Abstract
Purpose: Microspheres of chitosan (CS) cross-linked with polyethylene glycol (PEG) were prepared by emulsion-cross-linking followed by the solvent evaporation technique. The formulations were characterized and subjected to in vitro and in vivo tests to assess cell growth, changes in cell morphology, and activities by 3-(4,5-dimethylthiazol-2-yl)-2,5-diphenyltetrazolium bromide (MTT) assay on human HT-29 colon cancer cell-lines.
Methods: In vivo activity was evaluated for dimethyl hydrazine-induced colorectal cancer in albino male Wistar rats. Biochemical and histological parameters were evaluated to understand their effectiveness for colon cancer therapy.
Results: The 5-FU immediate release (IR) formulations suspended in SCMC produced an immediate cytotoxic effect, whereas microspheres inhibited proliferation of tumor cells to induce apoptosis over an extended time. Minimum inhibitory concentration (IC50) values for both standard plain 5-FU and 5-FU-loaded microspheres were respectively 5.00 ± 0.004 µg/mL and 165 ± 1.9 µg/mL which showed the improved safety profile of the microsphere formulation. Tissue distribution showed high concentration of 5-FU in colon that was higher than IC50 value required to stop the growth or death of colon cancer cells from the colonic dysplasia in Duke’s stage A. Significant reduction in tumor volume and multiplicity was observed with increased levels of liver enzymes in animals when treated with standard 5-FU formulation compared with 5-FU loaded microspheres. Elevated levels of serum albumin, creatinine, leukocytopenia, and thrombocytopenia were observed in animals for the standard 5-FU formulation.
Conclusion: The PEG cross-linked CS microspheres of this study slowly released 5-FU up to 24 h to colonic region and enhanced the antitumor activity.
Introduction
Colorectal cancer (CRC) is the most common disease prevalent in males and females (Jemal et al., Citation2011). Patients suffering from the advanced CRC are treated with chemotherapy as well as supportive care (Ishikawa et al., Citation1998; Meropol Citation1998). The widely used anticancer drug namely, 5-flurouracil (5-FU), a pyrimidine antimetabolite (Labianca et al., Citation2001), is known to act by inhibiting thymidylate synthase. In clinical practice, intravenous (iv) route is the preferred method over oral route as the latter has problems of erratic absorption of 5-FU (Djordjeuic et al., Citation1993; Herrmann, Citation1993), but oral route is widely preferred over iv treatment because of its side effects and patient compliance. In this pursuit, numerous efforts have been made to target drugs to colon such as prodrugs, pH-dependent and time release systems, microflora-activated systems, and intestinal-pressure colon delivery capsules (Torchillin, Citation1998; Yang et al., Citation2002; Chourasia & Jain, Citation2004a, b; Torchillin, Citation2007; Ghorab et al., Citation2011; Ramasamy et al., Citation2013; Rai et al., Citation2014; Sareen et al., Citation2014; Zeng et al., Citation2015). The wide pH range of gastro intestinal (GI) tract poses a challenge for the delivery of drug to the colon and hostile environment of the stomach become ineffective due the failure of a delivery device. To overcome this problem, enteric coating of the device matrix has been attempted (Ghorab et al., Citation2011; Rai et al., Citation2014; Sareen et al., Citation2014; Zeng et al., Citation2015).
In this research, chitosan (CS, a cationic polysaccharide, soluble, or swellable in acidic pH, but deswells in neutral or alkaline pH) is used as an oral delivery matrix for 5-FU to the colon (Kulkarni et al., Citation2005). In a previous study, pH-independent swelling characteristics was imparted to CS by cross-linking with a low molecular weight poly(ethylene) glycol (PEG) followed by enteric coating with cellulose acetate phthalate (CAP) to prolong the release of 5-FU to colon (Ganguly et al., Citation2011). Such cross-linking with PEG has several inherent advantages as it is devoid of toxic side effects due to pendant aldehyde formation as well as its deswelling property in alkaline media.
Recently, there has been a growing interest in multiparticulate delivery systems comprising polyelectrolyte complexes of CS with carboxymethyl starch (Assaad et al., Citation2011), chitosan-carboxymethyl konjac glucomannan (Du et al., Citation2006) and chitosan heparin for the delivery of albumin (Liu et al., Citation2007), heparin/CS nanoparticle carriers prepared by polyelectrolyte complexation (Liu et al., Citation2007) and CS-polyaspartate (Zhang et al., Citation2008) for delivering 5-FU. Folic acid-conjugated CS has also been used to formulate nanoparticles for targeted delivery of 5-aminolevulinic acid (5-ALA) to the colon (Li et al., Citation2011), wherein conjugation of folic acid imparts high affinity for colorectal cancer cells showing the overexpression of folate receptors. Chitosan and tamarind kernel powder interpolymer complex films have also been investigated for colon drug delivery (Kaur et al., Citation2010). Hitherto, only one attempt has been made to test the pharmacodynamics of 5-FU colon delivery systems for the treatment of CRC (Mundargi et al., Citation2007) using sodium alginate microspheres coated with Eudragit S100 that showed improved bioavailability and sustained release of 5-FU in the colon.
In our earlier in vitro study (Ganguly et al., Citation2011), pH-independent swelling behavior was imparted to chitosan microspheres by cross-linking it with PEG which prevented the chitosan from deswelling at alkaline pH. These were enteric coated with CAP that served as the dual purpose device for bypassing the 5-FU release in acidic media of the stomach to achieve the controlled release of 5-FU over an extended period of time. In continuation of that study, we now present in vitro and in vivo studies on the same formulations to compare their release data with 5-FU immediate release (IR) formulations suspended in SCMC assessed by MTT assay on human HT-29 colon cancer cell-lines. The formulations are subjected to GI distribution, pharmacokinetic, and pharmacodynamic evaluations (28) to understand the effect of physiological parameters such as enzymes and pH, which affect the drug release, providing improved therapeutic efficacy than the plain 5-FU. The reduction in systemic side effects due to sustained, but reduced dose exposure of colonic tumor to 5-FU released from the microspheres was evaluated by estimating different liver enzymes [serum glutamic oxaloacetic transaminase (SGOT), serum glutamate-pyruvate transaminase (SGPT), Alkaline phosphatase (ALP)], serum albumin, creatinine. The myelosuppressive effect of 5-FU-loaded novel microspheres was also evaluated. X-ray radiographic studies on albino mice were carried out to confirm the integrity of the delivery system in vivo. The pharmacodynamic efficacy of these pH-sensitive microspheres with a reduction in toxicity has been highlighted, thereby suggesting their potential therapeutic benefits.
Materials and methods
Materials
CAP-coated PEG-CS microspheres were prepared as described before (Rai et al., Citation2014). The composition of 5-FU standard formulation is as follows: 50 mg/mL 5-FU suspended in 0.5% w/v sodium carboxymethyl cellulose (SCMC). The 1,2-dimethylhydrazine (DMH) was purchased from Sigma-Aldrich (Munich, Germany). Kits for the measurement of serum glutamate oxaloacetate tramsaminase (SGOT), glutamate pyruvate transaminase (SGPT), albumin, creatinine, and alkaline phosphatase were purchased from Bayer India Ltd. (Mumbai, India). Sodium carboxymethylcellulose, ethylene diamine tetra acetic acid, and formalin were purchased from S.D. Fine Chemicals Ltd. (Mumbai, India). All other solvents and reagents were of high-performance liquid chromatography (HPLC) or analytical grade.
