Abstract
Repaglinide (RPG) is a fast-acting prandial glucose regulator. It acts by stimulating insulin release from pancreatic β-cells. Recurrent dosing of RPG before each meal is burdensome remedy. Hence the plan of the present study was to evaluate nanoemulsion as a hopeful carrier for RPG for persistent hypoglycemic effect. The drug was incorporated into oil phase of nanoemulsion to give improved biopharmaceutical properties as compared to the lipid-based systems. Pseudo ternary phase diagrams were prepared by aqueous titration method. Formulations were selected at a difference of 5% w/w of oil from the o/w nanoemulsion region of phase diagrams. The optimized nanoemulsion formulation constituted sefsol-218 (5% v/v) as an oil phase, 30% v/v of Tween-80 and transcutol as a surfactant and co-surfactant to restrain nanodroplet size and low viscosity and distilled water (65%). In vitro dissolution studies showed higher drug release (98.22%), finest droplet size (76.23 nm), slightest polydispersity value (0.183), least viscosity (21.45 cps) and immeasurable dilution capability from the nanoemulsion as compared with existing oral tablet formulation. The optimized RPG nanoemulsion formulation showed better hypoglycemic effect in comparison to tablet formulation in experimental diabetic rats. No significant variations were also observed in the optimized formulation when subjected to accelerated stability study at different temperature and relative humidity over a period of 3 months.
Introduction
Diabetes mellitus is a chronic metabolic disorder characterized by high blood glucose concentrations (hyperglycemia) caused by insulin deficiency that is often combined with insulin resistance (Arunachalam & Gunasekaran, Citation2014; Tripathi, Citation2003). Non-insulin-dependent diabetes mellitus (NIDDM) is a heterogeneous disorder, comprising milder forms of diabetes that occur predominately in adults. Most diabetic patients have IDDM resistance (Prasad et al., Citation2014). Repaglinide (2-ethoxy-4-[[3-methyl-1-[2-(1-piperidyl) phenyl]-butyl] carbamoylmethyl] benzoic acid) (RPG) is a potent second generation oral hypoglycemic agent widely used in the treatment of NIDDM. It acts by stimulating insulin release from pancreatic β-cells (Hadi et al., Citation2012).
Numerous potent lipophilic drugs exhibit low oral bioavailability due to their poor aqueous solubility and cannot be delivered by the oral route of administration in their original form due to instability, low membrane permeability, poor solubility and efflux transport mechanisms (Parveen et al., Citation2011; Akhtar et al., Citation2013). In recent years, lipid-based formulations (incorporation of the active lipophilic component into inert lipid vehicles) are used to improve the oral bioavailability of poorly water-soluble drug compounds, which include micro or nanoemulsions, oils, self-emulsifying formulations, surfactant dispersions, liposomes, solid lipid nanoparticles and lipid nanocarriers. Nanoemulsion offers several advantages over these drug delivery systems such as higher solubilization capacity, rapid onset of action (no extra time for dispersion), reduced intersubject variability in terms of gastrointestinal fluid volume and longer shelf life, toxicological safety, a high content of the lipid phase and the possibility of large scale production by high-pressure homogenization (Mustafa et al., Citation2009; Shakeel & Faisal, Citation2010; Parveen et al., Citation2011; Akhtar et al., Citation2014).
RPG is a fast-acting prandial glucose regulator with a short plasma half-life (∼1 h). Frequent dosing of RPG is cumbersome for patients. Hence, an attempt was made to evaluate nanoemulsion as a promising carrier for RPG for sustained hypoglycemic effect. It is almost exclusively metabolized in the liver and excreted in the bile, with only 8% excreted via the kidneys. This mode of excretion suggests that RPG could be used to treat type 2 diabetic patients who are elderly or renally insufficient, provided that they have sufficient β-cells function (Gumieniczek et al., Citation2009). RPG is the first member of the carbomoyl methyl benzoic acid chemical family to be used in a clinical setting and represents a new class of insulin secretagogues with an insulin-release profile that mimics physiological glucose-stimulated insulin release. RPG stimulates insulin secretion from pancreatic β-cells by closing ATP-sensitive potassium channels, but differs from sulfonylureas in its β-cells binding site (Fuhlendorff et al., Citation1998; Gumieniczek et al., Citation2009). The nanoemulsion of RPG was prepared using oil, surfactant and co-surfactant by titration method. This method is easy to carry out in the normal laboratory conditions and without use of any sophisticated instrument. Thermodynamic stability studies and dispersibility test were carried out and formulation was characterized for size, surface morphology, viscosity, conductivity and refractive index to select the stable and best formulation. In vitro drug release estimation was carried out by dialysis membrane method and compared with conventional dosage form. Antidiabetic potential of RPG nanoemulsion and marketed tablet formulations was also evaluated against streptozotocin (STZ) induced diabetic rats.
