics equations of BC-loaded ST-P123-MMs were indicated as follows: lnXL = lnX0 − 0.0789t, lnXM = lnX0 − 0.0993t, and lnXH = lnX0 − 0.0528t at low (27 μg/mL), middle (54 μg/mL), and high concentration (108 μg/mL) of BC, respectively. The equations of BC solution were lnXL = lnX0 − 0.027 t, lnXM = lnX0 − 0.0218t, and lnXH = lnX0 − 0.0254t at the same three drug concentrations. Besides, t1/2 (t1/2 = 0.693/Ka) of the BC solution and BC-loaded ST-P123-MMs are listed in . The percentage of absorption (P %) was calculated by the ratio of drug uptake amount/initial drug amount. All the correlation coefficients were greater than 0.95. The results showed that both absorption rate constants (Ka) and percentage of absorption (P) values of BC solution in the range of 27–108 mg/mL had no significant differences (p > 0.05), e.g. a concentration-independent change. However, the absorption of drugs increased over time, which suggested that the intestinal transport mechanism of the mixed micelles was passive diffusion (Li et al., Citation2009). Absorption kinetics of BC solution and BC-loaded ST-P123-MMs are shown in . At three different drug concentrations, both Ka and P values of BC-loaded mixed micelles were higher than those of BC solution, which indicated that the mixed micelles preparation could improve the intestinal absorption of drug. This may be explained by the small size and surface hydrophilicity of mixed micelles as well as the inhibition of P123 on P-glycoprotein (P-gp) efflux (Alakhov et al., Citation1996; Batrakova et al., Citation2001).
Figure 3. The absorption curves of BC solution (a) and BC-loaded ST-P123-MMs (b) in the rat intestine in situ for 6 h with various concentrations. Each data represents the mean ± standard deviation of three rats.
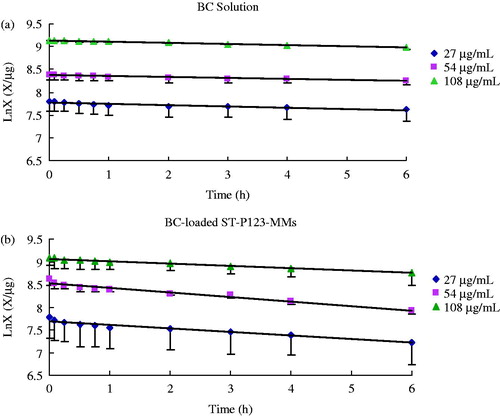
Table 1. Intestinal absorption kinetic parameters of BC solution and BC-loaded ST-P123-MMs in situ at different concentrations (results represent mean values ± SD, n = 3).
Uptake of coumarin-6-loaded ST-P123-MMs by Caco-2cells
In vitro cell uptake studies were performed to evaluate the effect of mixed micelles on loading drug permeability. Many methods have been employed to study the interaction of delivery system and intestinal membrane. Caco-2 cell, a cloned human colon cancer cells functionalized with many brush border enzymes and transport proteins, could be used to simulate the intestinal absorption as the gold standard (Artursson & Karlsson, Citation1991; Artursson et al., Citation2001). Hence, the Caco-2 cell monolayer could act as a useful model to investigate the absorption of orally administered drugs. FLM images of Caco-2 cells after incubation with coumarin-6 solution (A) and coumarin-6-loaded ST-P123-MMs (B) are visualized in . The stronger fluorescence intensity of the coumarin-6-loaded ST-P123-MMs group than the control group illustrated that the mixed micelles delivered more coumarin-6 into the Caco-2 cells than coumarin-6 solution, which confirmed the great internalization ability of ST-P123-MMs. This result may be explained by the internalization of MMs triggered by non-specific interactions between the delivery system and cells such as hydrogen bonding, hydrophobic, or van der Waal interactions, or fluid-phase pinocytosis route. The small size also favors the diffusion through the mucus layer, thereby realizing promising enterocyte absorption for oral delivery of drugs.
