Abstract
Nano-delivery systems have significantly evolved over the last decade for the treatment of cancer by enabling site-specific delivery and improved bioavailability. The widely investigated nanoparticle systems are biodegradable polyesters, dendrimers, liposomes, mesoporous silica and gold nanoparticles. These particles when conjugated with different targeting motifs enhance the therapeutic efficiency of the drug molecules and biocompatibility. However, the application of such systems towards the treatment of retinoblastoma (RB), a rapidly spreading childhood eye cancer, still remains in its infancy. Nanoparticle-based systems that have been investigated for RB therapy have displayed improved drug delivery to the most restricted posterior segment of the eyes and have increased intra-vitreal half-life of the chemotherapy agents highlighting its potential in treatment of this form of cancer. This review focuses on the challenges involved in the treatment of RB and highlights the attempts made to develop nano-dimensional systems for the treatment of RB.
Introduction
Retinoblastoma (RB) is a type of frequently encountered childhood ocular cancer (Gombos et al., Citation2007a). Its incidence is prevalent among children under 5 years with a frequency of 1 in 15 000 (Bishop & Madsen, Citation1975). Though this form of cancer has a high survival rate of about 95%, the progression of this disease can lead to severe vision disorders and also death, if left untreated (Leal-Leal et al., Citation2004). There also exists an anomaly in the survival rates of patients across continents. The patient survival and cure is reported to be very high in the United States with a mortality rate of about 4–5%. In contrast, the mortality rate in Asian and African countries lies between 39 and 70% (Desjardins et al., Citation2000). This vast difference in the patient survival rates arises primarily due to insufficient health measures and lack of early detection of this type of cancer (Rodriguez-Galindo et al., Citation2008). Late diagnosis leads to poor prognosis for patient survival and as a result the cancer progresses rapidly into the metastatic and terminal stages in nearly 90% of the RB cases in developing countries (Kivelä, Citation2009). Following detection, immediate medical intervention involving a multi-disciplinary team comprising oncologists, ophthalmologists, radiologists and geneticists with appropriate chemotherapy, radiotherapy and surgical interventions is required over long term for improved patient survival (Abramson, Citation2005).
From a histological perspective, the onset of RB has been attributed to the development of immature retinal cells and subsequent replacement of the retina and other intra-ocular tissues. A high mitotic rate and many regions of necrosis and dystrophic calcification are considered hallmarks of RB (Knudson, Citation1971; Meel et al., Citation2012). According to the “two-hit” hypothesis proposed by Alfred Knudson in 1971, the onset of RB may occur through mutations either in the germinal stage that affects all retinal cells or it can occur in specific retinal cells leading to alterations in cell cycle resulting in improper entry into S phase of cell cycle (Knudson, Citation1971; Chintagumpala et al., Citation2007). RB may occur sporadically or may be inherited. A salient feature of the inherited RB is that both mutations happen before fertilization and consequently the onset of cancer occurs at a younger age and usually develops into a bilateral RB (Knudson, Citation1971). In the sporadic form of RB, the somatic mutations are common and both mutations happen in a single retinal cell after fertilization. As a result, this type of cancer generally occurs in the later years and is not usually transmitted to the offspring (Van Quill et al., Citation2003).
Genetically, RB is characterized by the abnormal phenotypic expression or loss of the tumor suppressor gene RB1 present at 13q14.2 chromosome (Friend et al., Citation1986). RB occurs only when both copies of RB1 gene have abnormal functioning or are entirely lost (Mitter et al., Citation2011). These events occur before or during the fertilization phase and therefore lead to the development of an inheritable bilateral RB (Ramsubramanian & Shields, Citation2012). Several studies have however demonstrated that all bilateral RB cases are not hereditary. De novo mutations occurring during spermatogenesis can also lead to bilateral RB (Dryja et al., Citation1989; Zhu et al., Citation1989). In the sporadic form of RB, the mutations occurring in a single retinal cell post-fertilization develops into unilateral RB (Pandey, Citation2013). Though it was believed that all unilateral RB arise due to somatic mutations, it is now recognized that in about 15% cases, unilateral RB may originate due to hereditary germinal mutations (Aerts et al., Citation2006). The alteration in the coloration of strabismus and pupil red reflex are currently employed as indicators of possible onset of RB (Ruben et al., Citation2008). Additionally, techniques like fundoscopy, ultrasonography of the eye, and magnetic resonance imaging (MRI) help in identifying the presence of white creamy mass and intra- and extra-ocular extensions commonly associated with RB (Smith et al., Citation1990; Karcioglu et al., Citation1997). Genetic counseling and identification of prone family members can help in early diagnosis and also provide knowledge about the risk involved in having progeny with inherited RB (Chintagumpala et al., Citation2007).
