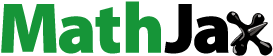
Abstract
Context: Paclitaxel (PTX) and carboplatin (CBP) are widely used for the combined chemotherapy of non-small cell lung cancer (NSCLC). However, the development of multidrug resistance of cancer cells, as well as systemic toxic side effects resulting from nonspecific localization of anticancer drugs to non-tumor areas are major obstacles to the success of chemotherapy in treating cancers.
Objective: This study aimed to engineer a prodrug-based nano-drug delivery system for co-encapsulate hydrophilic (CBP) and hydrophobic anti-tumor drugs (PTX). This system was expected to resolve the multidrug resistance cause by single drug, and the dual-drug-loaded liposome was also planned to specifically target the cancer cells without obvious influence on normal cells and tissues.
Methods: In this paper, PLGA-PEG-CBP was synthesized by the conjugation between the carboxylic group of PLGA-PEG-COOH and the amino group of CBP. Then, self-assembled nanoparticles for combination delivery of PTX and PLGA-PEG-CBP (PTX/CBP NPs) were prepared by solvent displacement technique. The in vitro and in vivo anti-tumor efficacy was assessed in NCL-H460 human non-small cell lung carcinoma cell line.
Results: PTX/CBP NPs achieved the highest cytotoxic effect among all formulations in vitro, as compared with single drug delivery NPs. In vivo investigation on NSCLC animal models showed that co-delivery of PTX and CBP possessed high tumor-targeting capacity and strong anti-tumor activity.
Conclusions: The PTX/CBP NPs constructed in this research offers an effective strategy for targeted combinational lung cancer therapy.
Introduction
Lung cancer is the leading cause of cancer deaths over the world, with almost 1.6 million deaths in 2012 alone (Powell et al., Citation2014). Non-small cell lung cancer (NSCLC) accounts for 80–85% of all lung cancers and surgical therapy is performed with curative intent in early stage NSCLC. However, 30–70% of patients with surgically resected NSCLC develop recurrence, and early metastatic spread limits long-term overall survival (Chang et al., Citation2014). Therefore, chemotherapy still plays an important role in NSCLC.
The first line chemotherapy treatment protocol for NSCLC is the following: two-drug combinations of cisplatin or carboplatin (CBP) plus a “third generation” drug (gemcitabine, vinorelbine, paclitaxel (PTX) and docetaxel) (Kelly et al., Citation2001; Scagliotti et al., Citation2002; Schiller et al., Citation2002; de Marinis et al., Citation2011). It has been reported in several cases in clinics that combination chemotherapy effectively generate synergistic anticancer effects, reduce individual drug-related toxicity, suppress multi-drug resistance through different mechanisms of action and reduce the dose of each agent (Gianni et al., Citation1995; Vasey et al., Citation1999). Among them, taxanes remain a key component in the first-line standard of care for advanced NSCLC and NSCLC subtypes. In 2012, a novel taxane, nab-PTX (Abraxane), was approved by FDA, in combination with CBP, for the first-line treatment of locally advanced or metastatic NSCLC. Although nab-PTX has more anti-tumor activity and tolerability compared with solvent-based PTX, free CBP and no delivery system for CBP has been employed in the regimen (Satouchi et al., Citation2013; Socinski, Citation2014). Therefore, it is urgent to design nanocarriers platform to co-encapsulate PTX and CBP to increase therapeutic efficacy and decrease toxic effect.
