Abstract
Enteric coating of microparticles prevents stomach degradation and enhances oral bioavailability of poorly soluble drugs. Thus, in the present study, enteric-coated microparticle (ECMP) of biochanin A was developed and their pharmacological potential was investigated in hypertensive ovariectomized rats (Ovx-HT). Biochanin A microparticles were prepared by using ionic gelation method and coating was done by ethyl cellulose using coacervation phase separation method. Surface modified microparticles were characterized on the basis of size, entrapment efficiency, polydispersity index, FTIR and zeta potential and percentage of in vitro release. Ovariectomized rats were administered deoxy corticosterone acetate (40 mg/kg, s.c. twice a week for 6 weeks) salt to induce Ovx-HT. Hypertension was assessed in terms of increase in systolic, diastolic, mean arterial blood pressure, lipid peroxidation level, tumor necrosis factor-alpha (TNF-α) level and decrease in serum nitrite and reduced glutathione (GSH) level. The optimized formulation of ECMP has shown significant increase in oral bioavailability assessed by high-performance liquid chromatography. Furthermore, these ECMPs significantly reduced the mean arterial blood pressure, systolic and diastolic blood pressure, reduced lipid peroxidation and TNF-α level and significantly increased the serum nitrite and reduced GSH level in Ovx-HT rats. However, L-NAME significantly prevented the ameliorative effect of ECMP. Thus, it may be concluded that ECMP of biochanin A has shown delayed release capacity, increase in oral bioavailability up to 6 folds than plain biochanin A and exerts antihypertensive effect in ovariectomized rats possibly in an eNOS dependent manner.
Introduction
Estrogen is an important vasoprotective molecule with marked actions on blood vessels. In hypertension allied with menopause estrogen deficiency is the critical factor. Phytoestrogen is polyphenolic non-steroidal plant compounds with estrogen like organic commotion. Biochanin A (BCA) is a phytoestrogen isolated from the Trifolium pratense (Fabeaceae) that exerts estrogenic action and causes vasodilation, and decrease in pro-inflammatory mediators. BCA is used for several purposes like estrogen deficiency, treatment of pain and/or reducing the severity of any nerve damage. BCA is BCS CLASS II drug due to its poor water solubility. Moreover, this drug is associated with certain drawbacks like poor oral bioavailability (4.6%) due to stomach degradation, extensive hepatic first-pass metabolism and susceptibility to hydrophilic degradation in gastrointestinal (GI) tract (Singh et al., Citation2011). Oral bioavailability can be enhanced by developing delivery system, which delivers drug directly into the intestine by using enteric-coated microparticles (ECMPs) (Chourasia & Jain, Citation2004). To overcome stomach degradation and to enhance oral bioavailability, intestinal lymphatic movement of the medicine can be exploited, which can be possibly done by using polymers as a drug carrier. There are different approaches used to improve the solubility and oral bioavailability of biochanin A, such as microemulsion, microbeads and microparticles, that improves aqueous solubility and dissolution rate of biochanin A. Microparticles are a type of drug delivery systems where the particle size ranges from 1 to 1000 µm in size. The drug is dissolved, entrapped, encapsulated or attached to microparticle matrix. For effective oral delivery of drug, various polymers have been used for enteric coating include cellulose acetate phthalate, cellulose acetate trimelliate, polyvinyl acetate phthalate and hydroxy methylcellulose phthalate, fatty acids, waxes, shellac, plastics, plant fibers, etc. (Singh et al., Citation2011). Recently, much emphasis is being laid on the development of multiparticulate dosage forms in comparison to single unit systems because of their potential advantages like increased bioavailability, diminution in the menace of systemic toxicity; local irritation and retention in the intestine for a relatively prolonged period of time. Furthermore, smaller particle size empowers these systems to pass through the GI tract easily, leading to decreased individual variability. Subsequently if administered in night, microparticles are predictable to sustain a low drug plasma concentration overnight (Cetin & Sahin, Citation2015) when the cardiovascular risks are reported to be the minimum and liberate the optimal concentrations in the morning between 6 and 12 h when the hypertensive risk is found to be the maximum (Patel et al., Citation2012).