Current treatment strategies for RB and their short-comings
A plethora of options are available for treatment of RB. These include enucleation, external beam radiotherapy, episcleral plaque radiotherapy, cryotherapy, chemoreduction and recently, intra-arterial chemotherapy (IAC; ). In advanced RB, enucleation is the only option, which reduces mortality and does not lead to any systemic complications (Balmer et al., Citation2006). However, the lesion results in facial deformity (Chang et al., Citation2011). Early stage RB has been treated using external beam radiotherapy. But this strategy has been accompanied by impairment of vision for the rest of the life necessitating cataract surgery and frequent change of multiple glasses (Hernandez et al., Citation1996). Radiotherapy can also result in dry eye condition that requires the use of eye lubrication permanently for the remainder of the life (Sussman et al., Citation2003). Foggy vision, orbito-facial dimorphism and corneal vascularization are other adverse effects that have been reported for radiotherapy (Roarty et al., Citation1988). Several studies also suggest that the children with RB due to germline mutation, when exposed to radiation during radiotherapy, have increased risk of developing secondary cancers (Wong et al., Citation1997). Chemotherapy is an extensively employed form of treatment strategy for RB. Anti-cancer drugs such as melphalan, topotecan, vincristine, etoposide or carboplatin have been used individually or in combination to treat RB. However, the adverse effects associated with the use of these highly cytotoxic agents persist leading to secondary complications (Samuel, Citation2011). Chemoreduction overcomes several problems associated with other therapeutic strategies, but produces new complications that are more severe. These include generation of secondary cancer (mostly leukemia), renal toxicity and temporary pancytopenia (Demirci et al., Citation2005; Shields et al., Citation2006). In some cases, chemoreduction has also been reported to permanently impair the normal hearing (Friedman et al., Citation2000; Gombos et al., Citation2007b). One of the major impediments in the use of chemotherapeutic agents for RB therapy is presented by the blood–retinal barrier (BRB), which limits the entry of different chemotherapeutic molecules into the tumor thereby reducing their efficacy (Lambert et al., Citation2008). A recent stratagem that has been introduced for treatment of RB with an aim to circumvent the challenges posed by the existence of the BRB is the IAC (Balderrama et al., Citation2013). In this procedure, the chemotherapeutic agent is introduced into the eye through a 4-Fr pediatric arterial catheter. The catheter is initially introduced into the femoral artery, passed through the abdomen followed by the internal carotid artery and then finally through the ophthalmic artery to transfer the chemotherapeutic agent into the eye (Shields & Shields, Citation2010a). However, local delivery of anti-cancer drugs using IAC has also been associated with several adverse effects like stroke, loss of limb movement, loss of vision, hemorrhage, and in some cases, death (Shields & Shields, Citation2010b).
Challenges in the treatment of retinoblastoma
The distinct anatomical and physiological arrangement of the eye presents a major obstacle for delivery of drugs to diseased regions in the eye (Yamane et al., Citation2004). The BRB, which is generally involved in the maintenance of homeostasis in the eye, represents another barrier for the transport of drug molecules into the eye () (Short, Citation2008). Retinal capillaries, retinal pigment, choroid and sclera are the main parts of the BRB which maintain the solute transport from blood to the retina. Like the brain, retina is part of central nervous system. Hence the normal functioning of retina and extremely critical high rate of oxygen consumption and glycolysis are preserved by existence of tight junctions (Winkler, Citation1981; Yu & Cringle, Citation2001; Kim et al., Citation2006).
Ocular cancer therapy has employed different routes of administration for the anti-cancer agents such as systemic, topical, sub-conjunctive, sub-tenon and intra-vitreal delivery. Though these delivery routes have been found efficacious in treating the anterior portion of the eye, they have been ineffective for treating posterior regions of the eye where the RB originates (Nair et al., Citation2008). Apart from the above-mentioned challenges, the treatment of RB is further limited by the drug toxicity arising due to poor target specificity of these molecules (Lim et al., Citation1999). Surgical interventions require highly skilled surgeons and also pose the risk of endophthalmitis and retinal detachment (Izak et al., Citation2003; Shane & Martin, Citation2003). Implantable devices for ocular therapy also have been found to be ineffective due to dangers of calcification, adverse host–tissue response and impairment of vision (Tiwari et al., Citation2012). In the light of the current scenario, a paradigm shift in the approach for treatment of RB to improve treatment efficacy is the need of the hour.
Nanotechnology-based treatment options
The advent of nanotechnology has introduced a new facet towards treatment of diseases. Use of nano-dimensional structures that can entrap drug molecules has not only ensured sustained release of the drugs, but these carriers can also be surface-modified to impart target-specific delivery of their cargo. Nano-delivery systems can reduce the frequency of drug administration as well as reduce the adverse effects arising from the use of the free drug apart from improving its therapeutic efficacy by enhancing its bioavailability and cellular uptake (Ramana et al., Citation2010; Salmaso & Caliceti, Citation2013). A large body of literature exists on the development of novel drug delivery systems and their characterization. Most of the work has focused on target-specific delivery of anti-infectives and anti-cancer agents. Commercialization of several nano-delivery systems has been achieved heralding in a new era of nano-therapeutics (Barenholz, Citation2012; Oliveira et al., Citation2013). Surprisingly, nano-interventions for ocular therapy have been limited when compared to other diseases. The unique challenges offered by the intra-ocular milieu towards delivery of drugs may be one of the prime causes for the limited attempts in designing therapeutic strategies involving nano-dimensional materials. Several nano-carriers that have been reported for treatment of RB have been described in the following section.
Nano-delivery systems for treatment of RB
One of the key requisites of the delivery systems for RB is that they should exhibit enhanced permeation through the retinal pigment endothelial layer, which restricts the entry of free drugs into the tumor. Improved bioavailability, sustained and controlled release of the encapsulated drugs are other requirements of the delivery system designed to replace multiple surgeries. The loading of cytotoxic anti-cancer drugs into the nano-drug delivery system also serves to reduce the toxicity, non-specific interactions of the drug and also increases the solubility of poorly water-soluble drug molecules thereby contributing to their improved efficacy (Jabr-Milane et al., Citation2008) The choice of the carrier material is an important criterion that determines the success of the drug delivery system. Use of non-degradable matrices can give rise to additional complexities involving the elimination of such particles from the biological system after release of the drugs. Hence, most of the work on drug delivery systems has employed biodegradable carriers where the drug release can be correlated to the rate at which the delivery system degrades in the biological milieu. Most common mode of degradation of these matrices is through hydrolysis (Engineer et al., Citation2011). Both bulk as well as surface degrading systems had been reported in literature to tailor the drug availability at any given instant. The carrier employed should be biocompatible and it also should not lead to formation of toxic by-products on degradation (De Jong & Borm, Citation2008). Polymeric systems represent a major class of materials that have been explored for drug delivery applications due to their tunable degradation properties that can be exploited for highly regulated release of the drugs. lists the different nano-dimensional carrier systems that have been investigated for RB therapy.
