Abstract
Context: Transporting drugs through the lymphatic system has attracted increasing attention. Lipid-based formulations have been proved to be an effective way to improve systemic bioavailability of highly lipophilic drugs by increasing intestinal lymphatic transport.
Objective: The formulation of polymer micelle was developed for probucol to improve its intestinal lymphatic transport.
Materials and methods: Methoxy-polyethylenelglycol-distearyl phosphatidyl-ethanolamine (mPEG-DSPE) polymer was chosen to develop the micelles for probucol. The physicochemical properties were characterized. Caco-2 cell model, unconscious and conscious lymph duct cannulated rat models were established for in vitro and in vivo evaluation of lymphatic transport.
Results: In vitro evaluation in the Caco-2 cell model showed that the micellar formulation could significantly increase the uptake and transport of probucol. The study in unconscious and conscious lymph duct cannulated rat models further verified the significant enhancement of lymphatic transport of probucol by mPEG-DSPE micelles.
Discussion and conclusion: These results suggested that mPEG-DSPE micellar formulation could provide a useful alternative approach for improving the lymphatic transport of hydrophobic compounds.
Introduction
Portal blood and lymph are two pathways for orally administered drugs access to the systemic circulation. Recently, transporting drug through the lymphatic system has attracted increasing attention because of avoidance of hepatic first-pass metabolism, prolonged time course of drug delivery to the systemic circulation, direct targeting of the lymphoid tissue and indirect targeting of specific sites associated with low-density lipoprotein receptors. The lymphatic transport in intestinal absorption of drug plays a significant role in this way (Yáñez et al., Citation2011; Fan et al., Citation2013).
The key mechanism of intestinal lymphatic transfer is the association of the lipophilic drug with chylomicrons in the enterocyte and subsequent uptake of these drug-containing large lipoproteins by the intestinal lymphatic system (Aji Alex et al., Citation2011; Shahnaz et al., Citation2011). Based on this, there are two main strategies to promote the intestinal lymphatic drug transport. One is prodrug approach, and the other is formulation approach. Lymphatic transport could be enhanced by the design of lipophilic prodrug. The association of a drug with the chylomicron may be facilitated through the design of prodrug with increased lipophilicity, or with some structural functional groups, which encourage incorporation into the lymphatic biochemical pathways (Charman & Porter, Citation1996). Lipid-based formulation is another effective way to improve lymphatic transport of poorly water-soluble drug. The lipid vehicles may stimulate the production of chylomicrons and association between chylomicrons and lipophilic drug. Especially for the highly lipophilic drug, preparing into lipid-based formulation has been proved to be a more successful strategy to enhance lymphatic transport comparing with the prodrug approach (Caliph et al., Citation2000; O'Driscoll, Citation2002).
Lipid-based formulations include solution, suspension, triglyceride emulsion, micellar system and self-emulsifying drug delivery system, self-microemulsifying drug delivery system, self-nanoemulsifying drug delivery system, liposome, solid lipid nanoparticle and nanostructured lipid carrier (Nanjwade et al., Citation2011; Ali Khan et al., Citation2013). Cremophor–oleic acid mixed micelle, TPGS-oleic acid mixed micelle and an oleic acid microemulsion were proved to enhance the intestinal lymphatic transport of saquinavir (Griffin & O'Driscoll, Citation2006). The self-emulsifying drug delivery system based on PEGylated bile acids provided a controlled release system with significant improvement of the bioavailability of itraconazole in rats (Le Dévédec et al., Citation2013). The micellar systems containing oleic acid or lecithin are favorable in terms of maximizing intestinal lymphatic transport and therein overall absorption of lycopene (Faisal et al., Citation2010). Microemulsion containing Tween 80, ethanol and ethyl oleate could improve the solubility and oral absorption of quercetin, a poorly water-soluble drug (Gao et al., Citation2009).