Material and methods
Repaginide was granted by Ranbaxy (Gurgaon, India) and Sefsol 218 (Propylene glycol-monocaprylic ester) provided by Nikko Chemicals (Tokyo, Japan) as gift samples. Tween-80 (Polyoxyethylene sorbitan monooleate) and water were procured from Merck (Schuchardh, Hokenbrunn, Germany) and Milli-Q water purification system (Millipore, Billerica, MA), respectively. All other chemicals and reagents used were of analytical (AR) grade and procured from Merck (Mumbai, India) and S.D. Fine Chem. (Mumbai, India). All workings used for the formulation of nanoemulsion were pharmaceutically suitable for oral administration.
Formulation improvement and optimization
Selection of components
Phase solubility studies were made to establish mainly appropriate oil for the preparation of nanoemulsion for RPG. Three millilitres of selected oils [oleic acid, isopropyl myristate (IPM), glycerol triacetate (Triacetin), caproyl 90, propylene glycol monocaprylic ester (Sefsol 218), propylene glycol laurate (Lauroglycol), labrafac] were taken in small vials (5.0 ml capacity) and surplus quantity of drug was added in the oils and set aside in biological shaker (Nirmal International, Delhi, India) for 72 h at a steady temperature (25 ± 1.0 °C) to achieve to an equilibrium (Lawrence & Rees, Citation2000; Leonard et al., Citation2006; Akhtar et al., Citation2014). The samples were detached from shaker and centrifuged at 3000 rpm for 15 min. The supernatant was filtered through a 0.45-μm membrane filter and the concentration of drug was determined by taking absorbance using HPTLC at λmax of 242 nm after dilution (Akhtar et al., Citation2014).
Phase diagram construction
Dissimilar volume ratios (1:0, 1:1, 1:2, 1:3, 2:1 and 3:1) of surfactant (Tween-80) and co-surfactant (Transcutol) mixtures (Smix) were prepared and stocks of 100 ml from each faction were arranged (Chennamsetty et al., Citation2005; Parveen et al., Citation2011; Akhtar et al., Citation2014). For every phase diagram, 16 dissimilar combinations of oil (Sefsol 218) and Smix [1:9, 1:8, 1:7, 1:6, 1:5, 2:8 (1:4), 1:3.5, 1:3, 3:7 (1:2.3), 1:2, 4:6 (1:1.5), 5:5 (1:1), 6:4 (1:0.7), 7:3 (1:0.43), 8:2 (1:0.25), 9:1 (1:0.1)] were made in unusual volume ratios from 1:9 to 9:1; as a result, maximum ratios were covered for the study (Lawrence & Rees, Citation2000; Akhtar et al., Citation2014). The blend of selected oil and Smix were titrated against distilled water. After each 5% accumulation of aqueous phase to the oil and Smix mixture, visual examination was made and documented. The fraction of water, oil and Smix within which nanoemulsion appear were chosen and designed on ternary phase diagrams with one axis characterized the aqueous phase, the other representing the oil and the third representing the Smix. These interpretations were prepared for all Smix ratios in every group individually.
Assortment of formulation commencing phase diagram
Dissimilar formulations were chosen from each phase diagram plotted for different Smix ratios on the basis of succeeding points.
The oil concentration is such that it dissolves distinct amount (2 mg) of RPG simply.
Oil concentration from each phase diagram was chosen as a numerous of five, i.e. 5%, 10% 15% and 20%.