Table 1. Different nanoparticulate systems reported for treatment of retinoblastoma.
Polymeric nano-delivery systems
Polymeric nanoparticles have been relatively more widely explored for treatment of RB when compared with other types of nanoparticles. depicts different nanocarriers that have been explored for the ocular delivery of anti-cancer agents for treatment of RB. Among the several biodegradable polymeric delivery systems, poly(lactic acid) (PLA) carriers (Anderson & Shive, Citation1997) have attracted considerable attention due to their biocompatibility and high entrapment efficiencies towards hydrophobic molecules. A study employing PLA nanoparticles encapsulating rhodamine dye as the model drug was carried out in Lewis rat models for delivery to the retina and retinal pigment endothelium (RPE). It was reported that a single intra-vitreal injection of PLA nanoparticles containing encapsulated rhodamine-6G (Rh) could diffuse through the retinal layers and localize in the RPE cells. This study also revealed that the PLA nanoparticles were retained in RPE upto 4 weeks. The RPE cells were stained by the Rh dye suggesting the release of Rh dye in the cells. The PLA nanoparticles, however, elicited mild inflammation in the ciliary body and posterior vitreous humor but the magnitude of inflammation reduced after 48 h (Bourges et al., Citation2003).
Figure 3. Different nanoparticles used for drug delivery to RB. Chemical structures are given in the top panel and nanoparticles formed by these molecules are depicted in the lower panel.
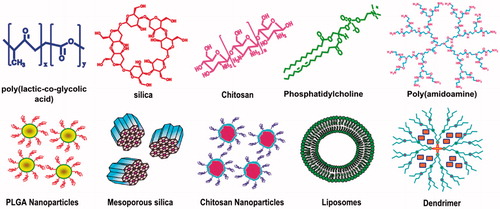
Hu et al. in 2007 reported the intra-vitreal delivery of doxorubicin (DOX)-loaded poly-β-hydroxybutyrate microspheres in rabbit ocular tissue. The microspheres exhibited a sustained release of DOX that was detected upto 10 d in ocular tissues. The sustained release of DOX from the microspheres resulted in a reduced amount of DOX in the ocular tissue that was in the therapeutic limit and did not attain toxic levels. This reduced the risk of undesired toxicity to the surrounding tissue (Hu et al., Citation2007). Kim et al. in Citation2009a have reported the development of another polyester-based system for the delivery of DOX. This group had employed nanoparticles of poly(l-lactide-co-glycolide) (PLGA) with an average diameter of 265 nm. The permeation of drug through the sclera was evaluated using a perfusion chamber. It was observed that the free drug exhibited a faster permeation when compared with the encapsulated DOX that displayed 29% diffusion across the sclera. This decrease was attributed to the sustained and slower release of the drug from the slow degrading PLGA matrix (Kim et al., 2009a). Such sustained release of a cytotoxic drug-like DOX from nanoparticles augers well for ocular therapy due to its reduced toxicity to normal tissues.
Carboplatin, a coordination complex containing platinum (II) as the central metal atom, has been employed as an anti-neoplastic agent due to its ability to interfere with DNA repair (Johnstone et al., Citation2014). However, the delivery of free carboplatin through sub-conjuctival injection was found to exhibit substantial toxicity to the surrounding tissues that was manifested in the form of ischemic necrosis, atrophy of the optic nerve and orbital fat necrosis (Schmack, Citation2006). Hyper-branched PAMAM dendritic nanoparticles were used to deliver carboplatin in an attempt to mitigate the toxic effects of the free drug. The experiment was carried out using LHβ-Tag mouse model induced with retinal tumors equivalent to human RB bilaterally. The results revealed that the 258 nm PAMAM particles were retained in the region of parenteral administration and were not transferred from the sclera, choroid and RPE thereby facilitating release of the drug at the site of administration for a longer duration. The study also established the superiority of the carboplatin-loaded dendrimer system over the free drug in reducing the tumor volume in mice models. It was reported that a single dose of carboplatin-loaded nanoparticles showed significant reduction in tumor size than free carboplatin after 22 d of treatment. Interestingly, the high dose of carboplatin-loaded dendrimers exhibited a reduction in the tumor in the contralateral eye that was left untreated. This was ascribed to the long retention periods of the high dose of nanoparticles facilitating the transport of the drug through the vasculature to the contralateral eye (Kang et al., Citation2009). Chitosan nanoparticles prepared by ionic gelation technique were developed for the delivery of DOX and were targeted to Y79 RB cell line expressing high folate receptor levels (Parveen & Sahoo, Citation2010). The findings suggested an enhanced toxicity of the folate-targeted nanoparticles when compared to their unmodified counterparts. This variation arises due to differences in the cell uptake between the targeted and non-targeted nanoparticles. This study highlights the importance of targeting strategies to increase the specificity of nanoparticles and reduce the non-specific interactions of the drug. A recent study had reported EpCAM antibody tagged gold-poly(ethyleneimine) nanoparticles encapsulated with EpCAM si-RNA that exhibited increased cell uptake and significant down-regulation of the EpCAM expression levels in Y79 RB cell line underlying the importance of targeting for effective treatment of cancers (Moutushy et al., Citation2013).