Preparation of PEG-cross-linked chitosan microspheres
PEG-cross-linked CS was prepared as per the method developed before (Ganguly et al., Citation2011). In brief, both CS and PEG were dissolved separately in formic acid (500 mg/10 mL); both the solutions were mixed by stirring on a magnetic stirrer for 15 min to which formaldehyde (500 μL) was added and mixed thoroughly for 2 h to complete the cross-linking at 45 °C. Then 30% w/w 5-FU was added to the reaction mixture. The entire solution was poured into 100 mL of liquid paraffin oil containing 1% (w/w) Tween-80 and stirred for 4 h using an overhead stirrer at the rotation speed of 1500 rpm at 45 °C. The microspheres were centrifuged, washed with petroleum ether followed by acetone, and deionized water to remove the unreacted formaldehyde. For preparing 5-FU-loaded PEG cross-linked microspheres, a similar method was adopted by mixing weighed quantities of 5-FU, i.e. 10%, 20%, and 30% w/w in the polymer solution before emulsification. The dried microspheres were coated with CAP using a spray coating method. The barium sulfate-loaded microspheres were prepared by dispersing barium sulfate in PEG-CS matrix followed by enteric coating with CAP.
Microsphere characterization
5-FU-loaded and CAP-coated microspheres were observed under scanning electron microscope (JSM-840A scanning electron microscope, Jeol, Tokyo, Japan) after a gold sputtering (JFC-1100E sputter coater, Jeol, Tokyo, Japan) in a high vacuum evaporator to study particle morphology and estimate the particle size. Microspheres were also evaluated for their encapsulation efficiency, equilibrium swelling and in vitro drug release (2 h in acidic media followed by alkaline media of pH 7.4 for 24 h) as described previously (Ganguly et al., Citation2011).
Animals
Male Wistar rats, aged 4 weeks, weighing 50–100 g, were obtained from Venkateshwara Enterprises, Bangalore, India. They were housed in propylene cages (six rats per cage) with husk bedding in animal room under constant temperature and humidity conditions (22 ± 2 °C and 45 ± 5% relative humidity) with 12 h light and dark cycle. Rats were fed standard rodent feed (Amruth Food, Sangli, India) and drinking water ad libitum. All animals used in the experiments were taken care of in compliance with the Principles of Laboratory Animal Care and Guidance for the Care and Use of Laboratory Animals. The experimental protocol was carried out after approval from the Institutional Animal Ethical Committee, SET’s College of Pharmacy, Dharwad, India (Approval no. SETCP/IAEC/2011-2012/466).
Cell culture
HT-29 (colon cancer) cell culture was procured from the National Centre for Cell Sciences (NCCS), Pune, India. Stock cells were cultured in Dulbecco’s Modified Eagle Medium (DMEM) supplemented with 10% inactivated fetal bovine serum (FBS), penicillin (100 IU/mL), streptomycin (100 µg/mL), and amphotericin B (5 µg/mL) in an humidified atmosphere of 5% CO2 at 37 °C until confluent. The cells were dissociated with TPVG solution (0.2% trypsin, 0.02% ethylene diamine tetra acetic acid (EDTA), and 0.05% glucose in PBS). The stock cultures were grown in 25 cm2 culture flasks and all the experiments were carried out in 96 microtitre plates (Tarsons India Pvt. Ltd., Kolkata, India). The culture medium was changed every other day until confluence was reached. The monolayer cultures of HT-29 cell-lines were obtained at the end of 48 h of incubation at 37 °C.
Cell growth curves
The monolayer cell culture was trypsinized and the cell count was adjusted to 1.0 × 105 cells/mL using DMEM medium containing 10% FBS. To each well of the 96-well microtitre plate, 0.1 mL of diluted cell suspension (approximately 10 000 cells) was added. After 24 h, when a partial monolayer was formed, the supernatant was flicked off, washed the monolayer once with medium and 100 µL of different test concentrations of test drugs were added onto the partial monolayer in microtitre plates. Before starting cytotoxicity experiments, a calibration curve of HT-29 cell-line was established for different concentrations (i.e. the number of cells/mL plotted on x-axis versus absorbance at 540 nm), which was used to calculate the cell concentrations after treatment with different concentrations of 5-FU (for both free and encapsulated forms). Absorbance was determined using a UV–visible spectrophotometer (Biotek, Winooski, VT).
MTT assay
Cell viability was determined by using 3-[4,5-dimethylthiozol-2-yl]-2,5-di phenylterrazolium bromide (MTT)-based cytotoxicity assay (Freshney, Citation1994; Routledge et al., Citation1994). MTT is a yellow water-soluble tetrazolium dye that is reduced by live cells to a purple formazan product that is insoluble in aqueous solutions. Dilutions of 5-FU in the growth medium was prepared by placing 100 µL of 5-FU solution into the first well and serially diluting to obtain seven different concentrations. After 48 h of incubation, HT-29 cell-lines were treated with various concentrations of 5-FU, blank CAP-coated PEG-CS MPs, and 5-FU-loaded CAP-coated PEG-CS MPs.
Surviving cell numbers were determined indirectly by MTT dye reduction. In brief, the plates were incubated at 37 °C for 3 d in 5% CO2 atmosphere and microscopic examination was carried out at every 24 h interval. After 72 h, drug solutions in the wells were discarded and 50 µL of MTT in PBS was added to each well. The plates were gently shaken and incubated for 3 h at 37 °C in 5% CO2 atmosphere. The supernatant was removed and 100 µL of propanol was added and plates were gently shaken to solubilize the formed formazan. The absorbance was measured using a microplate reader at a wavelength of 540 nm.
The % growth inhibition was calculated using the following formulas:
Note: OD refers to optical density.
The concentration of drug needed to inhibit cell growth by 50% (CTC50) values was generated from the dose–response curves for each cell-line. Cell morphology and distributions were studied and photographed by light microscopy (Kodak C43, Kodak Inc., Tokyo, Japan); results indicate the mean of at least three sets of experiments. A similar procedure was adopted for aliquots obtained at different time intervals after dissolution of 5-FU-loaded microspheres.
Pharmacokinetic and gastrointestinal distribution studies
The efficacy of 5-FU microspheres for local release to colon site was assessed in a rat model by comparing with a control treatment (5-FU suspended in 0.5% w/v sodium carboxy methyl cellulose (SCMC)). Animals were fasted for 12 h overnight before the studies and water was available ad libitum during the experiments. Rats were divided into three groups of six animals each. Group I received normal saline, Group II received 5-FU immediate release (IR) formulations suspended in SCMC, and Group III received 5-FU-loaded microspheres. The dose of 5-FU to be administered was calculated according to surface area of rat’s colon (0.0023 × 500 × 7 = 8.05 mg) (Preezel & Webling, Citation1971). Animals were given appropriate formulation containing equivalent of 8.05 mg of 5-FU by peroral route. Blood was collected by retro-orbital bleeding under light anesthesia at 0, 1, 2, 4, 6, 12, and 24 h after administration of 5-FU. Around 2 mL of blood was collected into centrifugation tubes by retroorbital method, centrifuged at 3000 rpm for 10 min and the serum was stored at −20 °C for later measurement of 5-FU concentration by HPLC. Animals were randomized before collection of blood at each time point to minimize the stress effects.