Perez et al. (Citation2000) have prepared and evaluated “(ɛ-caprolactone) microparticles containing both lipophilic and a hydrophilic drug” and for oral delivery based on both in oil-in-water (o/w) or the water-in-oil-in-water (w/o/w) solvent evaporation method was developed. The encapsulation efficiency was 91% for nifedipine and 37% for propranolol HCl with the o/w-method, and 83% for nifedipine and 57% for propranolol HCl with the w/o/w-method. Patel & Dhake (Citation2012) have prepared and evaluated “colon specific microspheres for chronotherapy of hypertension” by using emulsion cross-linking method. In that study, they found that the entrapment efficiency of microencapsulated formulations varied between 90% and 95% with increasing core–coat ratio. Lorenzo-Lamosa et al. (Citation1998) have prepared and evaluated “Design of microencapsulated chitosan microspheres for colonic drug delivery” by using sodium diclofenac, as a model drug, that was efficiently entrapped within CS microcores using spray-drying and further microencapsulated into Eudragit® L-100 and Eudragit S-100 using an oil-in-oil solvent evaporation method. In that study, they found that perfect pH-dependent kinetics profiles were attained by coating the CS microcores with Eudragit. No release was observed at acidic pH; however, a constant discharge of diclofenac was observed for a variable time (8–12 h) when reaching the Eudragit pH solubility. Patel et al. (Citation2012) have prepared and evaluated “Propranolol Hydrochloride-Loaded Carbopol-934 P/Ethyl Cellulose Mucoadhesive Microspheres” using emulsion-solvent evaporation technique. The best batch exhibited a high drug entrapment efficiency of 54%; 82% mucoadhesion after 1 h and particle size of 110 μm.
The aim of the present study was to develop oral sustained release dosage form of biochanin A with improved oral bioavailability by preventing stomach degradation and to investigate their pharmacological potential and mechanism of action in deoxy corticosterone acetate (DOCA) salt induced hypertension in ovariectomized rats.
Material and methods
Drugs and chemicals
BCA was purchased from Sigma Aldrich (New Delhi, India). L-NAME was purchased from Cayman Chemicals (New Delhi, India), and lisinopril was purchased from Avanscure Life sciences Pvt. Ltd., Gurgaon, India. Other chemicals and biochemical reagents were of analytical grade.
Preparation of ECMPs
Preparation of biochanin A-loaded pectin microparticles
BCA microparticles (BAMP) were prepared by ionotropic external gelation technique reported by Atyab et al. (Citation2005). First, different concentrations, like 1–6% w/v of pectin, were dispersed in a specified volume of cold water containing the drug and allowed to swell for 2 h. In another beaker, 2% w/v of sodium alginate was taken and mixed well with 10 ml of water. The pectin solution containing the drug was added to sodium alginate solution with stirring at 400 rpm for 15–20 min to produce a viscous form. Then, polymer drug solution was added dropwise by using syringe of 22 G having needle of 0.45 mm inner diameter from a height of about 5 cm into a beaker containing 6% w/v solution of calcium chloride with continuous stirring by magnetic stirrer. The gelatinous precipitate is formed by chemical reaction between sodium alginate and calcium chloride. The prepared beads were left under stirring in the medium at 1000 rpm for 20 min, removed by centrifugation at 5000 rpm (REMI CENTRIFUGE R8C, Mumbai, India) washed with distilled water. After that BAMP were vacuum dried and stored in well closed container for further use (Atyab et al., Citation2005).
Preparation of ethyl cellulose-coated pectin microparticles (ECMP) of biochanin A
BCA-loaded (BAMP) pectin microparticle was used as a core material for the preparation of double-coated system reported by Paharia et al. (Citation2007). A coacervation phase separation method was applied for this step. Known amount of the microparticles was dispersed in ethyl acetate (25 ml) solution containing ethyl cellulose and containing 0.02% w/v span 80. This mixture was agitated for 5 min at 400 rpm. Subsequently, 50 ml n-hexane (as the non-solvent) was poured into the polymeric solution containing the core material with the rate of 1 ml/min and core:coat ratio is 1:3. The medium was stirred for 60 min to complete the process of microparticle coating. Coated microparticles were then washed with an excess of n-hexane, filtered and dried at room temperature.