A micellar system comprising the biodegradable polymer PLGA and the hydrophilic poly(ethylene glycol) (PEG) was developed to deliver DOX (Boddu et al., Citation2010). The surface of the PLGA-PEG-PLGA micelles was modified with folic acid to target the folate receptor over-expressed in Y79 RB cells. This micellar delivery system exhibited a sustained release of DOX upto 2 weeks and about four-fold increased cell uptake when compared with the free drug indicating its potential to be employed for targeted delivery of anti-cancer agents to RB cells through an intra-vitreal route of administration. Another report on PLGA nanoparticles employed the emulsion-solvent evaporation technique to prepare etoposide-loaded PLGA nanoparticles. An encapsulation of about 86% of the drug was achieved in the nanoparticles that were around 240 nm in diameter and had a slightly cationic surface with a zeta potential of +19 mV. A sustained release of etoposide was observed over a period of 40 d and an IC50 value of 0.2 μg/mL was recorded in Y79 cells exposed to the nanoparticles (Moutushy et al., Citation2011). The nanoparticle delivery was also found to decrease the mitochondrial membrane potential. In addition, microarray experiments carried out demonstrated an up-regulation of 171 genes after treatment with the etoposide-loaded nanoparticles and also a down-regulation of 280 genes that included several well-known oncogenes. Further studies in this direction can serve to understand the role of nanoparticles on the expression levels of key genetic markers that are related with the progression of RB (Moutushy et al., Citation2011).
In another approach, a Chinese herbal medicine, celastrol that had been previously reported to be effective against several tumor cell lines was explored for its efficacy against RB. Celastrol was encapsulated in poly(ethylene glycol)-b-poly(ɛ-caprolactone) nanoparticles (Li, Citation2012). It was reported that the delivery of celastrol promoted apoptosis in SO-Rb50 human RB cells in a dose-dependent manner while reducing the expression of anti-apoptotic proteins such as Bcl-2 and transcriptional activators such as NF-κB and p65. The in vivo studies carried out using RB xenograft mice model also showed a significant decrease in tumor volume and tumor weight in the nanoparticle-treated group.
Multidrug resistance (MDR) caused by different proteins has emerged as a major obstacle in the treatment of various cancers. The ABC membrane transporter family protein (MRP-1) and lung resistance protein (LRP) have been extensively investigated for their role in the development of MDR in RB (Chearwae et al., Citation2006a,Citationb). It has now been recognized that Bcl-2 and NFκB proteins also play a substantial role in the development of MDR in RB (Das & Sahoo, Citation2012). In a recent report, folate tagged PLGA nanoparticles encapsulating curcumin and a chemotherapeutic drug nutlin-3a were developed in an effort to reverse MDR and promote cancer cell death. Nutlin-3a is an imidazole analogue that was chosen for its role in activation of p53 tumor suppressor protein and its non-genotoxic nature while curcumin was used as MDR modulator. The experiments were performed using Y79 cells and a down-regulation of the expression levels of MDR proteins MRP-1 and LRP, anti-apoptotic proteins and cell survival proteins was noted. The results revealed a synergistic effect between curcumin and nutlin-3a and have opened up new vistas in the treatment of RB through reversal of MDR induced by MDR proteins (Das & Sahoo, Citation2012).
One of the challenges in development of cancer therapeutics is the lack of correlation between the results obtained from in vitro experiments and in vivo studies. This ambiguity arises due to the absence of a 3D architecture in vitro as encountered in solid tumors. An interesting study for understanding the behavior of RB cells and their response to the chemotherapeutic drugs in a tumor-mimetic environment employed PLGA-gelatin particle-based scaffold to develop a 3D tumor model with Y79 RB cells (Moutushy et al., Citation2012). The gelatin enhanced the agglomeration of 2–3 microparticles that enabled aggregation of Y79 to form a tumor-like morphology. The cells were treated with different nanoparticles such as chitosan-carboplatin, PLGA-etoposide and PLGA-DOX. It was observed that the IC50 values of the native drug and drugs encapsulated in nanoparticles were higher in the 3D model when compared with the cells grown in the cell culture plate. Similar differences have also been encountered in vivo suggesting the necessity of a tumor-mimetic environment for better translation of therapeutic strategies in vivo. The cDNA and microRNA microarray studies also indicated significant changes in gene and microRNA expression in the cells in the 3D environment when compared to those cultured in the conventional 2D environment in culture plates. These findings emphasize the importance of appropriate models to design effective therapeutic strategies in solid tumor management (Moutushy et al., Citation2012).