Animals were euthanized by deep anesthesia and carcasses were placed on ice packs and opened by bilateral thoracotomy. The entire GI tract was removed and mesenteric and fatty tissues were separated. The GI tract was segmented into stomach, small intestine, caecum, and colon. The contents of lumen were removed by gentle pressure with wet scissors, and organs were cut longitudinally and washed with normal saline (0.9% w/v NaCl) to remove the remaining luminal contents. Caecal and colon contents were taken from caecum and colon at each time point. Organs were weighed and cut into small pieces, homogenized at 4 °C with a tissue homogenizer (type RQ127A) (Remi Motors Ltd., Mumbai, India) and centrifuged at 3000 rpm for 10 min at 4 °C. The fatty layer was discarded and the amount of 5-FU in the supernatant was determined by HPLC.
HPLC analysis of 5-FU
The HPLC system (Shimadzu HPLC class VP series, Shimadzu Inc., Kyoto, Japan) consisted of two LC 20 AT pumps, a variable wavelength programmable UV–Vis detector (SPD-20 A) and a reverse phase C18 column (250 × 4.6 mm i.d., particle size 5 µm; Phenomenex, Hyderabad, India) and a manual injection valve was equipped with a 20 µL sample loop. The 5-FU was extracted from plasma by mixing rat plasma with ethyl acetate and isopropanol (85/15, v/v), samples were dried at 37 °C and the dehydrated samples were dissolved in 400 µL of mobile phase diluents for subsequent analysis by HPLC.
Separations were done at 25 °C using 0.01 M KH2PO4 as the mobile phase, which was filtered and delivered at a flow rate of 1.0 mL min−1. The column was maintained at 25 °C and eluent was detected by UV detector at 266 nm. Pharmacokinetic (PK) parameters were calculated by non-compartment analysis based on the statistical moment theory using Microsoft Excel software PKsolver (PharmPK, St. Louis, MO). Area-under-the-plasma concentration–time curve up to the last time (t) (AUC0–t), area-under-the-curve extrapolated to infinity (AUC0–∞), and area-under-the-first moment curve extrapolated to infinity (AUMC0–∞) were all calculated using the linear trapezoidal rule. The mean residence time (MRT) was calculated as AUMC/AUC.
Biodistribution studies
The concentration of 5-FU in serum or tissue homogenate was determined using the method reported by Yan et al. (Yan et al., Citation2010) with some modifications. The mobile phase was 0.01 M KH2PO4 that was delivered at a flow rate of 1.0 mLmin−1 and 5-FU was detected at 266 nm. An aliquot (0.5 mL) of serum or tissue sample was measured into the homogenization tube, PBS was added at a three-fold volume of the weight of the tissue, the mixture was homogenized for 2 min and the homogenate was centrifuged at 2000g for 10 min. The homogenate was centrifuged at 2000g for 10 min followed by three times extraction with 6 mL ethyl acetate and isopropanol (85:15 v/v) mixture. The organic phase was separated and evaporated to dryness. The extracted 5-FU was dissolved in PBS, filtered through 0.22 µm membrane and subjected to HPLC analysis. The concentrations of 5-FU in tissue samples were determined. Consequently, the amount of 5-FU in each tissue was calculated from the concentration and tissue weight; recovery ranged from 94% to 105% for various tissues.
Pharmacodynamic studies
After 2 weeks of acclimatization, rats were randomly divided into three groups. All rats were given 20 mg. kg−1 subcutaneous injection of DMH (10% w/v solution in sterile normal saline with EDTA) in the groin region weekly for 4 weeks (Li & Li, Citation2003). Rats in Group I were given normal saline at the same time for the same period of experiment. After cancer induction, animals were given different formulations as per the treatment protocol outlined before. Groups II and III were given the 5-FU immediate release (IR) formulations suspended in SCMC or 5-FU-loaded microsphere, respectively. Group IV served as normal control without any cancer induction receiving normal saline throughout the study period. After 1, 2, 3, and 4 weeks of treatment with 5-FU formulations, six animals from Groups II and III were killed by deep ether anesthesia, while controls were killed at the end of the study. Total duration of the experiment was 14 weeks.
Tissue processing
The carcass was opened by bilateral thoracotomy immediately after death and around 10 mL of blood was withdrawn by cardiac puncture. Sodium EDTA was added to 2 mL blood sample for the determination of white blood cell (WBC) and platelet counts. The remaining blood was centrifuged at 2000 rpm for 10 min to separate the serum for biochemical estimations. Buffered formalin phosphate (10%) was immediately injected into the colon by intubation into the anus, such that the colon and small intestine were distended and fixed. The colons were removed and placed on plastic sheets, flushed with normal saline and then cut longitudinally. The number and the location of tumors in each animal were determined. Tumor width (W) and length (L) were measured with calipers to determine (Yoon et al., Citation1999) the tumor volume (TV) as TV = (L × W2)/2. Colon samples were fixed between the sheets of Whatman filter paper and stored in 10% buffered formalin phosphate for histological examinations. Afterwards, colon tissues were embedded in paraffin, sectioned, and stained with hematoxylin and eosin. The stained sections were examined histologically for tumor type and Duke’s stage.
Biochemical estimations
WBC and platelets were determined by a cell counter (Sysmex-K100, Transasia Biomedicals Ltd., Mumbai, India). SGOT and SGPT, alkaline phophatase, creatinine, and albumin concentration were estimated by a UV kinetics method (Bradley et al., Citation1972), p-nitrophenyl phosphate (PNPP) method (Bowers, Citation1980), alkaline picric acid method (Moss & Henderson, Citation1999) as well as the BCG (Bromocresol green) method (Doumas et al., Citation1981) as described by the International Federation of Clinical Chemistry (IFCC). The level of SGOT, SGPT, albumin, creatinine, and alkaline phophatase in the serum was determined using a biochemistry analyzer (Type MS-500 E) (Maysun Technology Co. Ltd., Mumbai, India).
Determination of aberrant crypt foci (ACF)
Bird (Bird, Citation1987) described a method for the determination of aberrant crypt foci. In brief, at the end of the 16 week study, rat colons were removed and flushed with potassium phosphate-buffered saline (0.1 M, pH 7.2). Colons were split open longitudinally and placed on strips of filter paper with their luminal surface open and exposed. Another strip of filter paper was placed on top of the luminal surface. The colons were then secured and fixed in a tray containing 10% buffered formalin overnight. Each fixed column was cut into proximal and distal portions of equal length and each portion was cut further into 2 cm long segments. Each segment was placed in a Petri dish and stained with 0.2% methylene blue solution for 2 min. The segments were then transferred to another Petri dish containing buffer to wash off excess stain. The segments were examined using a light microscope at low magnification to score the total number of ACF as well as the number of crypts per focus. ACFs were distinguished from the normal crypts by their thicker, darker stained, raised walls with elongated slit-like lumens and significantly increased distance from the lamina to basal surface of cells. ACFs in the colon were counted.
After visualization of crypt outlined dye was removed and sections were prepared for further classification by hematoxyline and eosin (H&E). This was done by random (distal part of colon) selection, embedded in paraffin wax, and serially cut into perpendicular section to the surface (5 µm). The paraffin was removed with xylene and rehydration was done. The dissected sections were stained with H&E and evaluated for proliferative activity.
In vivo X-ray radiography
The barium sulfate-loaded enteric coated and uncoated PEG-CS microspheres were administered orally with 1 ml of water to Swiss mice (n = 3; 30–35 g) that were fasted overnight with free access to water. Fluoroscopic images of the mice were taken before administration (0 h) and at 1 h, 2 h, 3 h, 4 h, and 6 h to trace the in vivo movement and behavior of the microspheres in the gastrointestinal tract. X-ray images of the mice in prone position were captured using L&T Vision 100 (C-arm) Xray machine (Larsen & Toubro Limited, Mumbai, India), at 64 mAs and 63 kV voltage.