Various nanotechnology-based drug delivery systems (DDS) have been developed to improve combinational chemotherapeutic efficiency, such as polymeric nanoparticles (NPs) (Duan et al., Citation2012), dendrimers (Jain et al., Citation2014), micelles (Gill et al., Citation2012) and liposomes (Dai et al., Citation2012). Most nanotechnology-based drug combinations comprise drug compounds with similar water solubility, such as liposomes for hydrophilic drugs and polymeric NPs for hydrophobic drugs (Shin et al., Citation2011). The difficulty in co-encapsulating hydrophilic and hydrophobic drugs leads to a major obstacle on the possible therapy combinations. Chemical modifications (prodrug) and polymeric NPs, prodrug-based nano-drug delivery system (P-N-DDS), have been published mostly as a platform to overcome this difficulty (Shiah et al., Citation2001; Kolishetti et al., Citation2010; Hu & Zhang, Citation2012; Xiao et al., Citation2012; Butler & Sadler, Citation2013; Oberoi et al., Citation2013). PLGA-PEG is one of the most common hydrophobic biodegradable polymers for NPs and prodrug nano-DDS (Kolishetti et al., Citation2010; Dhar et al., Citation2011; Xu et al., Citation2013; Zhang et al., Citation2014). Based on the platform-mentioned above, poly(D,L-lactic acid-co-glycolic acid)-block-poly(ethyleneglycol) copolymer-CBP (PLGA-PEG-CBP) was synthesized. PTX, the hydrophobic drug, was encapsulated in the core of PLGA-PEG-CBP NPs.
PTX, derived from the dark of the Pacific yew tree Taxus brevifolia, is a new class of microtubule anti-proliferative agent. To solve its hydrophobic problem, two kinds of technology, solubilization and NPs have been applied; and marketed products, Taxol® (Bristol-Myers Squibb Company, Princeton, NJ) and Abraxane® (Celgene Corporation, Summit, NJ), have been approved by the FDA in 2005 and 2012, respectively. Based on a large number of clinical researches, combination therapy of Abraxane® and CBP has been approved to treat NSCLC.
CBP is one of the most important anti-tumor drugs among the second generation of platinum metal-based drugs, and widely used in clinics. Its toxicities still reduce patient’s tolerance and severely limit the patient’s quality of life. Hematological side effects are more common with CBP compared with cisplatin and oxaliplatin. CBP exerts potent myelosuppression resulting in thrombocytopenia and neutropenia (McKeage, Citation1995; Oberoi et al., Citation2013). Therefore, it is necessary to explore alternate strategies such as the use of targeted platinum complexes or nanocarriers-based delivery approaches. Platinum drug delivery using nanocarriers contain liposomes (Boulikas, Citation2004; Stathopoulos & Boulikas, Citation2012), lipid-coated nanocapsules (Burger et al., Citation2002), polymer-platinum conjugates (Rice et al., Citation2006; Gill et al., Citation2012), nanotubes (Feazell et al., Citation2007) and micelles (Oberoi et al., Citation2011). Almost all of platinum nanocarriers DDS are involved with cisplatin. It is needed to explore this field for CBP to enhance therapeutic efficacy and decrease toxicity.
In this paper, PLGA-PEG-CBP was synthesized by the conjugation between the carboxylic group of PLGA-PEG-COOH and the amino group of CBP. Then, self-assembled NPs for combination delivery of PTX and PLGA-PEG-CBP were prepared by the solvent displacement technique. The in vitro and in vivo anti-tumor efficacy was assessed in NCL-H460 human non-small cell lung carcinoma cell line. This system was expected to achieve stable and controlled release DDS of CBP prodrug and PTX separately, to enhance synergistic anticancer effects, and decrease toxic effect.
Materials and methods
Materials
PTX was provided by Hisun Pharmaceutical Co Ltd. (Zhejiang, China). CBP was provided by Qilu Pharmaceutical Co Ltd. (Shandong, China). Poly (DL-lactide-co-glycolide)-b-Poly (ethylene glycol)-COOH (PLGA17k-PEG3k-COOH) was obtained from Shanghai ZZBio Co., Ltd (Shanghai, China). NCL-H460 human non-small cell lung carcinoma cells were obtained from the American type culture collection (Manassas, VA). All other chemicals were of analytical grade or higher.
Animals
BALB/c nude mice (4–6 weeks old, 18–22 g weight) were purchased from the Beijing Fuzhong Technology Development Co., Ltd (Beijing, China). All animal experiments complied with the Animal Management Rules of the Ministry of Health of the People’s Republic of China.
Synthesis and characteristics of PLGA-PEG-CBP
PLGA-PEG-CBP was synthesized by two steps reaction. The synthesis scheme is depicted in .