Liposomal delivery systems
Liposomes are self-assembled vesicular structures formed from amphipathic phospholipids and can encapsulate both hydrophobic and hydrophilic entities (Nounou et al., Citation2006; Mohan et al., Citation2014). Liposomes are amenable to surface modifications and have been widely used for delivery of chemotherapeutic agents. In the context of RB, very few attempts have been made using liposomal systems and are discussed in this section. Multilamellar vesicles (MLV) of about 100 to 600 nm encapsulating the fluorescent probes coumarin-6 or fluorescein isothiocyanate (FITC)-tagged polystyrene were administered to rodent and primate models through topical route of administration (Inokuchi et al., Citation2009). The results showed an improved coumarin-6 delivery to retina when the liposomal dimensions were reduced to submicron sizes. The rigidity of the liposomes was also identified as an important parameter that influences its entry into eye. Increasing the cholesterol content in the liposomes was found to make them more rigid. This study showed that administration of liposomal carriers significantly increased fluorescence in the regions surrounding the ganglion cell layer (GCL) and inner plexiform layer (IPL) in rabbit retina. The results also revealed a rapid uptake of liposomes within 30 to 60 min in the retina. In another study, liposomes of 90–110 nm size were formulated using l-α-distearoyl phosphatidylcholine (DSPC) or egg phosphatidylcholine (EPC) and the fluorescent molecule carboxyfluorescein (CF) was encapsulated with about 50% efficiency. The efficacy of this system was evaluated using human conjunctival cell line for retinal delivery. The fluorescence intensity monitored using laser scanning confocal microscopy was found to increase in the following order: Free CF < CF-loaded EPC-liposomes < CF-loaded DSPC-liposomes (Hironaka et al., Citation2011). Epifluorescence microscopy analysis of intra-ocular performance of liposomes after 10, 30, and 60 min of incubation revealed that the DSPC-liposomes exhibited greater fluorescence on retinal surface than EPC-liposomes suggesting that the liposomes formulated with DSPC have higher stability and rigidity that aid sustained release of the encapsulated hydrophilic molecule. Further in-depth studies are however warranted for exploiting the benefits of this carrier system in the treatment of RB.
Other non-polymeric nanoparticles
Various studies using nanoparticles suggest that the diffusion of the nanoparticle in the body depends on their size, shape and composition (Shang et al., Citation2014). These factors may also have a key role in the transport of the nanoparticles across the BRB. It has been demonstrated that gold nanoparticles of about 20-nm dimension administered intravenously were able to cross the BRB effectively (Kim et al., Citation2009b). The transmission electron micrographs revealed the distribution of these gold nanoparticles into retinal endothelial cells, retinal neurons, astrocytes and RB cells without exhibiting any significant cytotoxicity. Further, these particles did not cause any structural aberrations or changes in the expression of the tight junction protein ZO-1 in retinal endothelial cells. This indicates the potential of smaller sized particles in treatment of RB by overcoming the BRB.
Recently, highly oriented mesoporous silica nanoparticles (MSN) have also been evaluated for RB treatment. Camptothecin-loaded mesoporous nanoparticles functionalized with the monosaccharide galactose or mannose in combination with one photon or two photon excitation photosensitizers for photodynamic therapy (OPE-PDT and TPE-PDT) were assessed for treatment of RB using Y79 cells (Gary-Bobo et al., Citation2012; Gallud et al., Citation2013). Cell uptake and cell death with targeted nanoparticles were higher than non-targeted nanoparticles. OPE-PDT irradiation using mannose functionalized camptothecin-loaded MSN exhibited significant cell death in Y-79 cells when compared to the blank nanoparticles. Further studies are warranted to identify the potential of other non-polymeric nanocarriers for treatment of RB.
Imaging modalities in retinoblastoma
Imaging of RB with high resolution and sensitivity can facilitate rapid and accurate diagnosis. Several nanoparticle-based systems have been explored to advance imaging abilities of conventional imaging systems, especially for cancer. However, limited research has been carried out using nano-systems to improve conventional ophthalmic imaging like MRI, optical coherence tomography (OCT) and ultrasound imaging (Kharchenko et al., Citation2013). Quantum dots, nanoshells and magnetic iron oxide nanoparticles have been employed for imaging different forms of cancer and may have the potential for improving fluorescent and MRI of RB (Nair et al., Citation2008). However, this facet remains largely unexplored in the context of RB. Multi-modal nano-systems are emerging as a new paradigm in imaging cancers. Jaidev et al. have reported the synthesis of fluorescent iron oxide (FIO) nanoparticles that showed promise for both fluorescent as well as MRI (MRI) of RB in in vitro models (Jaidev et al., Citation2014). While MRI is used for tissue-level imaging, the incorporation of a fluorophore enables cell-level imaging (Hoffman, Citation2002). The iron oxide nanoparticles were surface modified with oleic acid and the fluorescent dye sulphorhodamine B. Iron oxide is known for its contrast enhancement property for T2 imaging in MRI. FIO displayed higher T2 contrast behavior both in MRI phantom gel assay and in vitro in Y79 RB cells (Jaidev et al., Citation2014). Integration of drug delivery as well as imaging capability in a single nano-dimensional structure represents the next generation of “smart” nanoparticles that can aid in simultaneous monitoring and treatment of cancers. Intensive research in this direction may lead to emergence of a new category of “theranostic” systems that can enable diagnosis as well as treatment of RB.
A recent study has reported the use of a fluorophore-tagged aptamer for imaging cancer cells. Nithya et al. used a novel epithelial cell adhesion molecule (EpCAM) aptamer conjugated with Alexa-fluor 594 (AF594) fluorescent probe tagged alkyne (alkyne-DIBO) for the imaging of EpCAM expressing cancer cell lines MCF7, MDA-MB-453, Weri-RB1 and PC3. Their observation shows the promising target specific detection of cancer cells in EpCAM-expressing cancers. RB has also been found to express EpCAM and hence the use of EpCAM aptamer conjugated-nano-systems could serve as an effective stratagem for imaging as well as target-specific delivery of anti-cancer agents (Nithya et al., Citation2014).
