Abstract
Context: Glioma is a common malignant brain tumor originating in the central nervous system. Efficient delivery of therapeutic agents to the cells and tissues is a difficult challenge. Co-delivery of anticancer drugs into the cancer cells or tissues by multifunctional nanocarriers may provide a new paradigm in cancer treatment.
Objective: In this study, solid lipid nanoparticles (SLNs) and nanostructured lipid carriers (NLCs) were constructed for co-delivery of vincristine (VCR) and temozolomide (TMZ) to develop the synergetic therapeutic action of the two drugs. The antitumor effects of these two systems were compared to provide a better choice for gliomatosis cerebri treatment.
Methods: VCR- and TMZ-loaded SLNs (VT-SLNs) and NLCs (VT-NLCs) were formulated. Their particle size, zeta potential, drug encapsulation efficiency (EE) and drug loading capacity were evaluated. The single TMZ-loaded SLNs and NLCs were also prepared as contrast. Anti-tumor efficacies of the two kinds of carriers were evaluated on U87 malignant glioma cells and mice bearing malignant glioma model.
Results: Significantly better glioma inhibition was observed on NLCs formulations than SLNs, and dual drugs displayed the highest antitumor efficacy in vivo and in vitro than all the other formulations used.
Conclusion: VT-NLCs can deliver VCR and TMZ into U87MG cells more efficiently, and inhibition efficacy is higher than VT-SLNs. This dual drugs-loaded NLCs could be an outstanding drug delivery system to achieve excellent therapeutic efficiency for the treatment of malignant gliomatosis cerebri.
Introduction
Glioblastoma multiforme (GBM, WHO grade IV astrocytoma) is the most common malignant brain tumor originating in the central nervous system in adults (Higa et al., Citation2015). The median survival in patients with GBM is generally less than 12 months after the time of diagnosis because of its rapid progression and invasive nature (Stupp et al., Citation2005). Current therapy includes surgical intervention, radiotherapy and chemotherapy (Messaoudi et al., Citation2014). The standard of care for patients diagnosed with high-grade malignant glioma includes postoperative temozolomide (TMZ) adjuvant to radiation (Buie & Valgus, Citation2012). However, this therapeutic strategy is associated with considerable toxicity and limited efficacy due to the resistance of tumor cells to the drug. Thus, there is an urgent need for more. On the one hand, combined chemotherapy is emerging as an important strategy to overcome drug resistance, maximize the therapeutic effect and reduce side effects (Greco & Vicent, Citation2009; Sarisozen et al., Citation2012). On the other hand, a novel drug delivery system is urgently needed to improve the solubility, prolong the circulation time, enhance the targeted effect and then reduce the systemic toxicity (Chen et al., Citation2013).
Lipid-based nanoparticles have attracted a large attention as possible alternatives to polymeric ones due to their highly biocompatible and biodegradable natural components (Wissing et al., Citation2004). Due to the physicochemical properties of lipids, lipid-based nanocarriers can be easily obtained by direct emulsification of the molten lipids and subsequent recrystallization, avoiding the use of potentially toxic solvents that are commonly required for the preparation of other kinds of nanocarriers (Fathi-Azarbayjani et al., Citation2015; Mattingly et al., Citation2015). Among the different types of lipid-based nanoparticles, solid lipid nanoparticles (SLNs) and nanostructured lipid carriers (NLCs) have come up as the latest development in the arena of lipid-based colloidal delivery systems after nanoemulsion and liposomes (Müller et al., Citation2011). SLNs are composed of the lipid matrix which is solid at body and room temperature (Doktorovova et al., Citation2014). NLCs are constituted of blends of lipids in solid and liquid states, produced by controlled mixing of solid lipids with spatially incompatible liquid lipids, leading to a specific nanostructure (Alam et al., Citation2013; Saupe et al., Citation2005).
U87 malignant glioma cells (U87 MG cells) are the established glioma cell lines that have been widely used for the antiglioma evaluation (Vlachostergios & Papandreou, Citation2015; Zhang et al., Citation2015). In the present study, vincristine- (VCR) and TMZ-loaded SLNs (VT-SLNs) and NLCs (VT-NLCs) was developed and examined in U87 MG cells and in mice bearing glioma xenografts. These two systems were compared all the ways to find out the better one for the more stable dual drugs delivery capacity, more effectively antitumor effect of glioma xenografts and the lower systemic toxicity of tumor-bearing animals.