Polymeric micelles (PMs) have gained considerable attention in the last two decades as a multifunctional nanotechnology-based delivery system for poorly water soluble drug. Typically PMs are formed from self-aggregation of amphiphilic polymers with the hydrophobic part of the polymer on the inside (core), which serves as a solubilization depot for lipophilic drug and hydrophilic part on the outside (shell), which protects the drug from inactivation in biological media (Dahmani et al., Citation2012; Lu & Park,Citation2013). PMs could be absorbed in their intact form via endocytosis due to their small particle size (<200 nm) (Nam et al., Citation2003). Moreover, PMs possess higher drug loading capacity, significantly lower critical micelle concentration values and greater thermodynamic stability than those of low-molecular weight surfactants micelles (Nishiyama & Kataoka, Citation2006). And the simple preparation process without organic solvents is also one of the important advantages of PMs (Han et al., Citation2006). Methoxy-polyethylenelglycol-distearyl phosphatidyl-ethanolamine (mPEG-DSPE) is generally used to prepare liposomes. Its potential in the preparation of micelles for the delivery of poorly soluble drugs has also been proved and thus received much recent attention (Yu et al., Citation2013).
In this work, probucol, a high lipophilic drug, was chosen as model drug. Probucol has been widely used in clinical practice for the prevention of the progression of atherosclerosis because this agent acts as a potent antioxidant (Li et al., Citation2009). In addition, probucol can stop progression of atherosclerotic plaques in carotid arteries. However, probucol is slightly absorbed in the gastrointestinal tract due to its poor solubility in water. From studies in rats, dogs and monkeys, it is known that probucol accumulates slowly in adipose tissue. Approximately 90% of probucol administered orally is unabsorbed. A PM delivery system with mPEG-DSPE was developed for probucol to improve its intestinal lymphatic transport. The effect of mPEG-DSPE micelles on the uptake and transport of probucol in vitro was investigated in Caco-2 cell. The unconscious and conscious lymph duct cannulated rat models were established for in vivo evaluation of lymphatic transport of the delivery system.
Materials and methods
Materials
The Caco-2 cells were obtained from the American Type Culture Collection (Rockville, MD). Fetal bovine serums (FBS), 0.25% Trypsin-EDTA, Dulbecco’s Modified Eagle’s medium (DMEM) with high glucose, l-glutamine, penicillin–streptomycin, non-essential amino acid and Hank’s balanced salt solution (HBSS) were purchased from Gibco Laboratory (Grand Island, NY). MPEG2000-DSPE was obtained from Merck (Schaffhausen, Switzerland). HPLC grade acetonitrile and methanol were purchased from Merck (Darmstadt, Germany). Deionized water was produced with a Milli-Q system from Millipore (Billerica, MA). Other reagents were of analytical or high-performance liquid chromatography (HPLC) grade.
Preparation and formulation optimization of probucol-loaded micelles
An amphiphilic material, mPEG2000-DSPE was used to prepare the probucol-loaded micelles by a film formation method. Briefly, probucol and mPEG-DSPE were weighed accurately and dissolved in acetonitrile. Then the solvent was evaporated under reduced pressure at 37 °C (Heidolph Laborota 4000; Heidolph Instruments, Schwabach, Germany) to form a thin film and the residual solvent remaining in the film was removed under vacuum at room temperature for 24 h. Subsequently, the dried film was dispersed in Hank's balanced salt solution by magnetic stirring (IKA RCT basic; IKA, Staufen, Germany) at 800 rpm in water bath at 37 °C to obtain probucol-loaded micelles. The micellar solution was filtered through a 0.22 μm filter membrane and freeze-dried.
The preparation parameters, including evaporating temperature, pore size of filter membrane, aqueous phase amount and ratio of drug and excipient were optimized by taking particle size, encapsulation efficiency and drug loading as indexes.
Characterization of micelles
The mean diameter and particle size distribution were measured by dynamic light scattering measurement using a NICOMP 380 ZLS Zeta Potential/Particle Sizer (PSS. Nicomp, Santa Barbara, CA) equipped with a 5 mW helium–neon laser at 632.8 nm.