For each oil fraction selected, the concentration of surfactant should be minimum for nanoemulsion preparation (Azeem et al., Citation2009; Akhtar et al., Citation2014).
Thermodynamic stability studies
Preferred formulations were subjected to thermodynamic stability stress tests as heating cooling cycle, centrifugation and freeze–thaw cycle: Heating–cooling cycles between 45 °C temperature and room temperature (25 ± 2 °C) with storage time of 24 h at each temperature (six cycles each) followed by centrifugation (5000 rpm for 30 min) and then Freeze–thaw cycles were finished following storing the samples below −20 °C in a deep freezer (Vest frost, Hyderabad, India) and thawed at room temperature (25 ± 2 °C) for 24 h were carried out 6 times (six cycles each) (Mehnert & Mäder, Citation2001; Faiyazuddin et al., Citation2010).
Dispersibility test
The effectiveness of self-emulsification of oral nanoemulsion was assessed by means of a standard USP XXII dissolution apparatus. One millilitre of nanoemulsion was mixed with 500 ml of media (distilled water and 0.1 N HCl, seperately) and retained at 37 ± 0.5 °C. The dissolution paddle rotated at a speed of 50 rpm to provide gentle mixing. The in vitro performance of the formulations was visually assessed using the following grading system.
Grade A speedily forming (within 1 min) nanoemulsion, showing a lucid or bluish look.
Grade B speedily forming, somewhat fewer obvious emulsions, showing a bluish white appearance.
Grade C clear fair emulsion (comparable to refine in emergence formed within 2 min.
Grade D tedious dirty white emulsions have somewhat oily look that is sluggish to emulsify (longer than 2 min).
Grade E unfortunate or least emulsification with huge oil globules on the surface.
Formulations in Grade A were selected for further studies as it permitted the thermodynamic stability and the dispersibility tests (Akhtar et al., Citation2014).
Formulation of drug restrains nanoemulsion
Drug containing nanoemulsion formulations were arranged by suspending 2 mg/kg body weight of drug in 5%, 10%, 15% and 20% of oil and relevant Smix ratios on vortex mixer and further requisite quantity of water. The resulting mixture gave nanoemulsion.
Rationalization of RPG nanoemulsion
Visual observation
It was carried out distinguish among nanoemulsion with macroemulsion.
Exterior morphology
Transmission electron microscopy (TEM) TOPCON 002B (Topcon, Tokyo, Japan) was used to determine surface morphology of nanoemulsion (Ghosh & Murthy, Citation2006; Akhtar et al., Citation2014). A bend of nanoemulsion was thinned by distilled water (1:100), filtered (0.22 μm) and applied on carbon-coated grid with 2% phosphotungestic acid and set aside it for 30 s. The dehydrated coated grid was taken on a slide and enclosed with a cover slip. The slide was observed under the light microscope operating at 200 kV.
Globule size investigation
Globule size of the nanoemulsion was calculated by photon correlation spectroscopy using Zetasizer 1000 HS (Malvern Instrument, Worcestershire, UK). The formulation was diluted with distilled water and filtered through 0.22 μm membrane filter in order to eliminate multiscattering phenomena and experimental errors. Light scattering was monitored at 25 °C at a scattering angle of 90° (Mehnert & Mäder, Citation2001; Akhtar et al., Citation2014).
Viscosity determination
Viscosity of the formulations was determined by Brookfield DV III ultra V6.0 RV cone and plate rheometer (Middleboro, MA) at 25 ± 0.5 °C (Parveen et al., Citation2011; Akhtar et al., Citation2014).
Refractive index
After calibration with castor oil refractive index of formulation was determined by an Abbes type of refractrometer (Minato-ku, Tokyo, Japan) (Shakeel & Faisal, Citation2010; Parveen et al., Citation2011; Akhtar et al., Citation2014).
Electrical conductivity
Conductometer (CDM 230, Villeurbanne Cedex, France, at 25 ± 1 °C) at the frequency of 94 Hz was used to calculate electrical conductivity of the optimized nanoformulations.