Conclusion
In the past decade, nanotechnology has made giant strides in treatment of various cancers and several nano-delivery systems have reached different phases of clinical trials. The present knowledge about treatment of RB using nanoparticles with different size, shape, and composition has displayed enormous promise in increasing the bioavailability of the drug, breaking the formidable BRB and reducing their non-specific interactions. However, such studies are few and the parameters influencing their therapeutic efficacy have not been completely identified. Hence, concerted efforts are required to clearly define the influence of the physico-chemical properties of the nanoparticles and the route of administration on the therapeutic potential of these systems towards combating RB.
Acknowledgements
The authors wish to acknowledge funding from Department of Biotechnology Government of India and SASTRA University for infrastructural support.
Declaration of interest
This study was funded by Department of Biotechnology Government of India (BT/PR11210/NNT/28/2008) and SASTRA University for infrastructural support.
References
- Abramson DH. (2005). Retinoblastoma in the 20th century: past success and future challenges. The Weisenfeld Lecture. Invest Ophthalmol Vis Sci 46:2684–91
- Aerts I, Rouic LL, Gauthier-villars M, et al. (2006). Retinoblastoma. Orphanet J Rare Dis 31:1–11
- Anderson JM, Shive MS. (1997). Biodegradation and biocompatibility of PLA and PLGA microspheres. Adv Drug Deliv Rev 28:5–24
- Balderrama J, Carlos Leal-Leal A, Alvis-Miranda H, et al. (2013). Intra-arterial chemotherapy for retinoblastoma: a practical review. Rom Neurosurg 20:327–33
- Balmer A, Zografos L, Munier F. (2006). Diagnosis and current management of retinoblastoma. Oncogene 38:5341–9
- Barenholz Y. (2012). Doxil® – the first FDA-approved nano-drug: lessons learned. J Control Rel 160:117–34
- Bishop JO, Madsen EC. (1975). Retinoblastoma: review of current status. Surv Ophthalmol 19:342–66
- Boddu HS, Jwala J, Chowdhury MR, Mitra AK. (2010). In vitro evaluation of a targeted and sustained release system for retinoblastoma cells using doxorubicin as a model drug. J Ocul Pharmacol Therap 26:459–68
- Bourges J, Gautier SE, Delie F, et al. (2003). Ocular drug delivery targeting the retina and retinal pigment epithelium using polylactide nanoparticles. Invest Ophthalmol Vis Sci 44:3562–9
- Chang JW, Yu YS, Kim JY, et al. (2011). The clinical outcomes of proton beam radiation therapy for retinoblastomas that were resistant to chemotherapy and focal treatment. Korean J Ophthalmol 25:387–93
- Chearwae W, Wu CP, Chu HY, et al. (2006a). Curcuminoids purified from turmeric powder modulate the function of human multidrug resistance protein 1(ABCC1). Cancer Chemother Pharmacol 57:376–88
- Chearwae W, Wu CP, Chu HY, et al. (2006b). Modulation of the function of the multidrug resistance-linked ATP-binding cassette transporter ABCG2 by the cancer chemopreventive agent curcumin. Mol Cancer Ther 5:1995–2006
- Chintagumpala M, Chevez-Barrios P, Paysse EA, et al. (2007). Retinoblastoma. Rev Curr Manage 12:1237–46
- Das M, Sahoo SK. (2012). Folate decorated dual drug loaded nanoparticle: role of curcumin in enhancing therapeutic potential of nutlin-3a by reversing multidrug resistance. PLoS One 7:e329202012
- De Jong WH, Borm PJ. (2008). Drug delivery and nanoparticles: applications and hazards. Int J Nanomedicine 3:133–49
- Demirci H, Shields CL, Meadows AT, Shields JA. (2005). Long-term visual outcome following chemoreduction for retinoblastoma. Arch Ophthalmol 123:1525–30
- Desjardins L, Levy C, Lumbroso L, et al. (2000). Current treatment of retinoblastoma. 153 children treated between 1995 and 1998. J Fr Ophtalmol 23:475–81
- Dryja TP, Mukai S, Petersen R, et al. (1989). Parental origin of mutations of the RB gene. Nature 339:556–8
- Engineer C, Parikh J, Raval A. (2011). Review on hydrolytic degradation behavior of biodegradable polymers from controlled drug delivery system. Trends Biomater Artif Organs 25:79–85
- Friedman DL, Himelstein B, Shields CL, et al. (2000). Chemoreduction and local ophthalmic therapy for intraocular retinoblastoma. J Clin Oncol 18:12–17
- Friend SH, Bernards R, Rogelj S, et al. (1986). A human DNA segment with properties of the gene that predisposes to retinoblastoma and osteosarcoma. Nature 323:643–6
- Gallud A, Silva AD, Maynadier M, et al. (2013). A functionalized nanoparticles for drug delivery, one- and two-photon photodynamic therapy as a promising treatment of retinoblastoma. J Clin Exp Ophthalmol 4:1000288
- Gary-Bobo M, Mir Y, Rouxel C, et al. (2012). Multifunctionalized mesoporous silica nanoparticles for the in vitro treatment of retinoblastoma: drug delivery, one and two-photon photodynamic therapy. Int J Pharm 432:99–104