First, PLGA-PEG-NHS was synthesized. Two grams PLGA-PEG-COOH was dissolved in DMSO. Then N-hydroxysuccinimide (NHS) and 1-ethyl-3-(3-dimethylaminopropyl)-carbodiimide (EDC) were added to react at room temperature for 48 h. PLGA-PEG-NHS was obtained by filtered and dried under vacuum.
Second, PLGA-PEG-CBP was obtained by the following method: CBP and PLGA-PEG-NHS (2:1, molar ration) were dissolved in DMSO separately, and mixed to synthesize for 48 h at room temperature. Then, the mixture was purified by dialysis with a 12 000–14 000 MV cut-off membrane for another 48 h to remove excess CBP and other impurities. The final product PLGA-PEG-CBP was obtained by lyophilization.
1H NMR spectra of PLGA-PEG-CBP were analyzed on a NMR Spectrometer (300 Hz, Bruker, Switzerland). δ (ppm): 1.96–2.42 (s, –CH2–); 2.02 (s, –NH2); 2.66 (s, –CO–CH2–), 3.54–3.65 (d, –O–CH2–CH2–O–); 3.03–3.85 (t, –CH–); 5.04 (t, –O–CO–CH2–O–); 5.23 (t, –OH); 8.19 (s, –NH–CO–); 8.12 (s, –C–NH–CO–). The structures of –NH–CO– and –O–CO–CH2– peaks confirmed the CBP molecules were successfully conjugated onto the PLGA-PEG. The production yield was 72%.
Preparation of drug-loaded NPs
The PTX-loaded PLGA-PEG-CBP NPs (PTX/CBP NPs) were prepared by the solvent diffusion method. Briefly, a solution of PLGA-PEG-CBP (16.5 mg/mL) and PTX (0.0625 mg/mL) in DMSO was prepared. The solution (4 mL) was allowed to stir for 3 h at room temperature. Then, the solution (4 mL) was added to distilled water (16 mL) drop by drop under moderate magnetic stirring for 8 h. Finally, the solution was dialyzed (MWCO 12 000–14 000) against distilled water for 24 h to obtain PTX-CBP NPs.
PTX-loaded PLGA-PEG NPs without CBP (PTX NPs), and PLGA-PEG-CBP NPs without PTX (CBP NPs) were prepared by the same method-mentioned above, for the contrast.
Characterization of drug-loaded NPs
The mean diameter, polydispersity index (PDI), and zeta potential of PTX NPs, CBP NPs, and PTX/CBP NPs were analyzed using photon correlation spectroscopy with a Zetasizer 3000 (Malvern Instruments, Malvern, England).
The PTX encapsulation efficiency (EE) of PTX NPs and PTX/CBP NPs was determined by a subtraction method. Briefly, drug-loaded NPs were dispersed in phosphate buffer solution (2.0% Tween 80 in PBS, pH 7.4) and vortexed for 3 min to dissolve the free PTX. Then, the dispersion was centrifuged for 0.5 h at 10 000 rpm to separate the free drug from NPs. After the centrifugation, PTX content in the supernatant was measured by HPLC (Waters2695, Milford, MA) (Mittal et al., Citation2007). Chromatographic separations were carried out using the Intertsil® ODS-3V (GL Sciences Inc, Tokyo, Japan) (25 cm × 4.6 mm, 5 μm). Mobile phase was consisted of a mixture of acetonitrile and water (50:50, v/v). Flow rate was kept at 1.0 mL/min and the system was maintained at 35 °C, the detection was carried out at λ = 227 nm. Injection volume was 20 μL.
(1)
(1)
where Wtotal-PTX is the weight of PTX added when preparing PTX NPs or PTX/CBP NPs; Wfree-PTX is the weight of the PTX measured in the supernatant of PTX NPs or PTX/CBP NPs.