In vitro drug release study
To perform dissolution study dissolution assembly # 2, at 50 rpm and 37 ± 0.5 °C was utilized having 500 ml of distilled water and simulated gastric fluid. Dissolution studies were performed to evaluate the discharge of drug (2 mg RPG) from six dissimilar formulations (RN12, RN13, RN16, RN17, RN18 and RN19) and available RPG tablet (REGAN) manufactured by Ranbaxy India Pvt. Ltd., Gurgaon, India. One millilitre of nanoemulsion was kept in treated dialysis bag. Each millilitre sample was taken at regular time intervals (0, 0.5, 1, 1.5, 2, 2.5, 3, 4, 5, 6, 8 and 24 h) and aliquot quantity of distilled water/simulated gastric fluid was substituted. The samples were analyzed for the drug content using self-developed HPTLC method at 242 nm (Akhtar et al., Citation2013).
Stability studies
The designated nanoemulsion formulations were placed into an air tight glass vials and subjected to stability studies at 8 ± 20 °C (in refrigerator) and at different temperatures and ambient humidity conditions 25 °C/60% and 40 °C/75% RH. Samples were thrilled to stability chambers (Thermolab, Mumbai, India) in glass bottles with humidity and temperature control. Samples were reserved at specified time intervals of 0, 30, 60 and 90 days, respectively, then drug substance, viscosity, particle size, refractive index and electrical conductivity were dogged (Rahman et al., Citation2013). The drug content analysis was approved by validated HPLC method at 242 nm over a period of 3 months under accelerated conditions (Akhtar et al., Citation2013).
Animal study protocol
Approval to carry out in vivo study was obtained from Integral University, Institutional Animal Ethics Committee, Lucknow, Uttar Pradesh (Registration No. IU/Pharm/Ph.D./CPCSEA/10/17) and CPCSEA guidelines were adhered for the complete study. The animals used for in vivo experiments were diabetic Sprague–Dawley rats. The in vivo study was performed to carry out oral administration of RPG formulations in diabetic rats. Diabetes was induced by single Streptozotocin (STZ) injection (100 µg/g i.p.) in rat pups (30–40 g) under extremely aseptic conditions. The general health conditions of rats were observed daily. When rats became adult (125–150 g) they were checked whether diabetic, having blood glucose >300 mg/dl (Ries & Klastersky, Citation1986; Ikebukuro et al., Citation2002; Arulmozhi et al., Citation2004). The blood glucose level was estimated with glucometer One Touch Basic Plus® (Life Scan Inc., Milpitas, CA) using the strips (glucose oxidase method). There were six rats in each group. A treatment schedule is given in .
Table 1. Treatment schedules of repaglinide formulations for antidiabetic activity in SD rats.
Statistical analysis
The pharmacokinetic data used for various formulations were adjudged for statistical significance by the one-way analysis of variance (ANOVA) followed by the Turkey–Kramer multiple comparisons test using Graph Pad Instat software (Graphpad Software Inc., La Jolla, CA).
Results and discussion
Selection of components
Oil characterizes a vital excipient in the nanoemulsion formulation; it can solubilize obvious quantity of the lipophilic drug as well as it can boost the amount of lipophilic drug transportation (Ping et al., Citation2005; Akhtar et al., Citation2014). Sefsol 218 was established to solubilize utmost amount of RPG, i.e. 182.06 ± 7.68 mg/ml to prepare the nanoemulsion (). Consequently, sefsol 218 was preferred as an oil phase designed for the improvement of nanoemulsion. Elevated oil solubility of an inadequately water soluble drug will help an overall stability of the formulation with efficient dose optimization leading to cost effective delivery system for RPG. Tween-80 was preferred as the surfactant and transcutol as the co-surfactant. Surfactant not only reduces the interfacial tension but it also provides a flexible film which can readily deform around the droplets and aid dispersion process. The occurrence of co-surfactants permits the interfacial film enough flexibility to occupy unusual curvatures necessary to shape nanoemulsion over a broad range of composition (Akhtar et al., Citation2014; Prasad et al., Citation2014). Milli-Q water was occupied as an aqueous phase. Each and every one selected excipients for the preparation of formulations were under the GRAS (Generally Regarded as Safe) category.