Pharmacokinetic study
The pharmacokinetic study in vivo was conducted to study the oral bioavailability of BC. The pharmacokinetic parameters of the BC suspension group and the BC-loaded mixed micelles group via oral administration were processed by DAS 2.0 and are listed in . As shown in , the drug concentration in plasma of the BC-loaded mixed micelles was significantly higher than that of BC suspension, which may have a positive effect on therapeutic index. The Tmax value of BC-loaded mixed micelles (8 h) was higher than that of BC suspension (4.83 h). The plasma concentration of BC-loaded mixed micelles (13.68 μg/mL) was increased by 1.8-fold compared with that of BC suspension (7.73 μg/mL). Promisingly, as a key therapeutic index of drug, the AUC(0–48 h) value of BC-loaded mixed micelles (409.76 h·μg/mL) was increased by 1.5-fold than that of the BC suspension (266.19 h·μg/mL). The results suggested that BC-loaded mixed micelles could improve the oral absorption of BC, which might be attributed to the small size of mixed micelle system and inhibiting effect of P123 on the P-gp-mediated efflux (Kabanov et al., Citation2003; Wang et al., Citation2011; Zhu et al., Citation2014). The in vivo plasma concentration–time profiles of the BC suspension and BC-loaded mixed micelles are depicted in . The curves showed a bimodal phenomenon with the first peak at 8 h and the other at 12 h. This phenomenon could be ascribed to the enterohepatic circulation. After the oral absorption, BC was excreted by bile excretion into lumen and then hydrolyzed to baicalein by bacterias. The baicalein was reabsorbed in the body and recovered to its original form, BC (Akao et al., Citation2000; Liu & Jiang, Citation2006; Hao et al., Citation2012; Yue et al., Citation2013). Besides, double peaks phenomenon also appeared in the mixed micelles system containing bile salt (Dong et al., Citation2013). The above data had clearly demonstrated that BC-loaded MMs had the ability to enhance oral bioavailability of the BC. The oral bioavailability of BC-loaded micelle was increased 1.54-fold than that of free BC in rats. The improved oral bioavailability of BC-loaded MMs might be attributed to enhanced drug solubility and stability in the gastrointestinal tract and the relatively small size (Jung et al., Citation2000; Behrens et al., Citation2001; Wang et al., Citation2011; Nair et al., Citation2014). The internalization of mixed micelles can be activated by non-specific interactions between the carrier materials and the intestinal cells to make the drug-loaded mixed micelles be absorbed intactly (Norris et al., Citation1998). Based on the results of the pharmacokinetic study, the time of maximum concentration (Tmax) in the rats was pro-longed 1.66-fold, which might be closely related to its long circulation time (Dahmani et al., Citation2012). In conclusion, the results demonstrated that the BC-loaded ST-P123-MMs maintained high concentration of BC in the plasma to facilitate the oral bioavailability of BC.
Figure 5. Mean plasma concentration–time curves of BC suspension and BC-loaded ST-P123-MMs after oral administration (n = 3).
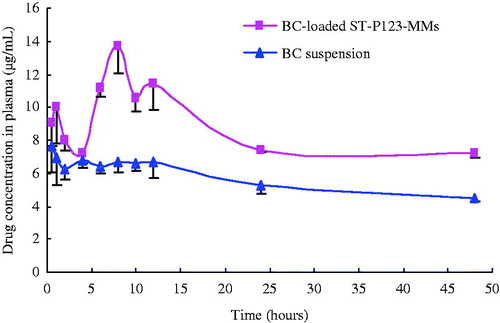
Table 2. Pharmacokinetic parameters of BC suspension and BC-loaded ST-P123-MMs via oral administration (results represent mean values ± SD, n = 3).
Conclusion
In this study, we evaluated the as-developed BC-loaded ST-P123-MMs in vitro and in vivo. According to XRPD analysis, the phase change of BC after being incorporated into BC-loaded ST-P123-MMs confirmed the previous deduction of DSC and TGA. Compared with the BC solution, higher absorption of BC-loaded ST-P123-MMs in intestinal perfusion experiment was achieved. Cell uptake test showed that the ST-P123-MMs system could be effectively internalized by the Caco-2 cells and locate inside the cells. PK experiment in vivo indicated that the BC-loaded ST-P123-MMs system performed high oral bioavailability. Taken together, the ST-P123-MMs system can be considered as a potent platform for the oral delivery of water-insoluble drugs.
Declaration of interest
This work is supported by the National Nature Science Foundation (Nos. 81171766 and 81373896) and the Natural Science Foundation of Shandong Province, China (No. ZR2011HM026).