Materials and methods
Materials
VCR was purchased from Shaanxi Pioneer Biotech Co., Ltd. (Xi'an, China). TMZ was kindly provided by Laimei Pharmaceutical Co., Ltd. (Chongqing, China). Injectable soya lecithin was obtained from Shanghai Taiwei Pharmaceutical Co., Ltd. (Shanghai, China). Stearic acid, dimethyldioctadecylammonium bromide (DDAB), (3-[4,5-dimehyl-2-thiazolyl]-2,5-diphenyl-2H-tetrazolium bromide (MTT), soybean phosphatidylcholine (SPC) were purchased from Sigma Aldrich (St. Louis, MO). COMPRITOL® 888 ATO (888 ATO) was generously provided by Gattefossé (Paramus, NJ). Polyoxyl castor oil (Cremophor ELP) was donated by BASF (Ludwigshafen, Germany). All other chemicals were of analytical grade or higher.
Cells and animals
U87 MG cells were obtained from the American type culture collection (Manassas, VA) and cultured in Dulbecco's modified Eagle's medium (DMEM) (Sigma) supplemented with 10% fetal bovine serum (Fisher Chemicals, Fairlawn, NJ) in a 5% CO2 fully humidified atmosphere.
BALB/c nude mice (5–6 week-old, 18–22 g) were purchased from the Shanghai Slack Laboratory Animal Co., Ltd (Shanghai, China). All animal experiments complied with the Animal Management Rules of the Ministry of Health of the People's Republic of China.
SLNs preparation
SLNs were prepared using the solvent displacement technique (Jiang et al., Citation2012). VCR (50 mg), TMZ (50 mg), stearic acid (1 g) and injectable soya lecithin (1 g) were dissolved in acetone (3 mL). The organic phase was added dropwise into the 0.2% DDAB solution (20 mL), under stirring at 600 rpm and room temperature. When complete evaporation of the organic solvent had occurred, the redundant stabilizers were separated by ultracentrifugation at 8000 rpm and 4 °C for 30 min. The pellet was vortexed and resuspended in Milli-Q water, washed three times, filtered through a 0.45-µm membrane to get VT-SLNs. TMZ-loaded SLNs (T-SLNs) were prepared using the same method by adding only TMZ. The SLNs suspensions obtained were stored at 2–8 °C.
NLCs preparation
NLCs were prepared by the solvent diffusion method (Tiwari & Pathak, Citation2011). The lipid dispersion was composed of 888 ATO, Cremophor ELP and SPC at a ratio of 2:1:1 (w/w/w). Injectable soya lecithin (1 g), VCR (50 mg) and TMZ (50 mg) were dissolved in 1 mL of DMSO and added to the lipid dispersion with heating at the temperature of 70–75 °C to form the lipid phase. Aqueous phase was prepared by dissolving 20 mg of DDAB in 10 mL of water. This aqueous solution was then stirred and heated to 30 °C. The lipid phase was rapidly injected into the stirred aqueous phase (800 rpm) at 30 °C, and the resulting suspension was then dissolved with Milli-Q water and then dialyzed against Milli-Q water for 24 h to get the VT-NLCs. TMZ-loaded NLCs (T-NLCs) were prepared using the same method by adding only TMZ. The NLCs obtained were stored at 2–8 °C.
SLNs and NLCs characterization
The mean particle size, size distribution (PDI) and zeta potential of the obtained SLNs and NLCs were measured by the Malvern Zetasizer Nano ZS (Malvern Instrument Ltd., Worcestershire, UK). The average particle size was expressed as a volume mean diameter.
The drug encapsulation efficiency (EE) and drug loading capacity (DL) of SLNs and NLCs formulations were measured as follows:
For the VCR measurement, high-performance liquid chromatography (HPLC) was applied (Zhang et al., Citation2013). Briefly, ethanol was added to disrupt the SLNs or NLCs, and 20 μL of the resulting transparent solution was injected into an HPLC system (Agilent 1260; Agilent Technologies, Santa Clara, CA). A Kromasil C18 reverse phase column (150 mm × 4.6 mm, 5 μm; AkzoNobel, Separation Products, Bohus, Sweden) and the mobile phase consisting of acetonitrile and 0.01 M NaH2PO4 (55/45, v/v, pH 7.0 adjusted with triethylamine) were used to separate the targeted component. The samples were eluted by the mobile phase at a flow rate of 1.0 mL/min at 35 °C and monitored at 297 nm.