In vitro drug release
In vitro release studies from NPs were assessed in release medium (0.1% Tween 80 in phosphate-buffered saline, PBS pH 7.4) by the dialysis method. Briefly, 5 mL of PTX NPs, CBP NPs and PTX-CBP NPs were placed in the dialysis bag separately. Then, the bag was incubated with 50 mL of release medium at 37 °C. One milliliter of medium was collected at predetermined time points and replaced with 50 mL of fresh medium. The concentrations of released PTX were determined by the HPLC method-mentioned above. The concentrations of released CBP were determined by atomic absorption spectroscopy (AAS, TAS-968) from Beijing Purkinje General Instrument Co., Ltd (Beijing, China), with the cathode platinum (Pt) lamp (Amorusi et al., Citation1994). The detection was carried out at λ = 265.9 nm. Flow rate of air and acetylene was kept at 8 L/min and 1500 mL/min.
In vitro cytotoxicity assays
The ability of various treatments to inhibit cell proliferation was evaluated by NCL-H460 cells with MTT assay (Ulukaya et al., Citation2008; Wang et al., Citation2013). Cells cultured in RPMI 1640 medium supplemented with 10% fetal bovine serum and antibiotics with 5% CO2 at 37 °C were seeded onto 96-well plates at 1 × 104 cells/well and incubated for 24 h. Then, cells were incubated with 0.9% saline (the control group), PTX solution, CBP solution, PTX NPs, CBP NPs and PTX/CBP NPs at various concentrations for 48 h at 37 °C and 5% CO2 atmosphere, respectively. Culture medium was used as the blank group. Then, MTT solution (5 mg/mL) was added to each well and cells were incubated for 4 h. MTT-containing medium was discarded and replaced with 200 μL DMSO to dissolve the MTT formazan crystals. The optical density of formazan product was measured using a microplate reader (Model 680, BIO-RAD, USA) at 570 nm. The relative cell viability was calculated by the below equation
The median inhibitory concentration (IC50) values of drug-loaded NPs were calculated.
In vivo anti-tumor efficacy
The combination anti-tumor efficacy of PTX and CBP was investigated in NCL-H460 tumor-bearing BALB/c nude mice models, which were developed by injection of cells in the right armpit of BALB/c mice. When tumor volume reached 90–100 mm3, transplanted mice were randomly divided into 6 groups (10 per group) separately. Formulations for seven groups were as follows: (1) 0.9% saline, (2) PTX solution (2 mg/kg), (3) CBP solution (4 mg/kg), (4) PTX NPs (2 mg/kg), (5) CBP NPs (4 mg/kg) and (6) PTX/CBP NPs (1 mg PTX/kg, 2 mg CBP/kg). The mice of each group were given the above formulations by tail vein injection once every two days. Fourteen days later, all the mice were sacrificed by cervical dislocation and the tumor tissue samples were taken out (Belani et al., Citation2008).
Tumor volume of each mouse was measured with a digital caliper every two days, and was calculated according to the below equation
The anti-tumor efficacy of each formulation was evaluated by tumor inhibition rate (TIR), which was calculated using the following formula:
where C and T represent the tumor weight of the control and treated groups, respectively.
Statistical analysis
All studies were repeated three times and all measurements were carried out in triplicate. Results were reported as means ± SD (SD = standard deviation). Statistical significance was tested by two-tailed Student’s t-test or one-way analysis of variance. Differences between experimental groups were considered significant when the p value was less than 0.05 (p < 0.05).
Results and discussion
Characterization of drug-loaded NPs
PLGA-PEG-CBP is an amphiphilic compound. CBP is the hydrophilic heads and while PLGA is the hydrophobic tails. PTX is a poor soluble drug. According to this characteristic, we constructed self-assemble NPs with PTX and CBP as the inner core, and PEG-CBP as the shell. summarized the mean diameter, PDI, zeta potential, and EEPTX of PTX NPs, CBP NPs, and PTX/CBP NPs.
Table 1. Characterization of drug-loaded NPs.