Optimization of ECMPs
Optimization of biochanin A microparticles was done by optimizing the parameters including stirring speed, concentration of polymer (pectin) for obtaining desired particle size, polydispersity index (PDI) and entrapment efficiency of microparticles.
Characterization of ECMPs
Shape and size
The shape and size of the ethyl cellulose-coated pectin microparticles were measured using Motic microscopy (New Delhi, India) (Wanjari & Gaikwad, Citation2012).
Determination of entrapment efficiency
Entrapment of biochanin A was determined by measuring the concentration of free drug. Enteric-coated microparticles were centrifuged at 5000 rpm for 20 min, then the supernatant was measured for the unentrapped drug in it with help of UV spectrophotometer at 262 nm.
Measurement of particle size and zeta potential
The mean particle size, PDI and zeta potential of the ECMPs of biochanin A were determined in purified water at 23 °C by zetasizer (Beckman Coulter Counter, New Delhi, India). Particle size distribution studies were performed at a fixed refractive index of the respective formulation and size was measured in triplicate.
Pharmacokinetic studies of ECMP of biochanin A
In vitro drug release
In vitro release study of ECMP was carried out using dialysis bag method. For this study, ECMP equivalent to 5 mg of biochanin A were measured and added to dialysis membrane-70 (Himedia, LA393-10MT, Mumbai, India). This dialysis membrane was taken into 50 ml of simulated gastric fluid of pH 1.2 in a conical flask for 2 h. Then, this buffer was replaced with fresh simulated intestinal buffer of pH 6.8 for small intestine. Further flask was taken in a incubator shaker and speed of shaker was maintained at 60 rpm at 37 ± 0.5 °C. At specific time intervals, samples (1 ml) were withdrawn and filtered. Same volume (1 ml) of the phosphate buffer pH 6.8 was replaced after each sampling. The drug content in the sample was determined spectrophotometrically at 262 nm (Kumar et al., Citation2014).
Experimental animals
Young female Wistar rats (180–250 g), procured from the Central Animal House of I.S.F. College of Pharmacy, Moga, Punjab, India, were maintained at standard conditions of temperature (25 ± 2 °C) and humidity 55 ± 2%. The rats were fed on standard chow diet and were provided water ad libitum. The experiment protocol was approved by Institutional Animal Ethics Committee (ISFCP/IAEC/CPCSEA/Meeting no. 14/2015/PROTOCOL No. 255) and was carried out in accordance with the guidelines of the Indian National Science Academy (INSA) for the use and care of experimental animals.
Experimental protocol
Animals were divided into seven groups. Each group consists of six animals. Group I Sham Control, Group II Ovariectomized-DOCA-hypertensive (Ovx-HT) rats, Group III Ovx-HT rats + biochanin A (10 mg/kg, p.o., 1 week) (Harini et al., Citation2012), Group IV Ovx-HT rats + biochanin A (10 mg/kg, p.o., 1 week) + L-NAME (10 mg/kg, p.o., 1 week), Group V Ovx-HT rats + biochanin A ECMP (ECMP: 10 mg/kg., p.o., 1 week), Group VI Ovx-HT rats + ECMP (10 mg/kg, p.o., 1 week) + L-NAME (10 mg/kg, p.o., 1 week), Group VII Ovx-HT rats + Lisinopril (1 mg/kg, p.o., 1 week) (Wienen et al., Citation2001).
Ovx-surgery
Prior to the surgery, the weight of animals was measured by a digital weighing machine. The animals were anesthetized with ketamine (80 mg/kg, i.p.) and xylazine (50 mg/kg, i.p.). Ovariectomy was preceded by a midline dorsal skin incision, 3-cm long, approximately halfway between the middle of the back and the base of the tail. A small transverse peritoneal incision of 0.4–0.6 cm was made with surgical scalpel blade no. 11 on the middle part of the abdomen slightly toward right. After peritoneal cavity was assessed, the adipose tissue was pulled away until the right uterine tube and the ovary surrounded by a variable amount of fat were identified. The ovary and associated fat were easily located and exteriorized by gentle retraction (Tanaka et al., Citation1997). The procedure was repeated for the left ovary through the same incision. After identifying the ovary and uterine horn, a braided silk suture was performed around the area of the distal uterine horns, that was sectioned thereafter, and the ovaries were removed. The uterine horn was returned to the peritoneal cavity after the removal of ovaries. The wound was closed in two layers (muscle and skin) using sterile sutures. The peritoneum and the muscle layers were sutured with one absorbable suture. Povidone iodine was applied on the area to disinfect the skin after suturing. High degree of aseptic procedure was maintained throughout the operation (Khajuria et al., Citation2012).