Statistical analysis
Graph pad Instat 3 software was used for statistical analysis (GraphPad Software Inc., San Diego, CA). Data are expressed as mean ± SEM (standard error of mean). Data were analyzed by one-way analysis of variance (ANOVA) followed by student’s T/Newman Keul multiple comparison test. The unpaired Student’s “t” test was used where applicable and differences were considered significant when p < 0.05.
Results
5-FU-loaded and CAP-coated PEG-CS microspheres were prepared, characterized, tested for cytotoxic activity using MTT assay and anticancer activity was evaluated in DMH-induced colorectal cancer in rats. shows the scanning electron micrograph of 30% w/w 5-FU-loaded CAP-coated and uncoated PEG-CS microspheres. On one hand, uncoated microspheres were spherical in shape with wrinkles and pores, which may be due to the evaporation of solvent. On the other hand, the surface of CAP-coated microspheres was smooth without any pores or wrinkles. The mean particle size of CAP-coated 5-FU-loaded PEG-CS microspheres was 316 ± 20 μm. The encapsulation efficiencies of 10%, 20%, and 30% (w/w) 5-FU microspheres were 22.4 ± 3.5%, 23.8 ± 3.2%, and 30.4 ± 4.0%, respectively. The % cumulative 5-FU release were ∼88%, 89%, and 97%, respectively at the end of 24 h ()
Figure 1. SEM images of (A) CAP-coated PEG-CS microspheres at 250× magnification, and (B) uncoated PEG-CS microspheres at 120× magnification.
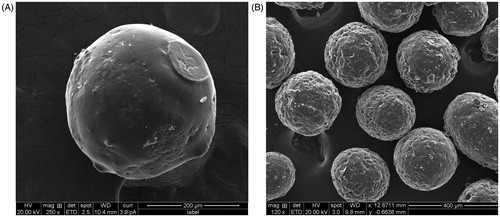
Figure 2. In vitro release of 5-FU from the CAP-coated and uncoated PEG-cross-linked CS microspheres (namely F1 and CF1) at 37 °C in pH 1.2 for 2 h followed by pH 7.4 up to 12 h.
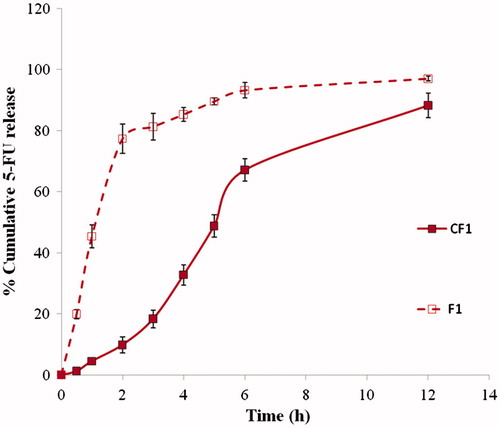
Cytotoxicity studies
The cytotoxic activity of 5-FU- and CAP-coated PEG-CS microspheres was assessed by MTT assay. The concentration range of 5-FU immediate release (IR) formulations suspended in SCMC was selected based on National Cancer Institute guidelines. As seen in , marked reduction in cell viability was observed when human HT-29 colorectal cancer cell-lines were incubated with 5-FU immediate release (IR) formulations suspended in SCMC, which showed an immediate cytotoxic effect with a CTC50 value 5.00 ± 0.004 µg/mL. It is also seen in that a lesser reduction in cell viability was observed with lower (1.56 µg/mL) concentration of 5-FU where the cell viability was reduced to 39% after 24 h. Incubation with high concentration of 5-FU immediate release (IR) formulations suspended in SCMC reduced the viability to ∼80 to 86% after 24 h. The cytotoxicity of blank microspheres was studied by using different amounts of CAP-coated PEG-CS microspheres in the range of 31–1000 µg/mL (). However, no significant reduction in cell viability was observed with the control microspheres (blank CAP-coated PEG-CS microspheres) within 24 h with a CTC50 value above 1000 µg/mL. Incubation with 5-FU-loaded CAP-coated PEG-CS microspheres showed a decline in cell viability () that was less than that observed with 5-FU immediate release (IR) formulations suspended in SCMC, due to a reduction in cell viability to ∼88 to 90% after 24 h incubation ().
Figure 3. (A) Cytotoxicity of 5-FU immediate release (IR) formulations suspended in SCMC on HT-29 cell lines by MTT assay. Cytotoxicity of blank and 5-FU-loaded CAP-coated PEG-CS microspheres (B).
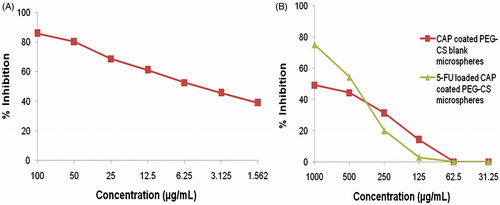
The results indicated that 5-FU concentration was sustained in the aliquots obtained after dissolution at certain time points and thus, cytotoxicity of the microspheres was maintained from 79 to 81% in the case of 80% (v/v) dissolution aliquots and 20.7 to 43.4% in case of 50% (v/v) dissolution aliquots as shown in . The CTC50 value of the aliquots ranged from 55 to 80% v/v.
Pharmacokinetics
Serum 5-FU concentration versus time profiles for the 5-FU immediate release (IR) formulations suspended in SCMC and CAP-coated PEG-CS microspheres are shown in . Pharmacokinetic parameters are shown in . The two formulations produced markedly different serum drug concentration profiles. There was no lag-time for both 5-FU immediate release (IR) formulations suspended in SCMC as well as CAP-coated PEG-CS microspheres. The mean peak plasma concentration (Cmax) was markedly different of the two formulations: 90.66 µg mL−1 versus 8.08 µg mL−1 for the 5-FU immediate release (IR) formulations suspended in SCMC and colon-specific microspheres, respectively. The mean residence time (MRT) was 1.37 h for the 5-FU immediate release (IR) formulations suspended in SCMC. The area-under-plasma-concentration-time curve (AUC) was 160.85 µg h mL−1 for the 5-FU immediate release (IR) formulations suspended in SCMC that is almost close to that of colon-specific microspheres (155.77 µg h mL−1). All the differences in pharmacokinetic parameters (Cmax, Tmax, and AUC) for the two formulations were significant (p < 0.001).
Figure 5. Serum 5-FU concentration versus time profiles of the 5-FU immediate release (IR) formulations suspended in SCMC and 5-FU-loaded CAP-coated PEG-CS microspheres.
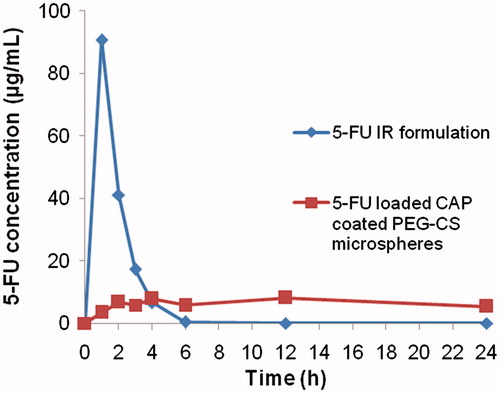
Table 1. Pharmacokinetic parameters of 5-FU after administration of CAP-coated PEG-CS microspheres and IR formulation in rats.