The size of PTX/CBP NPs was 125 nm, larger than PTX NPs (88 nm). This may because the CBP on the surface enlarged the size of the particles. Particle size is a key influence factor of the carriers (Rahman et al., Citation2013). It has impacts on the in vitro and in vivo efficiency of the NPs, including decreased uptake by the liver, prolonged blood circulation time and improved bioavailability (Fang et al., Citation2011). The zeta potential of PTX-BCL NPs was −5.1. The EEPTX in both PTX/CBP NPs and PTX NPs were around 85%. This could be the evidence that the conjugation of CBP did not have a passive effect on the encapsulation of PTX. The high EE achieved could offer advantages in the drug delivery in vitro and in vivo.
In vitro release studies
In vitro release studies from NPs were assessed by the dialysis method. The in vitro PTX and CBP release profiles of different NLCs formulations are depicted in and . PTX/CBP NPs, PTX NPs and CBP NPs showed the sustained-release behavior of both PTX and CBP. For PTX release, nearly complete accumulated releases were achieved at 72 h for both PTX/CBP NPs and PTX NPs. CBP release was slower, completely releases were observed at 96 h for PTX/CBP NPs and CBP NPs. The release profiles of three NPs for two drugs can be summarized as follows: (1) the dual drugs loaded NPs showed almost the same release behavior compared with the single drug-loaded NPs; (2) sustained-release phenomenon could be explained by the property of PLGA, and also by the degradation process of the PLGA-PEG-CBP prodrug; (3) the sustained-release behavior may help with the anti-tumor progress, bring about continuous anti-tumor effect.
In vitro cytotoxicity assays
The ability of various treatments to inhibit cell proliferation was evaluated by NCL-H460 cells with MTT assay. showed the IC50 values of drug-loaded NPs and drug solutions. The IC50 values of PTX were 3.78, 1.12, and 0.38 in PTX solution, PTX NPs and PTX/CBP NPs, respectively. The PTX IC50 value of PTX NPs was 3.4 fold than PTX solution, and PTX/CBP NPs was 3 fold than PTX NPs. The CBP IC50 value of PTX/CBP NPs was 2.3 and 6.4 fold than CBP NPs and CBP solution, respectively, accounting for the highest anti-tumor activity. These results could be the evidence of the better combination anti-tumor effect of the double drugs loaded NPs than the single drug NPs, also could conclude that the NPs formulas preformed better than their free drug solution counterparts.
Table 2. IC50 of drug-loaded NPs and drug solutions.
In vivo anticancer efficacy
The combination anti-tumor efficacy of PTX and CBP was investigated in NCL-H460 tumor-bearing BALB/c nude mice models. The tumor growth inhibitions over different groups were monitored for 14 days. illustrated that: (1) tumor growth was significantly inhibited by NPs formulations than drug solution groups (p < 0.05); (2) the combination of PTX and CBP NPs was more effective than single drug NPs (p < 0.05); (3) the TIR of PTX/CBP NPs, CBP NPs and PTX NPs were 79%, 45%, and 51%, separately. PTX/CBP NPs exhibited the best tumor inhibition efficiency than other formulations.
Conclusions
In the present work, PLGA-PEG-CBP prodrug was synthesized. PTX and CBP co-encapsulated NPs were constructed. PTX/CBP NPs were effective in lung cancer cells, showing strong cytotoxicity of tumor cells in vitro and significantly tumor growth inhibition ability of in vivo. The synergistic anti-tumor efficacy was assessed in NCL-H460 lung cancer cells and mice-bearing lung cancer cells model. PTX/CBP NPs could function as promising active-targeting nanomedicine, offers a promising combined tumor therapy strategy for the treatment of lung cancer.
Declaration of interest
The authors report no conflicts of interest. The authors alone are responsible for the content and writing of this article.