Figure 1. Bar diagram showing the highest solubility of repaglinide in sefsol 218. HCO, hydrogenated castor oil; CP90, Caproyl 90; SF218, Sefsol 218; T80, Tween-80; T20, Tween-20, TCN, triacetin; LBF, labrafac; LRG, lauroglycol; IPM, isopropyl myristate; OA, oleic acid; S:T 80 (2:1), Sefsol:Tween-80 (2:1); S:T 80 (1:1), Sefsol:Tween-80 (1:1).
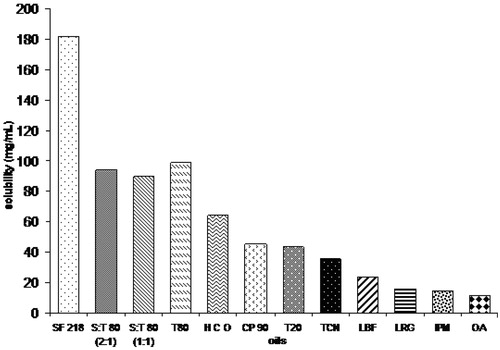
Phase diagram production
Pseudoternary phase diagrams were developed using the aqueous titration method. Deliberate titration with the aqueous phase was achieved for every blend of oil and Smix, independently. The quantity of aqueous phase added was mixed to make a water concentration in the range of 5–95% of sum volume at ∼5% interval. The phase performance of nanoemulsion system containing oil, water and Smix ratio can be studied with the support of ternary phase diagram. Each corner of the phase diagram shows 100% of the exacting constituent. Unique ability was taken to make sure that interpretations are not finished on metastable systems (Parveen et al., Citation2011; Akhtar et al., Citation2014). The pseudoternary phase diagrams were made using sefsol-218 as oily phase, Smix ratio (Tween-80 as a surfactant and transcutol as a co-surfactant) and water as an aqueous phase. In the phase diagrams, only o/w nanoemulsion region is shown, other phases are not revealed because of congestion of the diagrams. Pseudo ternary phase diagrams were built individually for every Smix ratio (). In , surfactant (Smix ratio 1:0) was used alone with no co-surfactant and observed that a low amount of oil (27%, v/v) was solubilized at an elevated concentration of surfactant (48% v/v). Oil solubilization was diminished as the concentration of surfactant was enlarged. There was better solubilization of oil at lower concentration of Smix (1:1) as co-surfactant was added. As a result, the region for nanoemulsion in the phase diagram was enlarged, as shown in . No distinction was observed in nanoemulsion region, as shown in phase diagram in with minute increase in the concentration of co-surfactant (Smix ratio 2:1). In Smix ratio 3:1, there was rise in the nanoemulsion region with increasing concentration of co-surfactant (). This may be attributed to the fact that the addition of co-surfactant may lead to greater penetration of the oil phase in the hydrophobic region of the surfactant monomers thereby further decreasing the interfacial tension, which will lead to increase in the fluidity of the interface and thus increasing the entropy of the system (Akhtar et al., Citation2014). However, the region for nanoemulsion was decreasing because of decreasing oil solubilization (). It may be because of rise concentration of surfactant in Smix 1:2 and 1:3. This shows that the suitable ratio of Smix is essential for an extensive range of nanoemulsion region in phase diagram. Optimized formulations having <27% of the oily phase and least amount of Smix were preferred from the phase diagrams for additional studies.
Thermodynamic stability studies
Chosen formulations were subjected to thermodynamic studies (i.e. heating cooling cycle, centrifugation and freeze–thaw cycle). The observation for thermodynamic stability studies are given in . Instability in the form of phase separation, creaming or cracking was not shown in the selected nanoemulsions. The interfacial energy is more than the entropy in macroemulsions. Hence, energy is required to produce the emulsion by the use of high-speed blender, i.e. the process of emulsification is non-spontaneous while in case of nanoemulsion the interfacial tension is made sufficiently low so that interfacial energy become comparable or even lower than the entropy of dispersion. Hence, the free energy of the system happens to zero or negative (Akhtar et al., Citation2014). The Formulations which not passed the thermodynamic tests were dropped out and the remaining was subjected to dispersibility test.