For the TMZ measurement, the HITACHI P-4010 inductively coupled plasma mass spectrometry (ICP-MS) (Hitachi Ltd., Kyoto, Japan) was used. Briefly, 5 mL SLNs or NLCs were centrifuged (16 000 rpm and 4 °C for 30 min) separately, and the supernatants were then determined using the ICP-MS. The EE was expressed as the percentage of the amount of TMZ encapsulated in the NLCs to the total amount of TMZ initially used.
Release studies in vitro
The release of SLNs and NLCs was evaluated by the dialysis method (Yang et al., Citation2013). Briefly, samples were added to the dialysis bag separately. Then, the dialysis bags were incubated with 30 mL of release medium (PBS, pH 7.4) at 37 °C with stirring at 100 rpm. At the predetermined time intervals, 1 mL of solution was removed, and 1 mL of fresh medium was filled to replace the remaining release medium. The amount of VCR and TMZ released from the samples was then determined by the same method described above.
Cytotoxicity study in vitro
The cytotoxicity of SLNs and NLCs formulations was tested in U87 MG cells using the MTT assay (Zaki, Citation2014). Briefly, cells were seeded in a 96-well plate at a density of 5000 cells/well and allowed to adhere for 24 h prior to the assay. Cells were exposed to various concentrations of SLNs and NLCs formulations, free drugs solution, blank SLNs and NLCs, respectively. Culture medium was used as a blank control. After 48 h of incubation, MTT solution (5 mg/mL) was added to each well and the cells were incubated for another 4 h. Cell viability was assessed according to the MTT manufacturer's procedures and the absorbance at 570 nm was measured using a microplate reader (Model 680, Bio-Rad Laboratories Inc., Philadelphia, PA). Cells without the addition of MTT reagents were used as a blank control. The drug concentration causing 50% inhibition (IC50) was calculated using the CalcuSyn software (Biosoft, Ferguson, MO).
Anti-tumor study in vivo
BALB/c nude mice were inoculating subcutaneously in the right armpit with U87 MG cells suspended in PBS for 24 h for the preparation of malignant glioma-bearing animal models. Mice were then divided into nine groups (six mice per group). The VT-NLCs, VT-SLNs, T-NLCs, T-SLNs, free TMZ solution, free VCR solution, blank NLCs, blank SLNs and 0.9% sodium chloride solution (control) were prepared and then injected intravenously into the mice via the tail vein (i.v.). The administration was then repeated once every 3 days over a 21-day therapeutic period.
The measurements were taken in two perpendicular dimensions and tumor volumes (mm3) were calculated by applying the formula: tumor volumes (mm3) = (L × W2)/2, where L is the longest dimension and W is the dimension perpendicular to L (Barua et al., Citation2014).
The antitumor efficacy of each formulation was evaluated by tumor inhibition rate, and was calculated using the following formula: tumor inhibition rate (%) = (Wc − Wt)/Wc × 100. Wt and Wc represent the mean tumor weight of the treated and control groups, respectively.
Statistical analysis
Quantitative data were presented as means ± standard deviation (SD). Statistical significance was analyzed using the Student's t-test with the p value less than 0.05 (p < 0.05) indicating significance.
Results and discussion
SLNs and NLCs characterization
The mean particle size, PDI, zeta potential, EE and DL of SLNs and NLCs formulations were characterized and summarized in . The SLNs formulas have sizes around 180 nm, while the NLCs sizes were about 120 nm. The loading of one or two drugs did not have significant effect on the size of the carriers. The zeta potential of VT-SLNs and VT-NLCs were +35.7 and +29.8 mV, respectively. It is reported that the positive surface charge and proper particle size of nanocarriers were important for efficient drug delivery (Bothun et al., Citation2011), so the obtained cationic SLNs and NLCs may facilitate the delivery of the drugs to the negatively charged cancer cells. The EE of VCR and TMZ encapsulated in SLNs and NLCs was all over 80%. The high EE achieved could offer advantages in the in vitro and in vivo anti-cancer efficiency.
Table 1. Characterization of different vectors.