References
- Amorusi P, Lessard D, Bansal SK, et al. (1994). Analysis of enloplatin by liquid chromatography and of platinum by atomic absorption spectrometry in various biological fluids. J Pharm Biomed Anal 12:1023–33
- Belani CP, Ramalingam S, Perry MC, et al. (2008). Randomized, phase III study of weekly paclitaxel in combination with carboplatin versus standard every-3-weeks administration of carboplatin and paclitaxel for patients with previously untreated advanced non-small-cell lung cancer. J Clin Oncol 26:468–73
- Boulikas T. (2004). Low toxicity and anticancer activity of a novel liposomal cisplatin (Lipoplatin) in mouse xenografts. Oncol Rep 12:3–12
- Burger KN, Staffhorst RW, de Vijlder HC, et al. (2002). Nanocapsules: lipid-coated aggregates of cisplatin with high cytotoxicity. Nat Med 8:81–4
- Butler JS, Sadler PJ. (2013). Targeted delivery of platinum-based anticancer complexes. Curr Opin Chem Bio 17:175–88
- Chang WJ, Sun JM, Lee JY, et al. (2014). A retrospective comparison of adjuvant chemotherapeutic regimens for non-small cell lung cancer (NSCLC): paclitaxel plus carboplatin versus vinorelbine plus cisplatin. Lung Cancer 84:51–5
- Dai W, Jin W, Zhang J, et al. (2012). Spatiotemporally controlled co-delivery of anti-vasculature agent and cytotoxic drug by octreotide-modified stealth liposomes. Pharm Res 29:2902–11
- de Marinis F, Rossi A, Di Maio M, et al. (2011). Italian association of thoracic oncology. Treatment of advanced non-small-cell lung cancer: Italian Association of Thoracic Oncology (AIOT) clinical practice guidelines. Lung Cancer 73:1–10
- Dhar S, Kolishetti N, Lippard SJ, et al. (2011). Targeted delivery of a cisplatin prodrug for safer and more effective prostate cancer therapy in vivo. Proc Natl Acad Sci USA 108:1850–5
- Duan J, Mansour HM, Zhang Y, et al. (2012). Reversion of multidrug resistance by co-encapsulation of doxorubicin and curcumin in chitosan/poly(butyl cyanoacrylate) nanoparticles. Int J Pharm 426:193–201
- Fang YP, Lin YK, Su YH, et al. (2011). Tryptanthrin-loaded nanoparticles for delivery into cultured human breast cancer cells, MCF7: the effects of solid lipid/liquid lipid ratios in the inner core. Chem Pharm Bull (Tokyo) 59:266–71
- Feazell RP, Nakayama-Ratchford N, Dai H, et al. (2007). Soluble single-walled carbon nanotubes as longboat delivery systems for platinum(IV) anticancer drug design. J Am Chem Soc 129:8438–9
- Gianni L, Munzone E, Capri G, et al. (1995). Paclitaxel by 3-hour infusion in combination with bolus doxorubicin in women with untreated metastatic breast cancer: high antitumor efficacy and cardiac effects in a dose-finding and sequence-finding study. J Clin Oncol 13:2688–99
- Gill KK, Kaddoumi A, Nazzal S. (2012). Mixed micelles of PEG(2000)-DSPE and vitamin-E TPGS for concurrent delivery of paclitaxel and parthenolide: enhanced chemosenstization and antitumor efficacy against non-small cell lung cancer (NSCLC) cell lines. Eur J Pharm Sci 46:64–71
- Hu CM, Zhang L. (2012). Nanoparticle-based combination therapy toward overcoming drug resistance in cancer. Biochem Pharmacol 83:1104–11
- Jain K, Gupta U, Jain NK. (2014). Dendronized nanoconjugates of lysine and folate for treatment of cancer. Eur J Pharm Biopharm 87:500–9
- Kelly K, Crowley J, Bunn PA Jr, et al. (2001). Randomized phase III trial of paclitaxel plus carboplatin versus vinorelbine plus cisplatin in the treatment of patients with advanced non–small-cell lung cancer: a Southwest Oncology Group trial. J Clin Oncol 19:3210–18
- Kolishetti N, Dhar S, Valencia PM, et al. (2010). Engineering of self-assembled nanoparticle platform for precisely controlled combination drug therapy. Proc Natl Acad Sci USA 107:17939–44