References
- Abouzeid AH, Patel NR, Torchilin VP. (2014). Polyethylene glycol-phosphatidylethanolamine (PEG-PE)/vitamin E micelles for co-delivery of paclitaxel and curcumin to overcome multi-drug resistance in ovarian cancer. Int J Pharm 464:178–84
- Akao T, Kawabata K, Yanagisawa E, et al. (2000). Balicalin, the predominant flavone glucuronide of Scutellariae radix, is absorbed from the rat gastrointestinal tract as the aglycone and restored to its original form. J Pharm Pharmacol 52:1563–8
- Akhtar M, Imam SS, Ahmad MA, et al. (2014). Neuroprotective study of Nigella sativa-loaded oral provesicular lipid formulation: in vitro and ex vivo study. Drug Deliv 21:487–94
- Alakhov VY, Moskaleva E, Batrakova EV, Kabanov AV. (1996). Hypersensitization of multidrug resistant human ovarian carcinoma cells by pluronic P85 block copolymer. Bioconjug Chem 7:209–16
- Artursson P, Karlsson J. (1991). Correlation between oral drug absorption in humans and apparent drug permeability coefficients in human intestinal epithelial (Caco-2) cells. Biochem Bioph Res Co 175:880–5
- Artursson P, Palm K, Luthman K. (2001). Caco-2 monolayers in experimental and theoretical predictions of drug transport. Adv Drug Deliver Rev 46:27–43
- Baek JS, Hwang CJ, Jung HW, et al. (2014). Comparative pharmacokinetics of a marker compound, baicalin in KOB extract after oral administration to normal and allergic-induced rats. Drug Deliv 21:453–8
- Batrakova EV, Li S, Vinogradov SV, et al. (2001). Mechanism of pluronic effect on P-glycoprotein efflux system in blood–brain barrier: contributions of energy depletion and membranefluidization. J Pharmacol Exp Ther 299:483–93
- Behrens I, Stenberg P, Artursson P, Kissel T. (2001). Transport of lipophilic drug molecules in a new mucus-secreting cell culture model based on HT 29-MTX cells. Pharm Res 18:1138–45
- Bottari E, Festa MR, Franco M. (1999). Composition of sodium taurocholate micellar solutions. Analyst 124:887–92
- Bromberg L. (2001). Hydrophobically modified polyelectrolytes and polyelectrolyte block- copolymers. In: Nalwa HS, ed. Handbook of surfaces and interfaces of materials. San Diego: Academic Press, 369–404
- Bromberg L. (2002). Hydrophobically modified polyelectrolytes and polyelectrolyte block-copolymers for biomedical applications. In: Tripathy SK, Kumar J, Nalwa HS, eds. Handbook of polyelectrolytes and their applications. Los Angeles, CA: American Scientific Publishers, 23–46
- Chen D, Yu H, Sun K, et al. (2014). Dual thermoresponsive and pH-responsive self-assembled micellar nanogel for anticancer drug delivery. Drug Deliv 21:258–64
- Cheng GQ, Feng NP, Tang QW, Li L. (2001). Studies on in vitro antibacterial action of baicalin in vitro. J Chin Hosp Pharm J 21:347–8
- Dahmani FZ, Yang H, Zhou J, et al. (2012). Enhanced oral bioavailability of paclitaxel in pluronic/LHR mixed polymeric micelles: preparation, in vitro and in vivo evaluation. Eur J Pharm Sci 47:179–89
- Dong F, Xie Y, Qi J, et al. (2013). Bile salt/phospholipid mixed micelle precursor pellets prepared by fluid-bed coating. Int J Nanomed 8:1653–63
- Dou J, Zhang H, Liu X, et al. (2014). Preparation and evaluation in vitro and in vivo of docetaxel loaded mixed micelles for oral administration. Colloid Surface B 114:20–7
- Duan Y, Wang J, Yang X, et al. (2014). Curcumin-loaded mixed micelles: preparation, optimization, physicochemical properties and cytotoxicity in vitro. Drug Deliv 22:1–8
- Feng SS, Mei L, Anitha P, et al. (2009). Poly(lactide)-vitamin E derivative/montmorillonite nanoparticle formulations for the oral delivery of docetaxel. Biomaterials 30:3297–306
- Gao, ZG, Fain HD, Rapoport N. (2005). Controlled and targeted tumor chemotherapy by micellar-encapsulated drug and ultrasound. J Control Release 102:203–22