Assessment of blood pressure
Assessment of systolic, diastolic and mean arterial blood pressure
The rat blood pressure was measured by the tail cuff method (NIBP-BIOPAC MP100, Goleta, CA). Rat tail was heated with exact time 3 min after that tail was inserted into the cuff, pressure was applied and systolic, diastolic and mean arterial blood pressures are recorded on BIOPAC].
Biochemical parameters
Reduced GSH
To measure the reduced GSH level, aortic tissue homogenate (in 0.1 M phosphate buffer pH 7.4) was taken. One milliliter of homogenate was mixed with 1 ml of 4% sulfosalicylic acid. This solution was kept for 1 h at 4 °C and centrifuged at 1200g for 15 min at 4 °C. After centrifugation 0.1 ml of supernatant was removed. Then in 0.1 ml of supernatant add 2.7 ml of phosphate buffer (pH 8.0) and 0.2 ml of 0.01 M DTNB (Ellman’s reagent) in 0.4% in phosphate buffer 0.1 M (pH 8.0). After completion of the total reaction, solutions were measured at 412 nm against blank. Absorbance values were compared with a standard curve generated from standard curve from known GSH. The level of GSH was expressed as µmol of GSH/mg of aortic wt (Ellman, Citation1959).
Serum nitrite/nitrate level using Greiss reagent
Blood samples from rats were collected from retro-orbital sinus and allowed to clot. The Eppendorff tubes were kept at 4 °C for 30 min and centrifuged at 1500g for 10 min to obtain serum. The accumulation of nitrite in the supernatant, an indicator of the production of nitrite oxide was determined by a colorimetric assay with Griess reagent (Reagent A – 0.1% N-(1-napthyl) ethylene diaminedihydrochloride, Reagent B – 1% sulfanilamide and 5% phosphoric acid. Both reagent A and reagent B were mixed in equal volume just before the experiment. In 800 µl of serum (sample), 1 ml of Griess reagent was added and the mixture was incubated for 5 min at room temperature. The absorbance was measured at 546 nm using Perkin Elmar Lambda 20 spectrophotometer. The concentration of the nitrite in the supernatant was determined from sodium nitrite standard curve and expressed as in µmol/l (Sastry et al., Citation2002).
Measurement of lipid peroxidation assay
Malondialdehyde (MDA) concentration was measured by estimating thiobarbituric acid reactive substances (TBARS). Concentration of TBARS was determined as index of lipid peroxidation. The extent of lipid peroxidation in the aortic homogenate was determined quantitatively by performing the method as described by Wills (Citation1966). Homogenate (0.5 ml) was added to 0.5 ml of Tris–HCl buffer (pH 7.4) and incubated for 2 h at 37 °C. Then, 1 ml of 10% ice-cold TCA was added to above solution. Furthermore, 1 ml of 0.67% TBA solution was added to 1 ml of supernatant following centrifugation at 1000 rpm for 10 min. The test tubes were kept in boiling water 10 min and cooled it, and 1 ml of double distilled water (DDW) was added. The amount of MDA was measured by reaction with thiobarbituric acid at 532 nm using Perkin Elmer Lambda 20 spectrophotometer (New Delhi, India). Values were calculated in nmol/mg protein (Pr).
Estimation of tumor necrosis factor-alpha levels
TNF-α level was estimated by using rat tumor necrosis factor-alpha (TNF-α) kit (Ray Bio (New Delhi, India), Rat TNF-α ELISA kit protocol), which uses a micropipette plate reader that read at 450 nm. Concentrations of TNF-α were calculated from standard curve (Krisgen Elisa kit of TNF-α determination).