Distribution
Distribution of 5 FU to the stomach and small intestine after administration of 5-FU-loaded CAP-coated PEG-CS microspheres and the 5-FU immediate release (IR) formulations suspended in SCMC are shown in , respectively. Results indicate the total amount in the upper part of the GI tract. The mean peak concentration of 5-FU in the stomach and small intestine tissues from the 5-FU immediate release (IR) formulations suspended in SCMC were 374.37 ± 87.65 µg. g−1 at 1 h and 20.41 ± 1.52 µg. g−1 at 2 h.
Figure 6. Concentration of 5-FU in (A) stomach tissue homogenate, (B) intestinal tissue homogenate, (C) caecum content homogenate, (D) caecum tissue homogenate, (E) colon content homogenate, and (F) colon tissue homogenate after administration of the 5-FU immediate release (IR) formulations suspended in SCMC and 5-FU loaded CAP coated PEG-CS microspheres.
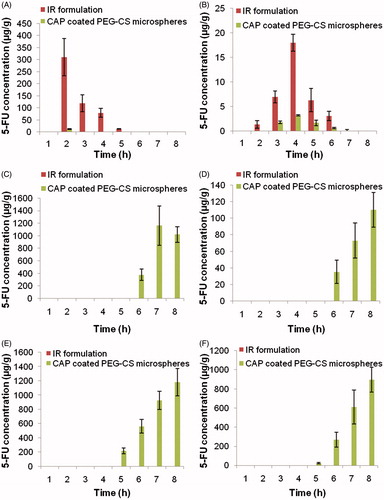
Distribution of 5-FU to caecal contents, caecum tissues, colon contents, and colon tissues after administration of the 5-FU immediate release (IR) formulations suspended in SCMC and colon specific microsphere is shown in . Significant differences in 5-FU concentration in colon tissues were observed after administration of both the two formulations (p < 0.001). The mean peak 5-FU concentration in caecal contents, caecum tissues, colon content, and colon tissues were 976.74 ± 147.71 µg. g−1, 110.21 ± 17.64 µg. g−1, 1055.34 ± 121.55 µg. g−1, and 1259.66 ± 219.22 µg. g−1, respectively, for the 5-FU immediate release (IR) formulations suspended in SCMC. However, a higher 5-FU concentration was achieved with CAP coated PEG-CS microspheres, but 5-FU was still detectable in the caecum and colon after 24 h in case of CAP coated PEG-CS microspheres. The 5-FU concentration in caecal contents, caecum tissues, colon content, and colon tissues 24 h after the administration of microspheres were 9.91 ± 1.12 µg. g−1, 46.79 ± 4.88 µg. g−1, 171.22 ± 3.27 µg. g−1, and 873.23 ±119.18 µg. g−1, respectively, whereas 5-FU was not detectable after 24 h in the case of the 5-FU immediate release (IR) formulations suspended in SCMC.
Pharmacodynamic studies
Body weight
Most of the rats (one animal died each in Groups I, II and III, respectively) survived after dosing of carcinogen in Groups I–IV. By the end of the 10th week, there was a significant difference in the average body weight between animals treated with DMH and those in the control group (results not shown, but p < 0.0001). Similarity, there were significant differences in the average weights () of animals after 14 weeks between Group I and Group IV (p < 0.001).
Table 2. Macroscopic findings and histopathology in rats after treatment with DMH (to induce colon cancer) and 5-FU formulations. Values are mean ± SEM.
Tumor incidence, distribution, volume, and multiplicity
Microscopic findings are summarized in , wherein tumor incidence is 100% in the group treated with the 5-FU immediate release (IR) formulations suspended in SCMC. A non-significant decrease in the number of tumors was observed in groups treated with CAP-coated PEG-CS microspheres. Tumors were distributed predominantly in proximal and middle colon. Tumor multiplicity was decreased in Group III compared with Group II, but it was not significant. A significant reduction in tumor volume was achieved after treatment with 5-FU-loaded CAP-coated PEG-CS microspheres (Group III) compared with Group I (tumor-induced group). There was a non-significant reduction in tumor volume by 5-FU-loaded CAP-coated PEG-CS microspheres compared with the 5-FU immediate release (IR) formulations suspended in SCMC.
Tumor histopathology
Animals in Group I treated with DMH showed development of well differentiated non-invasive adenocarcinoma restricted to mucosa (). The tumor tissues were composed of glands lined by cells with large, hyperchromatic nuclei (). Some glands showed obstruction of the lumen. Lymphocytic infiltration was minimal and limited to occasional cluster only. Rats of Group III, treated with the 5-FU immediate release (IR) formulations suspended in SCMC, showed minimal adenocarcinoma, but those treated with the colon-specific microspheres showed well-differentiated adenocarcinoma that was restricted to submucosa (). There was, however, a significant degree of lymphocytic infiltration within and around the malignant glands in the mucosa.
Figure 7. Morphological characteristics of colorectal cancer at different stages of different groups: (1A) and (1B) are DMH-induced groups. (2) The 5-FU immediate release (IR) formulations suspended in SCMC and (3) 5-FU-loaded CAP-coated PEG-CS microspheres-treated group.
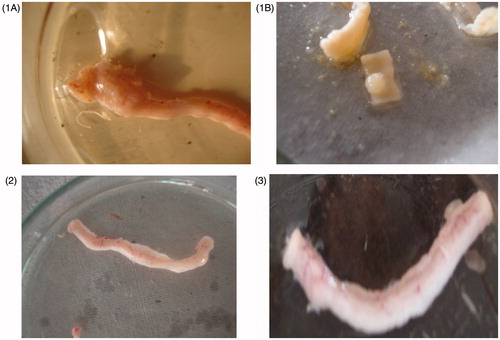
Figure 8. Aberrant crypt foci observed in colonic dysplasia in different groups: (1A and 1B) DMH-induced group, (2A and 2B) 5-FU standard treatment group, (3A and 3B) 5-FU-loaded CAP-coated PEG-CS microspheres-treated group. Numbers of aberrant crypt foci are high in DMH-induced group (1A and 1B) and markedly low in treated groups.
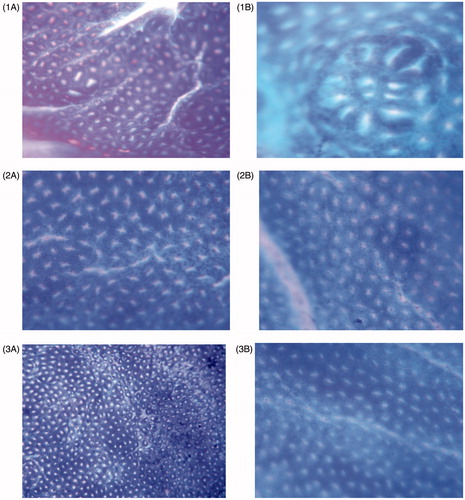
Figure 9. Proliferation activity carried out on colon tissues of different groups: (1A and 1B) DMH-induced group, (2A and 2B) 5-FU immediate release (IR) formulations suspended in SCMC, (3A and 3B) 5-FU-loaded CAP-coated PEG-CS microspheres. Portions marked in circles shows proliferation. The proliferation is high in DMH-induced group (1A and 1B) and is markedly low in treated groups.