- Guo C, Yang C, Li Q, et al. (2012). Development of a Quercetin-loaded nanostructured lipid carrier formulation for topical delivery. Int J Pharm 430:292–8
- Hao J, Wang F, Wang X, et al. (2012). Development and optimization of baicalin-loaded solid lipid nanoparticles prepared by coacervation method using central composite design. Eur J Pharm Sci 47:497–505
- Jain R, Duvvuri S, Kansara V, et al. (2007). Intestinal absorption of novel-dipeptide prodrugs of saquinavir in rats. Int J Pharm 336:233–40
- Jung T, Kamm W, Breitenbach A, et al. (2000). Biodegradable nanoparticle for oral delivery of peptides: is there a role for polymers to affect mucosal uptake. Eur J Pharm Biopharm 50:147–60
- Kabanov AV, Batrakova EV, Miller DW. (2003). Pluronic block copolymers as modulators of drug efflux transporter activity in the blood–brain barrier. Adv Drug Deliv Rev 55:151–64
- Khatun Z, Nurunnabi M, Reeck GR, et al. (2013). Oral delivery of taurocholic acid linked heparin–docetaxel conjugates for cancer therapy. J Control Release 170:74–82
- Kulthe, SS, Inamdar, NN, Choudhari, YM, et al. (2011). Mixed micelle formation with hydrophobic and hydrophilic Pluronic block copolymers: implications for controlled and targeted drug delivery. Colloid Surf B 88:691–6
- Li B, Wen M, Li W, et al. (2011). Preparation and characterization of baicalin-poly–vinylpyrrolidone coprecipitate. Int J Pharm 408:91–6
- Li C, Zhang D, Guo H, et al. (2013). Preparation and characterization of galactosylated bovine serum albumin nanoparticles for liver-targeted delivery of oridonin. Int J Pharm 448:79–86
- Li H, Zhao X, Ma Y, et al. (2009). Enhancement of gastrointestinal absorption of quercetin by solid lipid nanoparticles. J Control Release 133:238–44
- Liao ZG, Ping QN, Xiao W, Liang XL. (2005). The study in situ on rat intestinal absorption of the active components in GuizhiFuling capsule. Chin J Nat Med 3:303–7
- Liu TM, Jiang XH. (2006). Investigation of the absorption mechanisms of baicalin and baicalein in rats. J Pharm Sci 95:1326–33
- Luo Z, Liu Y, Zhao B, et al. (2013). Ex vivo and in situ approaches used to study intestinal absorption. J Pharmacol Toxicol 68:208–16
- Moes JJ, Koolen SLW, Huitema ADR, et al. (2011). Pharmaceutical development and preliminary clinical testing of an oral solid dispersion formulation of docetaxel (ModraDoc001). Int J Pharm 420:244–250
- Mu L, Teo MM, Ning HZ, et al. (2005). Novel powder formulations for controlled delivery of poorly soluble anticancer drug: application and investigation of TPGS and PEG in spray-dried particulate system. J Control Release 103:565–75
- Nair AB, Attimarad M, Al-Dhubiab BE, et al. (2014). Enhanced oral bioavailability of acyclovir by inclusion complex using hydroxypropyl-β-cyclodextrin. Drug Deliv 21:540–7
- Nakamura N, Hayasaka SJ, Zhang XY. (2003). Effects of baicalin, baicalein, and wogonin on interleukin-6 and interleukin-8 expression, and nuclear factor-kb binding activities induced by interleukin-1b in human retinal pigment epithelial cell line. Exp Eye Res 77:195–202
- Norris DA, Puri N, Sinko PJ. (1998). The effect of physical barriers and properties on the oral absorption of particulates. Adv Drug Deliv Rev 34:135–54
- Pal D, Nayak AK. (2012). Novel tamarind seed polysaccharide–alginate mucoadhesive microspheres for oral gliclazide delivery: in vitro–in vivo evaluation. Drug Deliv 19:123–31
- Qi L, Zhou R, Wang YF, Zhu YC. (1998). Study of major flavonoids in crude Scutel-lariae radix by micellar electrokinetic capillary chromatography. J Capillary Electrophor 5:181–3
- Riehle, RD, Cornea S, Degterev A, Torchilin V. (2013). Micellar formulations of pro-apoptotic DM-PIT-1 analogs and TRAIL in vitro and in vivo. Drug Deliv 20:78–85
- Sarisozen C, Vural I, Levchenko T, et al. (2012). Long-circulating PEG-PE micelles co-loaded with paclitaxel and elacridar (GG918) overcome multidrug resistance. Drug Deliv 19:363–70