Probucol is a nearly insoluble drug with high nonpolarity. The solubility of probucol is about 5 ng/mL in water at 25 °C. Most of the unencapsulated drug will precipitate. To evaluate the entrapment efficiency and drug loading, the free drug was separated from the micelles by filtration and ultrafiltration. Briefly, the micellar suspension was filtrated with 0.22 μm millipore filter to remove the drug precipitation. Then ultrafiltration was carried out with a centrifugal device (Amicon YM-3 k, Millipore, Billerica, MA) at 1000 g for 30 min at 4 °C to separate the free drug in solution. The amount of probucol in micellar solution before and after filtration and in ultrafiltrate was determined by reversed phase-HPLC method. The drug content before filtration was considered as the total drug used. The content after filtration minus the content in ultrafiltrate was considered as encapsulated drug in micelles. The encapsulation efficiency was defined as the ratio of the encapsulated drug in micelles to the total drug added, while the drug loading was expressed as the ratio of the weight of loaded drug and the weight of drug-loaded micelles.
Uptake and transport of micelles in Caco-2 monolayer
The cellular uptake and transport of micelles in the Caco-2 cell monolayers were investigated to clarify its absorption mechanism. Cell passages between 40 and 45 were used in the experiments. Cells were cultured routinely on 75 cm2 plastic culture flasks (Corning Costar, Cambridge, MA) in DMEM supplemented with 10% FBS, 1% non-essential amino acid, 1% l-glutamine, 100 IU/mL penicillin and 100 μg/mL streptomycin at 37 °C in an atmosphere of 5% CO2 and 90% relative humidity. For uptake experiments, cells were seeded in 12-wells plates at a density of 5 × 104 cells/cm2 and cultured for 14 days. As for the transport experiments, cells were seeded at the same density onto permeable polycarbonate inserts (0.4 μm pore size, 0.33 cm2, Millipore, MA) in 24-well tissue culture plates and cultured for 21 days. The integrity of the cell monolayers was evaluated by measuring transepithelial electrical resistance (TEER) values with an EVOM epithelial voltohmmeter (World Precision Instruments, Sarasota, FL) and the leakage of a paracellular transport marker, [3H]-mannitol. The cell inserts were employed in the experiments when the TEER was over 600 Ω cm2 and the permeability of mannitol was less than 0.23% of the dose/h/cm2, which indicated that cell monolayers were tight enough.
Before administration, the cell monolayers were firstly rinsed twice and pre-incubated with blank HBSS for 20 min. In the experiment of uptake, cell monolayers were subsequently incubated with HBSS containing micelles (25 μg/mL probucol, 0.05% mPEG-DSPE) for 30 min. After the medium was removed, the cell monolayer was rinsed with ice-cold HBSS for three times and scraped out into eppendorf tube with 1 mL deionized water containing 1% DMSO (v/v). The cells were disintegrated by ultrasonic cell disruptor (Scientz, Ningbo, China). One-half of the cell suspension was used to measure the content of protein by Coomassie Brilliant Blue method. The other was centrifuged and the supernatant was separated for the drug determination by HPLC.
In the experiment of transport, HBSS containing micelles was added to the apical side and HBSS containing DMSO (1%, v/v) was given to the basolateral side as the receiving medium. After incubation for 90 min, the receiving medium was harvested and analyzed.
The drug should be dissolved before dosing to the cells. Due to the extreme insolubility of probucol, 1% DMSO was used to prepare the probucol solution and mix solution of probucol and PEG-DSPE as the control in the Caco-2 cell experiment.
Animals
Male Wistars (280–320 g) were provided by Shanghai Institute of Materia Medica, Chinese Academy of Sciences (Shanghai, China, Protocol number for animal study: SYXK (Shanghai) 2003-0029). The rats were housed at a room temperature of 22 ± 2 °C and a relative humidity of 50 ± 10% and had ad libitum access to a standard diet and water except, wherever indicated. All animal procedures were conducted in accordance with appropriate regulatory standards as prescribed in the publication “Guide for the care and the use of the laboratory animals” (NIH Publication No. 92-93, revised 1985) and all the animal studies were approved and supervised by Animal Ethics Committee of Fudan University.