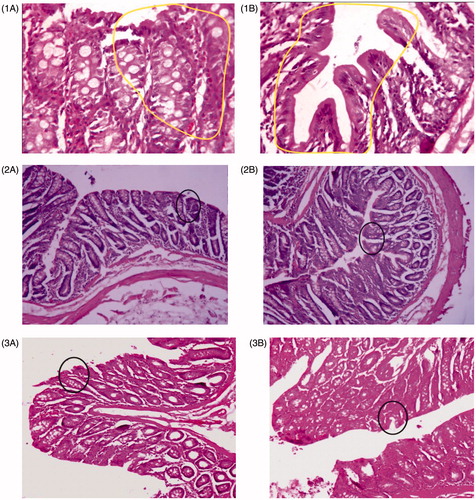
Biochemical parameters
Biochemical parameters are shown in . After 4 weeks of treatment with 5-FU formulations in carcinogen-treated animals, significantly higher serum levels of SGOT, SGPT, and alkaline phosphatase were observed in Group II compared with Group III (p < 0.001). Significantly high serum creatinine levels were observed in treatment Group II, clearly indicating the systemic toxic effects precipitated due to the standard 5-FU treatment. Similarly, significant differences in WBC count, serum albumin, and serum creatinine concentration were observed between the two groups of animals (p < 0.001).
Table 3. Biochemical parameters in rats treated with immediate-release 5-FU and CAP-coated PEG-CS microspheres. Data are mean ± SEM (n = 3).
Determination of aberrant crypt foci (ACF)
A significant decrease (p < 0.01) was observed in the number and the type of ACFs ( and ) observed in both 5-FU standard-treated group and 5-FU microsphere-treated group. The reduction in the number of ACFs clearly indicates the decrease the severity of dysplasia in Group II and III, respectively. The antiproliferative study () clearly indicates that proliferation of neoplastic cells was higher in Group I and was minimized in Group II and III.
Table 4. Number of aberrant crypt foci observed in induced control and treatment groups.
In vivo X-ray radiography
The series of results of roentgenographic study are shown in . is the X-ray image of the mice stomach just after administration of microspheres, while shows intactness of the microsphere after 1 h in the stomach. exhibits no significant difference in the integrity of microspheres in comparison with thereby indicating intactness of the coated microspheres on reaching the small intestine and its intactness was retained after 4th and 6th h of administration (), i.e. throughout the small intestine. Reduction in size of microspheres was seen in indicating erosion of the matrix in colon and the intact microspheres cannot be evidenced. These images clearly demonstrated the efficiency of the selected formulation for site specific targeting of the CAP coated PEG-CS microspheres to colon.
Discussion
The uncross-linked microspheres (data not shown) showed a drug release for around 5–6 h and then the 5-FU concentration declined. The PEG-cross-linked microspheres showed drug release up to 24 h. Thus, the enteric-coated PEG-cross-linked microspheres provided prolonged release as compared with the uncross-linked microspheres due to higher cross-linking density of the matrices. The microspheres showed an immediate burst release followed by controlled release which may be attributed to the drug crystals present on microsphere surface followed by controlled 5-FU release from the matrix. The pH sensitive CAP coating prevented the 5-FU release in the acidic media of stomach from coated PEG-CS microspheres and delivered the 5-FU to the colon. On one hand, the 5-FU immediate release (IR) formulations suspended in SCMC showed an immediate cytotoxic effect as evident by a rapid reduction in viability of HT-29 cells to ∼78 and ∼84% after 24 h incubation. On the other hand, lesser reductions in cell viability of ∼21 and ∼44% for 24 h were observed at a lower 5-FU concentration. The well-known higher cytotoxicity of pristine 5-FU was proved at varying concentration ranges as shown in the results but, 5-FU microspheres alone resulted in a reduction of cell viability that was less than both the previously described lower and higher concentrations of 5-FU immediate release (IR) formulations suspended in SCMC, but for a prolonged duration of time, thereby maintaining the cytotoxic effect. As per the literature, based on the report by Atjanasuppat et al., the antiproliferative/cytotoxic activities of any material were categorized according to the median inhibitory concentration (IC50) into four groups: ≤20 μg/mL, active; >20–100 μg/mL, moderately active; >100–1000 μg/mL, weakly active; and >1000 μg/mL, inactive (Atjanasuppat et al., Citation2009). In the case of cytotoxicty of blank CAP-coated PEG-CS microspheres, it is observed that IC50 value is observed above 1000 µg/mL concentration. The blank CAP-coated PEG-CS microspheres is non-toxic (IC50 > 1000 µg/mL) as per classification given for compounds by Atjanasuppat et al. at test doses against HT 29 cell lines. In short-term toxicity studies, blank CAP-coated PEG-CS microspheres do not show any toxicity even at 500 μg/mL and 1000 μg/mL, the highest concentration tested. It was clearly observed that the IC50 value of blank CAP-coated PEG-CS microspheres was more than 1000 μg/mL. At the highest concentration tested (1000 μg/mL), some cellular/CAP debris are observed, which may interfere with the results.
The 5-FU immediate release (IR) formulations suspended in SCMC showed immediate cytotoxic effect. The 5-FU-loaded CAP-coated PEG-CS microspheres showed a prolonged cytotoxic effect till the degradation of the polymer matrices. The effect of excipients was nullified by taking aliquots at different dissolution time intervals and the cytotoxic effect was measured.
The usual dose of 5-FU required for the average-risk patient suffering from CRC in good nutritional status with adequate hematopoietic function is 12 mg/kg iv once daily for 4 consecutive days. If no visible toxicity is observed, then the dose is minimized to 6 mg/kg on 6, 8, 10, and 12 d after which treatment is stopped. The maintenance therapy consists of a single dose iv administration at 10–15 mg/kg per week by either repeating the first course every 30 d after the last day of the previous course of the therapy or when the adverse effects as a result of the initial course of therapy, subside. In any case, there is a requirement of 9–45 courses of therapy over 12–60 months (Chabner et al., Citation1996).
The daily iv dose of 5-FU is fixed at 500 mg/m2 of body surface area. According to this dosage regimen, an adult dose of 5-FU to be administered through iv route is 600 mg (mean body surface area = 1.2 m2), but a dose of 5-FU to be administered specifically to human colon would be only 100 mg (mean colon surface area = 0.20 m2) (Sandle, Citation1998). Hence, targeted delivery of 5-FU to colon reduces the dose and, therefore, side effects are diminished. CAP-coated PEG-CS microspheres were able to prevent the 5-FU release in the stomach to prolong the drug release in colon, thereby reducing the systemic side effects as well as myelosuppressive effects associated with 5-FU therapy.
DMH-induced colon carcinogenesis model in Wistar rats closely mimics the pathophysiological resemblance in terms of disease presentation and gross as well as microscopic pathology of the human disease (LaMont & O’Gorman, Citation1978). DMH, a procarcinogen, forms reactive electrophilic carcinogens by means of oxidative metabolic activation in the liver. Azoxymethane, a known colon carcinogen, is produced due to DMH metabolism in the liver, which ultimately leads to the generation of methyl diazonium ion and carbonium ion, an active carcinogenic electrophile, which produces colonic neoplasia (Fiala, Citation1977; Fiala et al., Citation1987)
Absorption was delayed in the case of microspheres that showed slightly longer Tmax of around 12 h, indicating that CAP coating effectively prevented the drug release in the upper GI tract. The 5-FU-loaded CAP-coated PEG-CS microspheres showed the release at > 24 h, which indicates slow degradation of the microspheres. The Cmax was higher in the case of the 5-FU immediate release (IR) formulations suspended in SCMC due to rapid absorption in the upper part of GIT, which has a large surface area available for absorption. Interestingly, AUC was almost comparable with microsphere formulation accounting for ∼97% relative bioavailability. The lower bioavailability of 5-FU from the 5-FU immediate release (IR) formulations suspended in SCMC may be due to pH-dependent solubility of 5-FU, which precipitates in acidic pH of the stomach. The 5-FU immediate release (IR) formulations suspended in SCMC after oral administration led to precipitation of the drug in the stomach and consequently the overall amount of 5-FU absorbed was reduced (Zinutti et al., Citation1998).