- Gombos DS, Chevez-Barrios AP. (2007a). Current treatment and management of retinoblastoma. Curr Oncol Rep 9:453–8
- Gombos DS, Hungerford J, Abramson DH, et al. (2007b). Secondary acute myelogenous leukemia in patients with retinoblastoma. Is chemotherapy a factor? Ophthalmology 114:1378–83
- Hernandez JC, Brady LW, Shields JA, et al. (1996). External beam radiation for retinoblastoma: results, patterns of failure, and a proposal for treatment guidelines. Int J Radiat Oncol Biol Phys 35:125–32
- Hironaka K, Fujisawa T, Sasaki H, et al. (2011). Fluorescence investigation of the retinal delivery of hydrophilic compounds via liposomal eyedrops. Bio Pharma Bull 34:894–7
- Hoffman R. (2002). Green fluorescent protein imaging of tumour growth, metastasis, and angiogenesis in mouse models. Lancet Oncol 9:546–56
- Hu T, Le Q, Wu Z, Wu W. (2007). Determination of doxorubicin in rabbit ocular tissues and pharmacokinetics after intravitreal injection of a single dose of doxorubicin-loaded poly-beta-hydroxybutyrate microspheres. J Pharm Biomed Anal 43:263–9
- Inokuchi Y, Hironaka K, Fujisawa T, et al. (2009). Physicochemical properties affecting retinal drug/coumarin-6 delivery from nanocarrier systems via eyedrop administration. Invest Ophthalmol Vis Sci 51:3162–70
- Izak AM, Werner L, Pandey SK, Apple DJ. (2003). Calcification of modern foldable hydrogel intraocular lens designs. Eye 17:393–406
- Jabr-Milane LS, Van Vlerken LE, Yadav S, Amiji MM. (2008). Multifunctional nanocarriers to overcome tumor drug resistance. Cancer Treat Rev 34:592–602
- Jaidev LR, Bhavsar DV, Sharma U, et al. (2014). Engineered multifunctional nanomaterials for multimodal imaging of retinoblastoma cells in vitro. J Biomater Sci Polym Ed 25:1093–109
- Johnstone TC, Park GY, Lippard SJ. (2014). Understanding and improving platinum anticancer drugs phenanthriplatin. Anticancer Res 34:471–6
- Kang SJ, Durairaj C, Kompella UB, et al. (2009). Subconjunctival nanoparticle carboplatin in the treatment of murine retinoblastoma. Arch Ophthalmol 127:1043–7
- Karcioglu ZA, al-Mesfer SA, Abboud E, et al. (1997). Workup for metastatic retinoblastoma: a review of 261 patients. Ophthalmology 104:307–12
- Kharchenko S, Adamowicz J, Wojtkowski M, Drewa T. (2013). Optical coherence tomography diagnostics for onco-urology: review of clinical perspectives. Cent Eur J Urol 66:136–41
- Kim JH, Kim JH, Park JA, et al. (2006). Blood–neural barrier: intercellular communication at glio-vascular interface. J Biochem Mol Biol 39:339–45
- Kim ES, Durairaj C, Kadam RS, Lee SJ, Mo Y. (2009a). Geroski DH, Kompella UB, Edelhauser HF. Human scleral diffusion of anticancer drugs from solution and nanoparticle formulation. Pharmaceut res. 26, 1155–61
- Kim JH, Kim JH, Kim KW, et al. (2009b). Intravenously administered gold nanoparicles pass through the blood–retinal barrier depending on the particle size, and induce no retinal toxicity. Nanotechnology 20:505101
- Kivelä T. (2009). The epidemiological challenge of the most frequent eye cancer: retinoblastoma, an issue of birth and death. Br J Ophthalmol 93:1129–31
- Knudson AG. (1971). Mutation and cancer: statistical study of retinoblastoma. Proc Natl Acad Sci USA 68:820–3
- Lambert MP, Shields C, Meadows AT. (2008). A retrospective review of hearing in children with retinoblastoma treated with carboplatin-based chemotherapy. Pediatr Blood Cancer 50:223–6
- Leal-Leal C, Flores-Rojo M, Medina-Sansón A, et al. (2004). A multicentre report from the Mexican RB Group. Br J Ophthalmol 88:1074–7
- Li Z, Wu X, Li J, et al. (2012). Antitumor activity of celastrol nanoparticles in a xenograft retinoblastoma tumor model. J Nanomed 7:2389–98
- Lim JI, Wolitz RA, Dowling AH, et al. (1999). Visual and anatomic outcomes associated with posterior segment complications after ganciclovir implant procedures in patients with AIDS and cytomegalovirus retinitis. Am J Ophthalmol 127:288–93
- Meel R, Radhakrishnan V, Bakhshi S. (2012). Current therapy and recent advances in the management of RB. Indian Journal of Medical and Paediatric Oncology 33:80–8
- Mitter D, Ullmann R, Muradyan A, et al. (2011). Genotype-phenotype correlations in patients with retinoblastoma and interstitial 13q deletions. Eur J Hum Genet 19:947–58
- Mohan A, Shridhar N, Sethuraman S, Krishnan UM. (2014). Novel resveratrol and 5-fluorouracil coencapsulated in PEGylated nanoliposomes improve chemotherapeutic efficacy of combination against head and neck squamous cell carcinoma. BioMed Res, Early Online: 1–14. DOI: org/10.1155/2014/424239
- Moutushy M, Dilnawaz F, Misra R, et al. (2011). Toxicogenomics of nanoparticulate delivery of etoposide: potential impact on nanotechnology in retinoblastoma therapy. Cancer Nano 2:21–36