Table 2. Observations for thermodynamic stability study and dispersibility test of repaglinide nanoemulsion formulations.
Dispersibility tests
The gastrointestinal fluids employed for dilution of nanoemulsion may lead to steady desorption of surfactant located at the globule interface which can precipitate the drug or phase separation of the nanoemulsion producing inadequate formulation. Efficiency of nanoemulsion can be judged by the dispersibility test. Formulations which failed the dispersibility test (grade C, D and E) were discarded ().
Formulation of drug restraining nanoemulsion
The drug was added to the six selected formulations (RN12, RN13, RN16, RN17, RN18 and RN19) as they were passed the dispersibility tests and graded accordingly ().
Characterization of RPG nanoemulsion
Visual examination
The nanoemulsion was clear transparent, easily flowable liquid, whereas the macroemulsion was opaque and milky/cloudy white in appearance.
Exterior morphology
Transmission electron microscopy (TEM) was used to determine morphology and composition of the nanoemulsions droplets. The adjoining was light and the nanoemulsion express dark ().
Droplet size determination (particle dimension and allocation)
Polydispersity indicates the regularity of droplet size inside the formulation. The fraction of SD to mean droplet size is the polydispersity. When the value of polydispersity is low then there will be more uniformity of droplet size in the formulation. Droplet size measurement is not only important parameter to optimize the nanoemulsion formulation but it also helps to differentiate nanoemulsion from microemulsion. The RN13 formulation was presented minimum droplet size (76.23 nm), whereas RN17 and RN18 showed increase in the droplet size due to increased concentration of oil (). The distribution of droplets was in the range of 76–89 nm in formulation RN13 and the maximum droplets (82%) were below a size of 90 nm (). The formulation showed nanodroplets with low values of polydispersity indicating uniformity in the nanoemulsion formulation. The polydispersity values were 0.214, 0.183, 0.195, 0.229, 0.407 and 0.198 for different formulations RN12, RN13, RN16, RN17, RN18 and RN19, respectively ().
Table 3. Droplet size, polydispersity index, viscosity, refractive index and electrical conductivity of selected repaglinide nanoemulsion formulations.
Viscosity determination
The viscosities of the nanoemulsions (RN12, RN13, RN16, RN17, RN18 and RN19) are given in . The viscosity of nanoemulsion formulation was very low as expected as one of the characteristic. It was observed from that viscosity of all the formulations was <24 cps. Formulation RN13 has the minimum viscosity, i.e. 21.447 ± 0.215 cps. Results also revealed that the viscosity is directly proportional to the concentration of oils and surfactants used in the formulation. It can be observed that, in general, viscosity of all the formulations was very low.
Refractive index
Refractive index (RI) being an optical property is used to characterize the isotropic nature of the nanoemulsion. It was observed from that the selected nanoemulsion formulations were chemically stable and remained isotropic in nature, thus having no drug excipient interactions. The observation table shows that as the concentration of the oils increases in the formulation, the RI increases (RN19) ().
Electrical conductivity
Electrical conductivity (σ) was determined to check not only the type of nanoemulsion (o/w or w/o) but also the stability of the nanoemulsion (phase inversion on storage). The conductivity of the formulations is given in the . The lowest σ was found 403.213 ± 1.181 μS/cm for RN17 and the highest was 527.106 ± 2.125 μS/cm for RN12. This indicated that the formulation was o/w type. Electrical conductivity is directly proportional to the percentage of water. Higher the electrical conductivity more will be the percentage of water, which allows more freedom for mobility of ions.