Mesenteric lymphatic transport of micelles in rats
The in vivo lymphatic transport of micelles was evaluated in both the anesthetized rat model and the conscious rat model. Triple-cannulated anesthetized rat model has advantages, such as easy to manipulation, low rate of experimental failure. Contrastly, the conscious rat model was better for the study of lymphatic transport due to having stomach emptying process, more sufficient lymph collecting and longer sampling time. However, the conscious rat model was difficult to manipulate and had high failure rate. Both the models were successfully established in this study to investigate the lymphatic transport of micelles.
Male SD rats were fasted for 12 h with free access to water. One hour before the mesenteric lymph duct cannulation, the rats were given 1 mL of peanut oil by intragastric administration to make the lymphatics visualization. The animals were anaesthetized by intraperitoneal injection of 50 mg/kg sodium pentobarbital and placed on a heated pad maintained at 37 °C during the operation. The mesenteric lymph duct was cannulated using the method of Edwards with some modifications (Edwards et al., Citation2001). Briefly, the duodenum was cannulated 1 cm below the pylorus using PE-50 tubing and secured with instant cyanoacrylate adhesive (Compont Shunkang, Beijing, China). The right jugular vein was cannulated with polyethylene tubing (PE-50) and the end of the cannula was at the level of the right atrium. The continuous intraduodenal infusion of normal saline at 2.5 mL/h was performed via a constant rate infusion pump (Bioanalytical Sysems, West Lafayette, IN) to flush any remaining oil and maintain hydration. After 2 h stabilization, the anesthetized rat model was obtained which could be used for the pharmacokinetic experiment.
As for the construction of the conscious rat model, the difference with the anesthetized model included three aspects. Firstly, the conscious rats could be administered by intragastric infusion with no need for duodenum cannulation. Secondly, the PE-50 tubing for cannulation was longer to tunnel under the skin along the side of the body and exteriorize at the dorsal part of the neck to provide the good flexibility and tensile strength for animal motility. Finally, the animals were transferred to clean cages after surgery to recover overnight (15–18 h). During this recovery period and the pharmacokinetic experiment, a lactated Ringer’s solution containing 5% dextrose was provided ad libitum. The mesenteric lymph cannula and the jugular vein cannula were connected until 1 h before the experiment to mimic the normal physiology, draining lymph into jugular vein.
For each model, 15 rats were randomly assigned to three groups administered with probucol suspension, mixture suspension of probucol and mPEG-DSPE and micellar solution at the dose of 6 mg/kg, respectively. Probucol suspension and mixture suspension were prepared with water. Lymph was continuously collected into Eppendorf tubes containing EDTA which were changed per hour till 8 h. All samples were reserved at −20 °C until analysis.
Quantification of probucol by RP-HPLC
The analysis of probucol in vitro and in vivo was carried out with an Agilent 1200 HPLC system (Santa Clara, CA) with UV detection at 242 nm. The reversed-phase column (Agilent Eclipse XDB-C8 column, 5 μm, 4.6 × 150 mm) with a security guard column (Phenomenex, Torrance, CA) was used at 25 °C. The mobile phase was a mixture of acetonitrile and water (94:6, v/v) containing 0.01% n-butylamine and 0.01% glacial acetic acid. With a flow rate of 1.0 mL/min, the retention time of probucol was approximately 7.3 min.
Amiodarone (8 μg/mL in methanol, 50 μL), the internal standard, was mixed with 100 μL of lymph sample thoroughly. Then 400 μL of the mixture of isooctane and isopropanol (80:20, v/v) was added to precipitate protein. After vortexed for 1 min and centrifuged at 6000 g for 10 min, the supernatant was separated and evaporated under the stream of nitrogen at 37 °C. The residue was dissolved in 50 μL mobile phase and an aliquot of 20 μL was injected into the HPLC for analysis.