The 5-FU-loaded CAP-coated PEG-CS microspheres could sustain the release of 5-FU and thereby, maintained the therapeutic level without significant increase in systemically available 5-FU for interaction with non-tumor cells, thus increasing the safety and efficacy index of the microspheres. MRT of the microspheres could not be estimated as the release of 5-FU continued even after 24 h, which indicated that drug was showing a slow and steady absorption profile from colonic tissue and thereby, exerting its cytotoxic effect on colonic dysplasia. These results are in agreement with the previous studies (Zinutti et al., Citation1998; Rahman et al., Citation2008; Wei et al., Citation2008). The CTC50 value for 5-FU is the range of 15–7770 μM with a median value of 390 μM for various cancers (Fischel et al., Citation1995) and from 5.5 to 160 μM in resistant colon cancer cell-lines (Copur et al., Citation1995).
The tissue or organ concentration of 5-FU is an indicator of its specificity, efficacy, and pharmacological response. The GI distribution studies of 5-FU showed high concentration of 5-FU in colon for the enteric-coated microsphere formulation in comparison with the 5-FU immediate release (IR) formulations suspended in SCMC. The concentration achieved at 6, 12, and 24 h after administration of the microspheres was 270 ± 78, 611 ± 177, and 895 ± 131 µg. g−1, respectively. This concentration was well above the CTC50 of 5-FU required for resistant colon cancer cell-lines such as HT-29, indicating that little drug was released in the non-target site and the concentration of 5-FU was achieved to arrest the tumor growth.
A reduction in tumor size and survival of the rats determine the safety and the efficacy of the microspheres. The tumor volume and multiplicity decreased after 4 weeks treatment with microspheres, which were less compared with the 5-FU immediate release (IR) formulations suspended in SCMC. The accurate prognostic information about the colonic dysplasias was determined by Duke’s staging. The animals remained in Duke’s stage A even after 4 weeks of treatment with 5-FU formulations. Animals treated with microspheres showed marked lymphocytic infiltration in and around the tumor cells, which indicated that body was responding to 5-FU therapy. Moreover, there was a significant weight loss in case of the 5-FU immediate release (IR) formulations suspended in SCMC, which led to lower survival rate in comparison with the microsphere formulation and thus, the drug was delivered to the target site with minimal exposure to systemic circulation.
Severe digestive, hematological, and mucosal damages occur due to 5-FU therapy. The 5-FU basically undergoes anabolism and catabolism in the liver. The biochemical parameters were not drastically altered in the case of microspheres compared with the 5-FU immediate release (IR) formulations suspended in SCMC. SGOT, SGPT, and alkaline phosphatase, indicators of liver function were less affected in the case of microspheres-treated animals. There was a significant loss in body weight, food intake, and diarrhea in animals treated with the 5-FU immediate release (IR) formulations suspended in SCMC of 5-FU, which may be ascribed to mucositis of the GI tract due to systemic exposure. Leucocytopenia and thrombocytopenia were significant in animals treated with the 5-FU immediate release (IR) formulations suspended in SCMC in comparison with microspheres. Serum albumin and creatinine were markedly increased in the case of animals treated with the 5-FU immediate release (IR) formulations suspended in SCMC. The systemic exposure of 5-FU immediate release (IR) formulations suspended in SCMC led to the elevated level of these enzymes and biochemical parameters in animals. These changes were less severe in animals treated with microsphere formulation. Thus, the novel microsphere formulation showed colonic delivery with low systemic toxicity. The morphological and histopathological changes were evident, but insignificant, thus showing that chronic dosing studies are required to explore the potential of this formulation for oral delivery of 5-FU for the treatment of CRC.
Conclusion
In colon cancer therapy, 5-FU is the choice of drug for clinical therapeutics although it has severe systemic side effects. In efforts to improve therapeutic efficacy and safety, a novel pH-sensitive micro-delivery device for localized delivery of 5-FU to colon has been developed using CAP-coated PEG-cross-linked chitosan microspheres that were evaluated for in vitro and in vivo efficacies. The in vitro study indicated the prolongation of cytotoxic effect of 5-FU encapsulated in the microspheres. The pharmacokinetic study indicated increased bioavailability, while the GI distribution indicated localized 5-FU concentration in the colon that was higher than CTC50 of 5-FU for colon cancer cell-lines. Biochemical parameters did not change drastically after treatment with microspheres, but a significant reduction in tumor volume was observed. The novel 5-FU-loaded microspheres of this study are proven to be the promising oral delivery devices for the treatment of CRC. Further clinical studies in humans are necessary to establish the safety and therapeutic efficacy of the formulations.
Acknowledgements
Authors duly acknowledge the help of Dr. S. D. Kholkute and RMRC, Belgaum, in this study.
Declaration of interest
The authors report that they have no conflicts of interest. Mr. Kuntal Ganguly thanks for the financial support from the Council of Scientific and Industrial Research (CSIR) [Grant no. 08/558(0001)/2010-EMRI], New Delhi, India, for providing a research fellowship. Professor T. M. Aminabhavi acknowledges the All India Council for Technical Education (AICTE), New Delhi, India [Grant no. AICTE. F.No. 1-51/RIFD/EF(13)/2011-12] for Emeritus Fellowship. Authors also thank Vision Group of Science and Technology for K-FIST grant for the study.