- Saxena V, Hussain MD. (2012). Poloxamer 407/TPGS mixed micelles for delivery of gambogic acid to breast and multidrug-resistant cancer. Int J Nanomed 7:713–21
- Saxena V, Hussain MD. (2013). Formulation and in vitro evaluation of 17-allyamino-17-demethoxygeldanamycin (17-AAG) loaded polymeric mixed micelles for glioblastoma multiforme. Colloid Surf B 112:350–5
- Shen YC, Chiou WF, Chou YC, Chen CF. (2003). Mechanisms in mediating the anti-inflammatory effects of baicalin and baicalein in human leukocytes. Eur J Pharmacol 465:171–81
- Shin HC, Alani AW, Rao DA, et al. (2009). Multi-drug loaded polymeric micelles for simultaneous delivery of poorly soluble anticancer drugs. J Control Release 140:294–300
- Stewart BH, Chan OH, Jezyk N, Fleisher D. (1997). Discrimination between drug candidates using models for evaluation of intestinal absorption. Adv Drug Deliv Rev 23:27–45
- Sugioka H, Moroi Y. (1999). Micelle formation of sodium cholate and solubilization into the micelle. Biochim Biophys Acta 1394:99–110
- Tsai PL, Tsai TH. (2004). Pharmacokinetics of baicalin in rats and its interactions with cyclosporin A, quinidine and SKF-525A: a microdialysis study. Planta Med 70:1069–74
- Ukawala M, Rajyaguru T, Chaudhari K, et al. (2012). Investigation on design of stable etoposide-loaded PEG-PCL micelles: effect of molecular weight of PEG-PCL diblock copolymer on the in vitro and in vivo performance of micelles. Drug Deliv 19:155–67
- Wang T, Wang N, Song H, et al. (2011). Preparation of an anhydrous reverse micelle delivery system to enhance oral bioavailability and anti-diabetic efficacy of berberine. Eur J Pharm Sci 44:127–35
- Wu J, Chen D, Zhang R. (1999). Study on the bioavailability of baicalin–phospholipid complex by using HPLC. Biomed Chromatogr 13:493–5
- Yano K, Masaoka Y, Kataoka M, et al. (2010). Mechanisms of membrane transport of poorly soluble drugs: role of micelles in oral absorption processes. J Pharm Sci 99:1336–45
- Yin YM, Cui FD, Mu CF, et al. (2009). Docetaxel microemulsion for enhanced oral bioavailability: preparation and in vitro and in vivo evaluation. J Control Release 140:86–94
- Yokoyama M. (2010). Polymeric micelles as a new drug carrier system and their required considerations for clinical trials. Expert Opin Drug Deliv 7:145–158
- Yu JN, Zhu Y, Wang L, et al. (2010). Enhancement of oral bioavailability of the poorly water-soluble drug silybin by sodium cholate/phospholipid-mixed micelles. Acta Pharmacol Sin 31:759–64
- Yue PF, li, Y., Wan, J., et al. (2013). Process optimization and evaluation of novel baicalin solid nanocrystals. Int J Nanomed 8:2961–2973
- Zhang HQ, Zhao LL, Chu LJ, et al. (2014). Preparation, optimization, characterization and cytotoxicity in vitro of Baicalin-loaded mixed micelles. J Colloid Interf Sci 434:40–7
- Zhang W, Hao, JG, Shi Y, et al. (2009). Paclitaxel-loaded Pluronic P123/F127 mixed polymeric micelles: formulation, optimization and in vitro characterization. Int J Pharm 376:176–85
- Zhang W, Shi Y, Chen Y, et al. (2011). Multifunctional Pluronic P123/F127 mixed polymeric micelles loaded with paclitaxel for the treatment of multidrug resistant tumors. Biomaterials 32:2894–906
- Zhang W, Shi Y, Chen YZ, et al. (2010). Enhanced antitumor efficacy by Paclitaxel-loaded Pluronic P123/F127 mixed micelles against non-small cell lung cancer based on passive tumor targeting and modulation of drug resistance. Eur J Pharm Biopharm 75:341–53
- Zhao L, Du J, Duan Y, et al. (2012). Curcumin loaded mixed micelles composed of Pluronic P123 and F68: preparation, optimization and in vitro characterization. Colloid Surf B 97:101–8
- Zhu Y, Peng W, Zhang J, et al. (2014). Enhanced oral bioavailability of capsaicin in mixed polymeric micelles: preparation, in vitro and in vivo evaluation. J Funct Foods 8:358–66