- Moutushy M, Kandalam M, Rangasamy J, et al. (2013). Novel epithelial cell adhesion molecule antibody conjugated polyethyleneimine-capped gold nanoparticles for enhanced and targeted small interfering RNA delivery to retinoblastoma cells. Mol Vis 19:1029–38
- Moutushy M, Mohanty C, Harila A, et al. (2012). A novel in vitro three-dimensional retinoblastoma model for evaluating chemotherapeutic drugs. Mol Vis 18:1361–78
- Nair A, Thevenot P, Hu W, Tang L. (2008). Nanotechnology in the treatment and detection of intraocular cancers. J Biomed Nanotechnol 4:410–18
- Nithya S, Jagdeesh Babu S, Baghavathi B, et al. (2014). A strain-promoted alkyne–azide cycloaddition (SPAAC) reaction of a novel EpCAM aptamer–fluorescent conjugate for imaging of cancer cells. Chem Com 50:11810–13
- Nounou NM, El-khordagui LK, Khalafallah NA, Khalil SA. (2006). In vitro release of hydrophilic and hydrophobic drugs from liposomal dispersions and gels. Acta Pharma 56:311–24
- Oliveira MF, Guimarães PPG, Gomes ADM, et al. (2013). Strategies to target tumors using nanodelivery systems based on biodegradable polymers, aspects of intellectual property, and market. J Chem Bio 6:7–23
- Pandey AN. (2013). Retinoblastoma: an overview. Saudi J Ophthalmol 28:310–15
- Parveen S, Sahoo SK. (2010). Evaluation of cytotoxicity and mechanism of apoptosis of doxorubicin using folate-decorated chitosan nanoparticles for targeted delivery to retinoblastoma. Cancer Nano 1:47–62
- Ramana LN, Sethuraman S, Ranga U, Krishnan UM. (2010). Development of a liposomal nanodelivery system for nevirapine. J Biomed Sci 17:57
- Ramsubramanian A, Shields CL. (2012). Retinoblastoma. New Delhi: Jaypee Brothers Medical Publisher
- Roarty JD, McLean IW, Zimmerman LE. (1988). Incidence of second neoplasms in patients with bilateral RB. Ophthalmology 95:1583–87
- Rodriguez-Galindo C, Wilson MW, Chantada G, et al. (2008). Retinoblastoma: one world, one vision. Pediatrics 122:e763
- Ruben JB, Buckley EJ, Ellis GS, et al. (2008). Red reflex examination in neonates, infants, and children. Pediatrics 122:1401–4
- Salmaso S, Caliceti P. (2013). Stealth properties to improve therapeutic efficacy of drug nanocarriers. J Drug Deliv 2013:374252
- Samuel KH. (2011). Current update on retinoblastoma. Int Ophthalmol Clin 51:77–91
- Schmack I, Hubbard GB, Kang SJ, et al. (2006). Ischemic necrosis and atrophy of the optic nerve after periocular carboplatin injection for intraocular retinoblastoma. Am J Ophthalmol 142:310–15
- Shane TS, Martin DF. (2003). Endophthalmitis after ganciclovir implant in patients with AIDS and cytomegalovirus retinitis. Am J Ophthalmol 136:649–54
- Shang L, Nienhaus K, Nienhaus GU. (2014). Engineered nanoparticles interacting with cells: size matters. J Nanobiotechnol 12:5
- Shields CL, Mashayekhi A, Au AK, et al. (2006). The International Classification of Retinoblastoma predicts chemoreduction success. Ophthalmology 113:2276–80
- Shields CL, Shields JA. (2010a). Review Intra-arterial chemotherapy for retinoblastoma: the beginning of a long journey. Clin Exp Ophthalmol 21:638–43
- Shields CL, Shields JA. (2010b). Retinoblastoma management: advances in enucleation, intravenous chemoreduction, and intra- arterial chemotherapy. Curr Opin Ophthalmol 21:203–12
- Short BG. (2008). Safety evaluation of ocular drug delivery formulations: techniques and practical considerations. Toxicol Pathol 36:49–62
- Smith EV, Gragoudas ES, Koloday NH, D’Amic DJ. (1990). Magnetic resonance imaging: an emerging technique for the diagnosis of ocular disorders. Int Ophthalmol 14:119–24
- Sussman DA, Escalona-Benz E, Benz MS, et al. (2003). Comparison of retinoblastoma reduction for chemotherapy vs external beam radiotherapy. Arch Ophthalmol 121:979–84
- Tiwari G, Tiwari R, Sriwastawa B, et al. (2012). Drug delivery systems: an updated review. Int J Pharm Investig 2:2–11
- Van Quill KR, O'Brien JM. (2003). The role of the retinoblastoma protein in health, malignancy, and the pathogenesis of RB. In: Albert DM, Polans A, eds. Ocular oncology. New York: Marcel Dekker, Inc., 103–28
- Winkler BS. (1981). Glycolytic and oxidative metabolism in relation to retinal function. J Gen Physiol 77:667–92
- Wong FL, Boice JD Jr, Abramson DH, et al. (1997). Cancer incidence after retinoblastoma radiation dose and sarcoma risk. J Am Med Assoc 278:1262–7
- Yamane T, Kaneko A, Mohri M. (2004). The technique of ophthalmic arterial infusion therapy for patients with intraocular retino- blastoma. Int J Clin Oncol 9:69–73
- Yu DY, Cringle SJ. (2001). Oxygen distribution and consumption within the retina in vascularised and avascular retinas and in animal models of retinal disease. Progr Retina Eye Res 20:175–208
- Zhu XP, Dunn JM, Phillips RA, et al. (1989). Preferential germline mutation of the paternal allele in retinoblastoma. Nature 340:312–13