In vitro drug release
Dissolution studies were performed to compare the release of drug from six different formulations (RN12, RN13, RN16, RN17, RN18 and RN19) and marketed formulation, i.e. RPG tablet (REGAN®). The concentration was determined by extrapolation of calibration curve and graph was plotted between time and percent cumulative release (). The pattern of drug release in distilled water and simulated gastric fluid was found very similar to each other in all the formulations. The highest release, i.e. 98.22% was obtained in case of RN13. The minimum release was observed in RN19 formulation (61.90%), this may be due to bigger globule size, which may slow down the release of the drug from nanoemulsion formulation. All the nanoemulsion formulations showed better results as compared to conventional marketed formulation (i.e. tablet) because of small globule size, low viscosity and low polydispersity values. Release of drug from RN19 (10% v/v, oil) was lower than that from RN12, RN13, RN16, RN17 and RN18 (5% v/v, oil) because of higher oil concentration and bigger droplet size. In addition to this, the higher oil concentration may restrain the release of drug into the medium due to lipophilic character of RPG as the partitioning of drug will be more towards the oil. The complete dissolution of RPG in oily phase showed maximum release because of small droplet size, and eventually higher surface area, which permits faster rate of drug release. The RN13 formulation (for each dose, i.e. R1, R2 and R3) was selected for in vivo studies because it offered highest drug release (98.22%), optimum globule size (76.23 nm), minimum polydispersity value (0.183), lower viscosity (21.45 cps) and surfactant concentration (30%).
Stability studies
During stability studies, droplet size, viscosity, drug content and refractive index were determined at 0, 30, 60 and 90 days. These parameters were slightly varied with respect to time but the changes in the observed parameters were not found to be statistically significant (p > 0.05). Stability studies at 8 ± 2 °C, 25 °C/60% RH and 40 °C/75% RH predicted highest degradation of 0.99% of RPG in the optimized RN13 formulation at 40 °C at the end of 90 days.
Biochemical evaluation
The time course profile of blood glucose response in rats is shown in . The blood glucose level in diabetic rats significantly (p < 0.001) reduced in orally administered drug (2 mg) upon 12 h and was reported as 109.99 ± 7.92 mg/dl from 304.40 ± 20.73 mg/dl, it was 169.02 ± 18.18 mg/dl (p < 0.01), 99.74 ± 8.52 mg/dl (p < 0.001) and 100.36 ± 9.50 mg/dl (p < 0.001) from 301.73 ± 12.53 mg/dl, 302.32 ± 11.21 mg/dl and 309.63 ± 15.21 mg/dl for 12 h in case of orally administered nanoemulsion containing 0.5, 1.0 and 2 mg of RPG. The findings showed nanoemulsion (having 1 mg RPG) significantly decreased blood glucose level which was almost similar to oral conventional formulation of 2 mg RPG in diabetic rats.
Table 4. Effects of repaglinide nanoemulsion on glucose level in the control and experimental groups of rat.
Conclusions
Repaglinide nanoemulsion was successfully prepared with an optimized composition including 5% v/v of sefsol 218 as the oily phase, 30% v/v of Smix (Tween-80 as surfactant, transcutol as the co-surfactant, 2:1) and 65% v/v of distilled water as an aqueous phase. That above formulation was optimized on the basis of optimum globule size, minimum polydispersity index, higher drug release, lower viscosity, lower surfactant concentration and higher solubilization of drug in minimum amount of oil.
Results from the stability studies at 8 ± 2 °C, 25 °C/60% RH and 40 °C/75% RH indicated stability of the optimized formulation as there was no significant change in the observed physical parameters. The optimized preparation presented good antidiabetic activity in experimental diabetic rats for 12 h. It may be due to its nanosize and higher surface area, which permits faster rate of drug release and better absorption followed by better bioactivity in lesser dose of drug.
Acknowledgements
The authors are thankful to Ranbaxy (Gurgaon, Delhi) for providing gift sample of drug. They are also thankful to Professor S. W. Akhtar, Vice Chancellor of Integral University, Lucknow, India, for providing the necessary facilities to carry out the present research work.
Declaration of interest
Authors declare no conflict(s) of interest.