Release studies in vitro
and show the VCR and TMZ release profiles of the samples, respectively. The drugs were release from SLNs and NLCs at a sustained rate. Both VCR and TMZ release from VT-NLCs was slower than that of VT-SLNs, the complete release of the two drugs from VT-NLCs was about 48 h. While for VT-SLNs, nearly complete release of VCR and TMZ was achieved at 24 and 36 h, respectively. These may indicate that the lipid structure of NLCs helps to retain the drugs inside the carriers, bring about more persistent therapeutic effect than SLNs.
Cytotoxicity study in vitro
The cytotoxicity of SLNs and NLCs formulations was evaluated and compared in U87MG cells at different concentrations. shows that the cell inhibition rates of all formulas conformed to concentration-dependent patterns. The IC50 values of different groups are summarized in . The IC50 value of VT-NLCs (0.23 μM) was 2.4 times dose advantage over T-NLCs (0.55 μM), and 5 times dose advantage over VT-SLNs (1.18 μM). NLCs formulations showed significantly better tumor cells inhibition efficiency than SLNs groups (p < 0.05). Dual drug-loaded NLCs and SLNs exhibited remarkably higher inhibition effect than their single drug-loaded counterparts (p < 0.05). The IC50 of VT-NLCs was the lowest; the reducing viability of malignant glioma cells was the most obviously, accounting for the highest antitumor activity. The results demonstrated the better tumor cell inhibition ability of NLCs formulas than SLNs. Would the NLCs more efficient than SLNs in vivo? The answers could be found in the following studies.
Figure 3. The cytotoxicity of SLNs and NLCs formulations evaluated in U87MG cells at different concentrations.
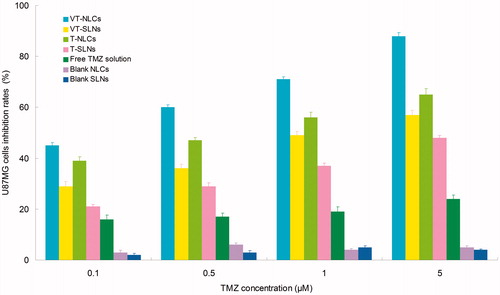
Table 2. IC50 values of different formulations.
Anti-tumor study in vivo
The antitumor study of SLNs and NLCs formulations was evaluated in U87 MG solid tumors in mice. shows the tumor growth inhibition effects after the formulations were treated in vivo. The tumor growths were significantly inhibited by NLCs formulas than SLNs and free solution group (p < 0.05). The tumor regression of VT-NLCs was more obviously than T-NLCs (p < 0.05). At the end of study, the tumor inhibition rates of tumor-bearing mice treated with different formulation are shown in . VT-NLCs inhibited tumor growth over 80%, which is higher than that treated with VT-SLNs (56%) and T-NLCs (70%). The results suggested that the NLCs formulations preformed better than the SLNs ones, and the dual drug-loaded NLCs formula is more efficient than single drug-loaded NLCs. These results in vivo are in accordance with the outcomes in the cytotoxicity studies. VT-NLCs constructed in this research could be better drug delivery system for in vivo dual drugs delivery, and the resulting nanomedicine can be efficient for the treatment of glioma.
Table 3. Tumor inhibition rates of different formulations.
Conclusion
VT-NLCs can deliver VCR and TMZ into U87MG cells more efficiently than their SLNs counterparts. The inhibition efficacies in vitro and in vivo of dual drugs-loaded VT-NLCs are also higher than single drug-loaded vectors. This dual drugs-loaded NLCs could be an outstanding drug delivery system to achieve excellent therapeutic efficiency for the treatment of glioma.
Declaration of interest
The authors report no conflicts of interest. The authors alone are responsible for the content and writing of this article.