Statistical analysis
All results were reported as the mean ± standard deviation (SD) unless otherwise indicated. Differences between two groups were analyzed using Student’s t-test. Differences between multiple groups were analyzed using ANOVA. A value of p < 0.05 was considered statistically significant.
Results
Characterization of micelles
Probucol-loaded micelles were prepared by film formation method. The dynamic light scattering measurements showed that the mean diameter of micelles was 20.7 ± 2.3 nm and the particle size distribution was relatively narrow (). The encapsulation efficiency and drug loading of mPEG-DSPE micelles were 94.69 ± 1.94% and 4.27 ± 0.15%, respectively. Few probucol leaking from micelles was observed during 8 h at room temperature.
Uptake and transport in the Caco-2 cell model
The intestinal absorption of probucol mPEG-DSPE micelles in vitro was investigated in the Caco-2 cell model. The experimental results showed that the uptake and transport of probucol were significantly enhanced by the preparation of mPEG-DSPE micelles, which were 2.4-fold and 22-fold, respectively of those of drug suspension (p < 0.05) ( and ). Physical mixture with mPEG-DSPE also could improve the uptake and transport of probucol in the Caco-2 cell (p < 0.05). But the micellar solution had the more significant enhancement effect than physical mixture, especially on the transport (p < 0.05).
Mesenteric lymphatic transport in rats
The HPLC method for probucol analysis was validated. Linearity of the standard curves was demonstrated at the concentration range studied, and endogenous components had no interference in the chromatograms. The anesthetic and conscious cannulated rat models were established successfully. The profiles of the extent of intestinal lymphatic uptake versus time of probucol after oral administration of micelles, mixtures, suspensions at a single dose of 6 mg/kg are shown in and , and the respective cumulative amount secreted in lymph is given in . There was no significant difference between the suspension group and mixture group in vivo. It was different from the result in cell experiment. The cumulative drug amount of micelles in lymph was significantly increased following the time prolonging and the absolute cumulative drug amount was much higher than that of the suspension group and mixture group (p < 0.05) in both the anesthetic and conscious rat models during 8 h. It showed that mPEG-DSPE micelles could enhance the lymphatic transport of the drug approximately 19-fold and 3-fold higher than that of suspension in the anesthetic group and conscious group, respectively. Compared with the mixture group, the micelles also obtained much higher lymph accumulative amount, especially in the anesthetic rat model. It certified the ability of mPEG-DSPE micelles in targeting probucol to intestinal lymphatic region. Meanwhile, a significant difference in lymphatic absorption between the anesthetic model and conscious model was observed. Till 8 h, the cumulative drug amount transported in lymph in the conscious rat model was much higher than that in the anesthetic rat model, especially for the probucol suspension (p < 0.01). The difference between the suspension and the micelle in the conscious model was much smaller than that in the anesthetic model.
Figure 4. The mean cumulative drug amount in lymph versus time profiles of probucol in the anesthetic rat model. The data represent the mean ± SD, n = 5.
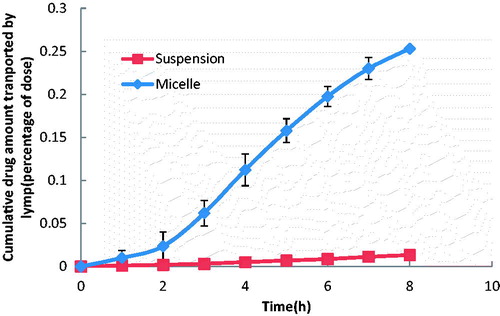
Figure 5. The mean cumulative drug amount in lymph versus time profiles of probucol in the conscious rat model. The data represent the mean ± SD, n = 5.
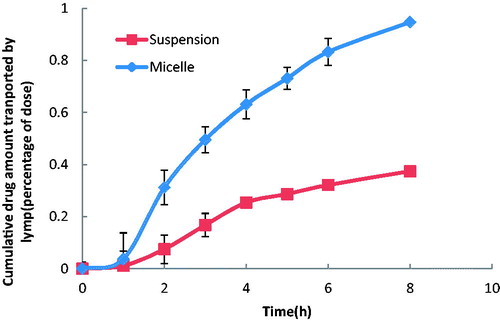
Table 1. Cumulative percentage dose of probucol transported in lymph in 8 h.