References
- Akhtar J, Fareed S, Aqil M. (2013). Stability-indicating assay of repaglinide in bulk and optimized nanoemulsion by validated high performance thin layer chromatography technique. J Pharm Bioallied Sci 5:184–90
- Akhtar J, Hussain Siddiqui H, Badruddeen, et al. (2014). Nanomulsion as a carrier for efficient delivery of metformin. Curr Drug Deliv 11:243–52
- Arulmozhi DK, Veeranjaneyulu A, Bodhankar SL. (2004). Neonatal streptozotocin-induced rat model of Type 2 diabetes mellitus: a glance. Indian J Pharmacol 36:217–18
- Arunachalam S, Gunasekaran, S. (2002). Tuberculosis research in India and China: from bibliometrics to research policy. Curr Sci 82:933–47
- Azeem A, Rizwan M, Ahmad FJ, et al. (2009). Nanoemulsion components screening and selection: a technical note. AAPS PharmSciTech 10:69–76
- Chennamsetty N, Bock H, Scanu LF, et al. (2005). Cosurfactant and cosolvent effects on surfactant self-assembly in supercritical carbon dioxide. J Chem Phys 122:094710–11
- Faiyazuddin M, Baboota S, Ali J, et al. (2010). Chromatographic analysis of trans and cis-citral in lemongrass oil and in a topical phytonanocosmeceutical formulation, and validation of the method. J Planar Chromatogr Mod TLC 23:233–6
- Fuhlendorff J, Rorsman P, Kofod H, et al. (1998). Stimulation of insulin release by repaglinide and glibenclamide involves both common and distinct processes. Diabetes 47:345–51
- Ghosh PK, Murthy RSR. (2006). Microemulsions: a potential drug delivery system. Curr Drug Deliv 3:167–80
- Gumieniczek A, Krzywdzinska M, Nowak M, et al. (2009). Modulation of nitrosative/oxidative stress in the lung of hyperglycemic rabbits by two antidiabetics, pioglitazone and repaglinide. Exp Lung Res 35:371–9
- Hadi NR, Rezeg FA, Al-Amran F, et al. (2012). Evaluation of the effects of glimepiride (Amaryl) and repaglinide (novoNorm) on atherosclerosis progression in high cholesterol-fed male rabbits. J Cardiovasc Dis Res 3:5–11
- Ikebukuro K, Adachi Y, Yamada Y, et al. (2002). Treatment of streptozotocin-induced diabetes mellitus by transplantation of islet cells plus bone marrow cells via portal vein in rats. Transplantation 73:512–18
- Lawrence MJ, Rees GD. (2000). Microemulsion-based media as novel drug delivery systems. Adv Drug Deliv Rev 45:89–21
- Leonard TW, Lynch J, McKenna MJ, et al. (2006). Promoting absorption of drugs in humans using medium-chain fatty acid-based solid dosage forms: GIPET™. Expert Opin Drug Deliv 3:685–92
- Mehnert W, Mäder K. (2001). Solid lipid nanoparticles: production, characterization and applications. Adv Drug Deliv Rev 47:165–96
- Mustafa G, Khan ZI, Bansal T, Talegaonkar S. (2009). Preparation and characterization of oil in water nano-reservoir systems for improved oral delivery of atorvastatin. Curr Nanosci 5:428–40
- Parveen R, Baboota S, Ali J, et al. (2011). Oil based nanocarrier for improved oral delivery of silymarin: in vitro and in vivo studies. Int J Pharm 413:245–53
- Ping L, Ghosh A, Wagner RF, et al. (2005). Effect of combined use of nonionic surfactant on formation of oil-in-water microemulsions. Int J Pharm 288:27–34
- Prasad PS, Imam SS, Aqil M, et al. (2014). QbD-based carbopol transgel formulation. Characterization, pharmacokinetic assessment and therapeutic efficacy in diabetes. Drug Deliv. [Epub ahead of print]. http://dx.doi.org/10.3109/10717544.2014.936536
- Rahman MA, Hussain A, Hussain MS, et al. (2013). Role of excipients in successful development of self-emulsifying/microemulsifying drug delivery system (SEDDS/SMEDDS). Drug Dev Ind Pharm 39:1–19
- Ries F, Klastersky J. (1986). Nephrotoxicity induced by cancer chemotherapy with special emphasis on cisplatin toxicity. Am J Kidney Dis 8:368–79
- Shakeel F, Faisal MS. (2010). Nanoemulsion: a promising tool for solubility and dissolution enhancement of celecoxib. Pharm Dev Technol 15:53–6
- Tripathi KD. (2003). Essential of medical pharmacology. New Delhi: Jaypee Brother’s Medical Publishers (P) Ltd., 247–51