References
- Alam MI, Baboota S, Ahuja A, et al. (2013). Intranasal infusion of nanostructured lipid carriers (NLC) containing CNS acting drug and estimation in brain and blood. Drug Deliv 20:247–5
- Barua NU, Hopkins K, Woolley M, et al. 2014. A novel implantable catheter system with transcutaneous port for intermittent convection-enhanced delivery of carboplatin for recurrent glioblastoma. Drug Deliv. [Epub ahead of print]. doi: 10.3109/10717544.2014.908248
- Bothun GD, Lelis A, Chen Y, et al. (2011). Multicomponent folate-targeted magnetoliposomes: design, characterization, and cellular uptake. Nanomedicine 7:797–805
- Buie LW, Valgus JM. (2012). Current treatment options for the management of glioblastoma multiforme. Hematol Oncol Pharm 2:57–63
- Chen M, Chen R, Wang S, et al. (2013). Chemical components, pharmacological properties, and nanoparticulate delivery systems of Brucea javanica. Int J Nanomedicine 8:85–92
- Doktorovova S, Souto EB, Silva AM. (2014). Nanotoxicology applied to solid lipid nanoparticles and nanostructured lipid carriers – a systematic review of in vitro data. Eur J Pharm Biopharm 87:1–18
- Fathi-Azarbayjani A, Ng KX, Chan YW, et al. (2015). Lipid vesicles for the skin delivery of diclofenac: cerosomes vs. other lipid suspensions. Adv Pharm Bull 5:25–33
- Greco F, Vicent MJ. (2009). Combination therapy: opportunities and challenges for polymer-drug conjugates as anticancer nanomedicines. Adv Drug Deliv Rev 61:1203–13
- Higa M, Katagiri C, Shimizu-Okabe C, et al. (2015). Identification of a novel cell-penetrating peptide targeting human glioblastoma cell lines as a cancer-homing transporter. Biochem Biophys Res Commun 457:206–12
- Jiang Z, Sun C, Yin Z, et al. (2012). Comparison of two kinds of nanomedicine for targeted gene therapy: premodified or postmodified gene delivery systems. Int J Nanomedicine 7:2019–31
- Mattingly SJ, O'Toole MG, James KT, et al. (2015). Magnetic nanoparticle-supported lipid bilayers for drug delivery. Langmuir 31:3326–32
- Messaoudi K, Saulnier P, Boesen K, et al. (2014). Anti-epidermal growth factor receptor siRNA carried by chitosan-transacylated lipid nanocapsules increases sensitivity of glioblastoma cells to temozolomide. Int J Nanomedicine 9:1479–90
- Müller RH, Shegokar R, Keck CM. (2011). 20 Years of lipid nanoparticles (SLN and NLC): present state of development and industrial applications. Curr Drug Discov Technol 8:207–27
- Sarisozen C, Vural I, Levchenko T, et al. (2012). Long-circulating PEG-PE micelles co-loaded with paclitaxel and elacridar (GG918) overcome multidrug resistance. Drug Deliv 19:363–70
- Saupe A, Wissing SA, Lenk A, et al. (2005). Solid lipid nanoparticles (SLN) and nanostructured lipid carriers (NLC) – structural investigations on two different carrier systems. Bio-Med Mater Eng 15:393–402
- Stupp R, Mason WP, van den Bent MJ, et al. (2005). Radiotherapy plus concomitant and adjuvant temozolomide for glioblastoma. N Engl J Med 352:987–96
- Tiwari R, Pathak K. (2011). Nanostructured lipid carrier versus solid lipid nanoparticles of simvastatin: comparative analysis of characteristics, pharmacokinetics and tissue uptake. Int J Pharm 415:232–43
- Vlachostergios PJ, Papandreou CN. (2015). Efficacy of low dose temozolomide in combination with bortezomib in U87 glioma cells: a flow cytometric analysis. Arch Med Sci 25;11:307–10
- Wissing SA, Kayser O, Müller RH. (2004). Solid lipid nanoparticles for parenteral drug delivery. Adv Drug Deliv Rev 56:1257–72
- Yang XY, Li YX, Li M, et al. (2013). Hyaluronic acid-coated nanostructured lipid carriers for targeting paclitaxel to cancer. Cancer Lett 334:338–45
- Zaki NM. (2014). Augmented cytotoxicity of hydroxycamptothecin-loaded nanoparticles in lung and colon cancer cells by chemosensitizing pharmaceutical excipients. Drug Deliv 21:265–75
- Zhang B, Dou Y, Xu X, et al. (2015). Endogenous FOXP3 inhibits cell proliferation, migration and invasion in glioma cells. Int J Clin Exp Med 15;8:1792–802
- Zhang T, Zheng Y, Peng Q, et al. (2013). A novel submicron emulsion system loaded with vincristine-oleic acid ion-pair complex with improved anticancer effect: in vitro and in vivo studies. Int J Nanomedicine 8:1185–96