Discussion
The potential to use a Caco-2 cell model to investigate lipid absorption mechanisms at the cellular level has been reviewed by O’Driscoll (Lane et al., Citation1998). Several studies have indicated that many of the biochemical and metabolic features of lipid processing in vivo exist in the Caco-2 cells, such as uptake of fatty acid followed by esterification to form triglycerides, synthesis and secretion same apoproteins as small intestinal enterocyte which is essential for normal assembly, secretion and catabolism of intestinal lipoproteins, production of triglycerdein-rich lipoproteins in similar size and density with human intestine in the presence of fatty acid, and so on (Trotter et al., Citation1996; Field & Mathur,Citation1995). These abilities found in the Caco-2 cells make it a useful model to investigate the lymphatic transport in vitro. Therefore, the evaluation of the effect in vitro on drug lymphatic transport was conducted on the Caco-2 cell model in this study.
It is generally believed that lipid-based vehicles enhance intestinal lymphatic drug transport via two main ways: stimulation of chylomicron secretion and association of the drug with the triglyceride core of the lipoprotein. Effect on stimulation of chylomicron secretion is consanguineous related with the vehicle materials’ character. It was supposed that the effect could be attributed to mPEG-DSPE if exist in our study. A series of correlative experiments were carried out to verify our deduction. The association of drug and chylomicron can occur both intracellular and extracellular. An increased drug uptake and transport by the Caco-2 cells could result in a higher drug concentration in cell matrix and intercellular space, facilitating the association and enhancing drug lymphatic transport. Significantly increased drug uptake and transport in drug-loaded micelle group were observed (2.4-fold in uptake and 22-fold in transport compared with the pure drug group, 1.2-fold in uptake and 6-fold in transport compared with mixture of drug and excipient) in this study. Obviously, encapsulation of probucol in mPEG-DSPE micelle increased the hydrophilic interaction and facilitated the membrane penetration, and then enhanced drug uptake and transport.
A much more significant facilitation in transport than that in uptake suggested that the micelle could enhance drug permeation through the cell monolayer by not only transcellular pathway, but also paracellular pathway.
Advanced in vivo research in rats was carried out to verify the result obtained from the in vitro study and complement the evaluation of effect on enhancing drug lymphatic transport ulteriorly. In vivo study was conducted both in the unconscious and conscious lymph duct cannulated rat models. A 19-fold of enhancement on drug lymphatic transport in the micelle group versus the suspension group during 8 h was observed in the anesthetic group, which verified the result obtained from the in vitro study. A same trend was obtained in the conscious group. All of these results indicated that the micellar formulation with mPEG-DSPE could significantly enhance lymphatic transport of probucol and had the potential to be used for the delivery of hydrophobic compounds, such as probucol to the lymphatic system by oral administration.