- McKeage MJ. (1995). Comparative adverse effect profiles of platinum drugs. Drug Saf 13:228–44
- Mittal A, Chitkara D, Kumar N. (2007). HPLC method for the determination of carboplatin and paclitaxel with cremophorEL in an amphiphilic polymer matrix. J Chromatogr B Analyt Technol Biomed Life Sci 855:211–19
- Oberoi HS, Laquer FC, Marky LA, et al. (2011). Core cross-linked block ionomer micelles as pH-responsive carriers for cis-diamminedichloroplatinum(II). J Control Release 153:64–72
- Oberoi HS, Nukolova NV, Kabanov AV, et al. (2013). Nanocarriers for delivery of platinum anticancer drugs. Adv Drug Deliv Rev 65:1667–85
- Powell HA, Tata LJ, Baldwin DR, et al. (2014). Treatment decisions and survival for people with small-cell lung cancer. Br J Cancer 110:908–15
- Rahman HS, Rasedee A, How CW, et al. (2013). Zerumbone-loaded nanostructured lipid carriers: preparation, characterization, and antileukemic effect. Int J Nanomed 8:2769–81
- Rice JR, Gerberich JL, Nowotnik DP, et al. (2006). Preclinical efficacy and pharmacokinetics of AP5346, a novel diaminocyclohexane-platinum tumor-targeting drug delivery system. Clin Cancer Res 12:2248–54
- Satouchi M, Okamoto I, Sakai H, et al. (2013). Efficacy and safety of weekly nab-paclitaxel plus carboplatin in patients with advanced non-small cell lung cancer. Lung Cancer 81:97–101
- Scagliotti GV, De Marinis F, Rinaldi M, et al. (2002). Italian lung cancer project. Phase III randomized trial comparing three platinum-based doublets in advanced non-small-cell lung cancer. J Clin Oncol 20:4285–91
- Schiller JH, Harrington D, Belani CP, et al. (2002). Eastern cooperative oncology group. Comparison of four chemotherapy regimens for advanced non-small-cell lung cancer. N Engl J Med 346:92–8
- Shin HC, Alani AW, Cho H, et al. (2011). A 3-in-1 polymeric micelle nanocontainer for poorly water-soluble drugs. Mol Pharm 8:1257–65
- Shiah JG, Sun Y, Kopecková P, et al. (2001). Combination chemotherapy and photodynamic therapy of targetable N-(2-hydroxypropyl)methacrylamide copolymer-doxorubicin/mesochlorin e(6)-OV-TL 16 antibody immunoconjugates. J Control Release 74:249–53
- Socinski MA. (2014). Update on taxanes in the first-line treatment of advanced non-small-cell lung cancer. Curr Oncol 21:e691–703
- Stathopoulos GP, Boulikas T. (2012). Lipoplatin formulation review article. J Drug Deliv 2012:581363
- Ulukaya E, Ozdikicioglu F, Oral AY, et al. (2008). The MTT assay yields a relatively lower result of growth inhibition than the ATP assay depending on the chemotherapeutic drugs tested. Toxicol In Vitro 22:232–9
- Vasey PA, Paul J, Birt A, et al. (1999). Docetaxel and cisplatin in combination as first-line chemotherapy for advanced epithelial ovarian cancer. J Clin Oncol 17:2069–80
- Wang P, Zhang L, Peng H, et al. (2013). The formulation and delivery of curcumin with solid lipid nanoparticles for the treatment of on non-small cell lung cancer both in vitro and in vivo. Mater Sci Eng C Mater Biol Appl 33:4802–8
- Xiao H, Li W, Qi R, et al. (2012). Co-delivery of daunomycin and oxaliplatin by biodegradable polymers for safer and more efficacious combination therapy. J Control Release 163:304–14
- Xu X, Xie K, Zhang XQ, et al. (2013). Enhancing tumor cell response to chemotherapy through nanoparticle-mediated codelivery of siRNA and cisplatin prodrug. Proc Natl Acad Sci USA 110:18638–43
- Zhang K, Tang X, Zhang J, et al. (2014). PEG-PLGA copolymers: their structure and structure-influenced drug delivery applications. J Control Release 183:77–86