References
- Assaad E, Wang YJ, Zhu XX, Mateescu MA. (2011). Polyelectrolyte complex of carboxymethyl starch and chitosan as drug carrier for oral administration. Carbohydr Polym 84:1399
- Atjanasuppat K, Wongkham W, Meepowpan P, et al. (2009). In vitro screening for anthelmintic and antitumour activity of ethnomedicinal plants from Thailand. J Ethnopharmacol 123:475–82
- Bird RP. (1987). Observation and quantification of aberrant crypts in the murine colon treated with a colon carcinogen: preliminary findings. Cancer Lett 37:147–51
- Bowers LD. (1980). Kinetic plasma creatinine assays I. The role of various factors in determining specificity. Clin Chem 26:551–4
- Bradley DW, Maynard JE, Emery G, Webster H. (1972). Transaminase activities in plasma of long-term hemodialysis patients. Clin Chem 18:1442
- Chabner BA, Allegra CJ, Curt GA, Calabresi P. (1996). Anti-neoplastic agents. In: Hardman JG, Limbird LE, Berry PM, Raymond WR, eds. Goodman and Gilman’s the pharmacological basis of therapeutics. New Delhi: McGraw-Hil, p. 1233–87
- Chourasia MK, Jain SK. (2004a). Polysaccharides for colon targeted drug delivery. Drug Deliv 11:129–48
- Chourasia MK, Jain SK. (2004b). Design and development of multiparticulate system for targeted drug delivery to colon. Drug Deliv 11:201–7
- Copur S, Aiba K, Drake JC, et al. (1995). Thymidylate synthase gene amplification in human colon cancer cell lines resistant to 5-fluorouracil. Biochem Pharmacol 49:1419–26
- Djordjeuic B, Lange CS, Allison RR, Rotman M. (1993). Response of primary colon cancer cells in hybrid spheroids to 5-fluorouracil. Cancer Invest 11:291–3
- Doumas BT, Byase DD, Carter RJ, et al. (1981). A candidate reference method for determination of total protein in plasma. I. Development and validation. Clin Chem 27/10:1642–50
- Du J, Dai J, Liu J, Dankovich T. (2006). Novel pH-sensitive polyelectrolyte carboxymethyl Konjac glucomannan-chitosan beads as drug carriers. React Funct Polym 66:1055
- Fiala ES. (1977). Investigation into metabolism and mode of action of the colon carcinogen 1,2-dimethylhydrazine and azoxymethane. Cancer 40:2436–45
- Fiala ES, Sohn OS, Hamilton SR. (1987). Effects of chronic dietary ethanol on the in vivo and in vitro metabolism of methylazoxymethanol and on methylazoxymethanol induced DNA methylation in the rat colon and liver. Cancer Res 47:5939–43
- Fischel JL, Etienne MC, Spector T, et al. (1995). Dihydropyrimidine dehydrogenase: a tumoral target for fluorouracil modulation. Clin Cancer Res 1:991–6
- Freshney I. (1994). Culture of animal cells. New York: Wiley-Liss
- Ganguly K, Aminabhavi TM, Kulkarni AR. (2011). Colon targeting of 5-fluorouracil using polyethylene glycol cross-linked chitosan microspheres enteric coated with cellulose acetate phthalate. Ind Eng Chem Res 50:11797–807
- Ghorab DM, Amin MM, Khowessah OM, Tadros MI. (2011). Colon-targeted celecoxib-loaded Eudragit® S100-coated poly-ε-caprolactone microparticles: preparation, characterization and in vivo evaluation in rats. Drug Deliv 18:523–35
- Herrmann R. (1993). Systemic treatment of colorectal cancer. Eur J Cancer 29A:583–6
- Ishikawa T, Utoh M, Sawada N, et al. (1998). Tumor selective delivery of 5-fluorouracil by capecitabine, a new oral fluoropyrimidine carbamate, in human cancer xenografts. Biochem Pharmacol 55:1091–7
- Jemal A, Bray F, Center MM, et al. (2011). Global cancer statistics. CA Cancer J Clin 61:69–90
- Kaur G, Jain S, Tiwary AK. (2010). Chitosan-carboxymethyl tamarind kernel powder interpolymer complexation: investigations for colon drug delivery. Sci Pharm 78:57
- Kulkarni AR, Hukkeri VI, Sung HW, Liang HF. (2005). A novel method for the synthesis of the PEG-crosslinked chitosan with a pH independent swelling behavior. Macromol Biosci 5:925
- Labianca RF, Beretta GD, Pessi MA. (2001). Colorectal cancer: disease management considerations. Drugs 61:1751–64
- LaMont JT, O’Gorman TA. (1978). Experimental colon cancer. Gastroenterology 75:1157–69
- Li W, Li CB. (2003). Lack of inhibitory effect of lactic acid bacteria on 1,2-dimethylhydrazine-induced colon tumors in rat. World J Gastroenterol 9:2469–73
- Li P, Wang Y, Zeng F, et al. (2011). Synthesis and characterization of folate conjugated chitosan and cellular uptake of its nanoparticles in HT-29 cells. Carbohydr Res 346:801
- Liu Z, Jiao Y, Liu F, Zhang Z. (2007). Heparin/chitosan nanoparticle carriers prepared by polyelectrolyte complexation. J Biomed Mater Res Part A 83:806
- Meropol NJ. (1998). Oral fluoropyrimidines in the treatment of colorectal cancer. Eur J Cancer 34:1509–13
- Moss DW, Henderson AR. (1999). Clinical enzymology. In: Burtis CA, Ashwood ER, eds. Tietz textbook of clinical chemistry. Philadelphia: WB Saunders Co, p. 652
- Mundargi RC, Patil SA, Agnihotri SA, Aminabhavi TM. (2007). Development of polysaccharide-based colon targeted drug delivery systems for the treatment of amoebiasis. Drug Dev Ind Pharm 33:255–64
- Preezel NC, Webling DDA. (1971). The length and mucosal surface area of the small and large gut in young rat. J Anat 108:295–6
- Rahman Z, Kohli K, Zhang SQ, et al. (2008). In-vivo evaluation in rats of colon-specific microspheres containing 5-fluorouracil. J Pharm Pharmacol 60:615–23
- Rai G, Yadav AK, Jain NK, Agarwal GP. (2014). Eudragit-coated dextran microspheres of 5-fluorouracil for site-specific delivery to colon. Drug Deliv. [Epub ahead of print]. DOI: 10.3109/10717544.2014.913733
- Ramasamy T, Ruttala HB, Shanmugam S, Umadevi SK. (2013). Eudragit-coated aceclofenac-loaded pectin microspheres in chronopharmacological treatment of rheumatoid arthritis. Drug Deliv 20:65–77
- Routledge ED, Gorman S, Clark MR. (1994). Cell and tissue culture: laboratory procedures. New York: Wiley
- Sandle GI. (1998). Salt and water absorption in the human colon: a modern appraisal. Gut 43:294–9
- Sareen R, Jain N, Rajkumari A, Dhar KL. (2014). pH triggered delivery of curcumin from Eudragit-coated chitosan microspheres for inflammatory bowel disease: characterization and pharmacodynamic evaluation. Drug Deliv. [Epub ahead of print]. DOI: 10.3109/10717544.2014.903534
- Torchillin VP. (2007). Targeted pharmaceutical nanocarriers for cancer therapy and imaging. AAPS J 9 :E128–47
- Torchillin VP. (1998). Polymer-coated long-circulating microparticulate pharmaceuticals. J Microencapsul 15:1–19
- Wei H, Qing D, De-Ying C, et al. (2008). Study on colon-specific pectin/ethylcellulose film-coated 5-fluorouracil pellets in rats. Int J Pharm 348:35–45
- Yan C, Gu J, Guo Y, Chen D. (2010). In vivo biodistribution for tumor targeting of 5-fluorouracil (5-FU) loaded N-succinyl-chitosan (Suc-Chi) nanoparticles. Yakugaku Zasshi 130:801–4
- Yang L, Chu JS, Fix JA. (2002). Colon-specific drug delivery: new approaches and in vitro/in vivo evaluation. Int J Pharm 235:1–15
- Yoon SS, Eto S, Lin CM, et al. (1999). Mouse endostatin inhibits the formation of lung and liver metastasis. Cancer Res 59:6251–6
- Zeng A, Dong K, Wang M, et al. (2015). Investigation of the colon-targeting, improvement on the side-effects and therapy on the experimental colitis in mouse of a resin microcapsule loading dexamethasone sodium phosphate. Drug Deliv. [Epub ahead of print]. DOI: 10.3109/10717544.2015.1046569
- Zhang DY, Shen XZ, Wang JY, et al. (2008). Preparation of chitosan-polyaspartic acid-5-fluorouracil nanoparticles and its anti-carcinoma effect on tumor growth in nude mice. World J Gastroenterol 14:3554
- Zinutti C, Barberi-Heyob M, Hoffman M, Maincent P. (1998). In-vivo evaluation of sustained release microspheres of 5-FU in rabbits. Int J Pharm 166:231–4