The lymph duct cannulated animal model can be manipulated in rat, mouse and dog, which helps to get the firsthand information for evaluation of drug lymphatic transport. Rat model is the most widely used and can be divided into the unconscious, the conscious restrained and the conscious un-restrained models. The conscious rat model is supposed to best represent the in vivo situation in terms of both lack of anesthetic effect and the ability to administer oral drug formulations. But the surgery procedure is much more complicated and gets more risks comparing with the unconscious model. In this study, it was found that there are some critical limitations in the unconscious model. The first is the short sampling time. After anesthetization, the rats could maintain normal physiological state for only about 8 h. The lymph was harvested no longer than 8 h, which could not satisfy the integral evaluation of drug lymphatic transport. A longer sampling period of 12 h even 24 h was needed to get it. Secondly, blood sample could not be collected at the same time because the harvest of blood every 30 min would speed the dehydration of the anesthesia rat. It would lead to the reduction of collected lymph amount or even the death of rat during the experiment. So, the effect of micellar system on oral absorption could not be comprehensively evaluated due to the lack of data of plasma drug concentration. Thirdly, the data in this study showed that the cumulative drug amount transported in lymph in the conscious model was much higher than that in the anesthetic model. Both the digestion and absorption of lipid-soluble drug in small intestine were based on the mixing and emulsification process in stomach. In the anesthetic model, the formulations were administered intraduodenally and circumvented the inherent emulsifying action of the stomach and the potential effect of lipids on gastric emptying, which directly affected digestion of formulation in small intestine and brought out a lower absorption rate. The improvement of solubility of probucol by active intestinal peristalsis in the conscious model may be another major reason. The rate of absorption of poorly water soluble drugs was generally slower in the unconscious model than in the conscious model. Furthermore, lymph flow rate was influenced by anesthesia. In the anaesthetized rat, it ranges between 0.1 and 0.6 mL/h and in the conscious rat it may increase up to 1–3 mL/h. It was caused by decrease in motility and function of the gastrointestinal tract, altered capillary permeability and reduced interstitial fluid formation and venous return in the anaesthetized rate. Those led to a more significant difference in lymphatic transport of probucol suspension between the conscious and unconscious models. In addition, physical mixture of probucol and mPEG-DSPE had different influence on the uptake and transport in the Caco-2 cell and lymph transport in rats. The mixture could significantly improve the uptake and transport of probucol in the Caco-2 cell, but no significant effect in lymph accumulation in vivo. It was caused by the different state of probucol in the in vitro and in vivo study. Probucol was a highly insoluble drug. In the cell experiment, the drug should be dissolved before dosing. So 1% DMSO was used to prepare the probucol solution and the mix solution. For the mixture group, the mPEG-DSPE could improve the drug molecule uptake and transport. So the facilitation effect was obtained in the Caco-2 cell. But in the in vivo study, the mixture group was administrated in suspension. Probucol was only dispersed in the media. The dissolution was the rate-limiting step for drug lymphatic transport, which caused that only a light enhancement effect of mPEG-DSPE in animal study.
Conclusion
In this study, it was showed that mPEG-DSPE micelles could load probucol with high efficiency. The in vitro evaluation in the Caco-2 cell model indicated that the micellar formulation could significantly enhance the uptake and transport of probucol. The study in the unconscious and conscious lymph duct cannulated rat models further verified a significant improvement of lymphatic transport of mPEG-DSPE micelles. These results suggested that mPEG-DSPE micellar formulation could provide a useful alternative dosage form for improving the lymphatic transport of hydrophobic compounds. The significant difference in cumulative percentage dose of probucol transported in lymph between the unconscious and conscious models indicated that the conscious lymph duct cannulated model was the more appropriate model for the evaluation of lymphatic transport, although the same trend was observed from the two models.
Declaration of interest
The authors report no conflicts of interest. The authors alone are responsible for the content and writing of this work.
This work was financially supported by F Hoffmann-La Roche Ltd. (Basel, Switzerland), National Natural Science Foundation of China (81001642) and National Science and Technology Major Project (2012ZX09304004).
References
- Aji Alex MR, Chacko AJ, Jose S, Souto EB. (2011). Lopinavir loaded solid lipid nanoparticles (SLN) for intestinal lymphatic targeting. Eur J Pharm Sci 42:11–18
- Ali Khan A, Mudassir J, Mohtar N, Darwis Y. (2013). Advanced drug delivery to the lymphatic system: lipid-based nanoformulations. Int J Nanomedicine 8:2733–44
- Caliph SM, Charman WN, Porter CJ. (2000). Effect of short-, medium-, and long-chain fatty acid based vehicles on the absolute oral bioavailability and intestinal lymphatic transport of halofantrine and assessment of mass balance in lymph-cannulated and non-cannulated rats. J Pharm Sci 89:1073–84
- Charman WN, Porter CJH. (1996). Lipophilic prodrugs designed for intestinal lymphatic transport. Adv Drug Deliv Rev 19:149–69
- Dahmani FZ, Yang H, Zhou J, et al. (2012). Enhanced oral bioavailability of paclitaxel in pluronic/LHR mixed polymeric micelles: preparation, in vitro and in vivo evaluation. Eur J Pharm Sci 47:179–89
- Edwards GA, Porter CJ, Caliph SM, et al. (2001). Animal models for the study of intestinal lymphatic drug transport. Adv Drug Deliv Rev 50:45–60
- Faisal W, O'Driscoll CM, Griffin BT. (2010). Bioavailability of lycopene in the rat: the role of intestinal lymphatic transport. J Pharm Pharmacol 62:323–31
- Fan Z, Wu J, Fang X, Sha X. (2013). A new function of Vitamin E-TPGS in the intestinal lymphatic transport of lipophilic drugs: enhancing the secretion of chylomicrons. Int J Pharm 445:141–7
- Field FJ, Mathur SN. (1995). Intestinal lipoprotein synthesis and secretion. Prog Lipid Res 34:185–98
- Gao Y, Wang Y, Ma Y, et al. (2009). Formulation optimization and in situ absorption in rat intestinal tract of quercetin-loaded microemulsion. Colloids Surf B Biointerfaces 71:306–14
- Griffin BT, O'Driscoll CM. (2006). A comparison of intestinal lymphatic transport and systemic bioavailability of saquinavir from three lipid-based formulations in the anaesthetised rat model. J Pharm Pharmacol 58:917–25
- Han LM, Guo J, Zhang LJ, et al. (2006). Pharmacokinetics and biodistribution of polymeric micelles of paclitaxel with Pluronic P123. Acta Pharmacol Sin 27:747–53
- Lane ME, Meaney CM, Gubbins R, et al. (1998). Correlation between permeability co-efficients in a perfused rat model and the CaCo-2 cell model. Eur J Pharm Sci 6:S24
- Le Dévédec F, Strandman S, Hildgen P, et al. (2013). PEGylated bile acids for use in drug delivery systems: enhanced solubility and bioavailability of itraconazole. Mol Pharm 10:3057–66
- Li GP, Yin L, Liu T, et al. (2009). Role of probucol in preventing contrast-induced acute kidney injury after coronary interventional procedure. Am J Cardiol 103:512–14
- Lu Y, Park K. (2013). Polymeric micelles and alternative nanonized delivery vehicles for poorly soluble drugs. Int J Pharm 453:198–214
- Nam YS, Kang HS, Park JY, et al. (2003). New micelle-like polymer aggregates made from PEI-PLGA diblock copolymers: micellar characteristics and cellular uptake. Biomaterials 24:2053–9
- Nanjwade BK, Patel DJ, Udhani RA, Manvi FV. (2011). Functions of lipids for enhancement of oral bioavailability of poorly water-soluble drugs. Sci Pharm 79:705–27
- Nishiyama N, Kataoka K. (2006). Nanostructured devices based on block copolymer assemblies for drug delivery: designing structures for enhanced drug function. Adv Polym Sci 193: 67–101
- O’Driscoll CM. (2002). Lipid-based formulations for intestinal lymphatic delivery. Eur J Pharm Sci 15:405–15
- Shahnaz G, Hartl M, Barthelmes J, et al. (2011). Uptake of phenothiazines by the harvested chylomicrons ex vivo model: influence of self-nanoemulsifying formulation design. Eur J Pharm Biopharm 79:171–80
- Trotter PJ, Ho SY, Storch J. (1996). Fatty acid uptake by Caco-2 human intestinal cells. J Lipid Res 37:336–46
- Yáñez JA, Wang SW, Knemeyer IW, et al. (2011). Intestinal lymphatic transport for drug delivery. Adv Drug Deliv Rev 63:923–42
- Yu C, He B, Xiong MH, et al. (2013). The effect of hydrophilic and hydrophobic structure of amphiphilic polymeric micelles on their transport in epithelial MDCK cells. Biomaterials 34:6284–98