HPLC method for determination of plasma drug concentration
Pharmacokinetic studies were carried out in female Wistar rats (180–250 g). The animals were divided into two groups (n = 6), one group was administered pure drug and other group received ECMPs with a dose of 10 mg/kg, p.o. The blood samples were collected at predetermined time intervals, into tubes with heparin for avoiding blood clotting. The collected blood samples were immediately centrifuged by cold centrifugation at 10 000 rpm for 5 min and the obtained plasma was stored at −70 °C until analysis. BCA plasma concentration was analyzed using reversed-phase high-performance liquid chromatographic (RP-HPLC) method as reported by Chen et al. (Citation2010). Briefly, the analyte was extracted from the plasma. C18 analytical column (250 mm× 4 mm i.d.) is used for HPLC separation with acetonitrile–water containing 0.5% phosphoric acid (40:60), as a mobile phase. Detection was performed at 262 nm. Calibration graphs were linear from 25 to 1000 ngml−1 biochanin A. Stock solutions with ethanol–water (50:50) containing 0.5% phosphoric acid were used as an internal standard.
Assessment of integrity of vascular endothelium
Histopathology
The histopathological study was performed as following:
Fixation: Aortic tissue was fixed in 2.5% glutraldehyde phosphate buffer.
Processing of tissues: Embedding of 3–4 mm aortic rings in paraffin is accomplished in the form of block.
Cutting: Cutting was done using microtome up to size of 5 µm.
Staining: Staining was performed with eosin and hematoxylin dye.
Photomicrography: It was done by observing tissue architecture under microscope in 10× and the microscope imaging was also done.
Statistical analysis
All values were expressed as mean ± SD. Various biochemical parameters, i.e. Lipid peroxidation, serum nitrite level, reduced GSH and Blood pressure measurements, i.e. systolic blood pressure, diastolic blood pressure, mean arterial pressure were statistically analyzed using one-way ANOVA followed by Tukey’s multiple comparison test. The p value of less than 0.05 was considered to be statistically significant.
Results
Measurement of particle size, zeta potential and infra red spectra (FTIR)
The mean particle size of formulation F7 was observed to be 3.36 µm and PDI 0.290. Furthermore, mean particle size of formulation F9 ECMP was observed to be 4.96 µm and PDI 0.25 ().
Figure 1. (a) Zetasizer and PDI of drug-loaded biochanin A microparticle (BAMP). (b) Zeta-sizer and PDI of ECMPs of biochanin A.
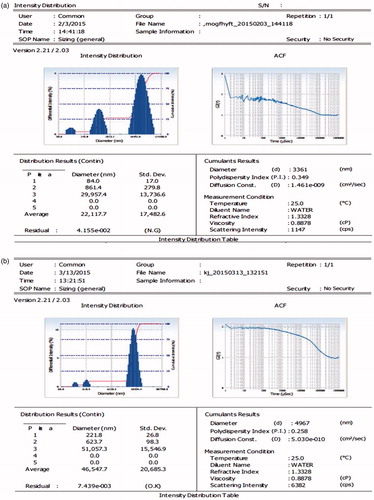
The FTIR of BAMP and ECMP was carried out by OMNIC Software and different peaks were observed. The phenolic OH stretch was observed at 3600–3200 cm−1, the stretching vibration of C = O was observed at 1716–1675 cm−1 and C–O stretch was observed at 1000–1300 cm−1 ( and and ).
Optimization and characterization of ECMPs of biochanin A
Many factors including composition, particle size and zeta potential may influence in vivo characteristics of microcarriers. Different formulations of variating concentrations and time were used for optimization of ECMP. Each formulation is prepared three times. The mean particle size of final optimized ECMP(F9) was 4.9 µm, with a zeta potential of approximately −1.8 mV. Entrapment efficiency of final formulation was found to be 94%. Details with regard to the pharmaceutical properties of the prepared EMPs are summarized in .
Table 1. Optimization of drug, BAMP and ECMP.
Table 2. FTIR frequency of observed peaks of biochanin A-loaded pectin microparticles (BAMP) and its interpretation.
Table 3. FTIR frequency of observed peaks of ethyl cellulose-coated pectin microparticles of biochanin A (ECMP) and its interpretation.
F1–F6 formulations (without drug) were optimized for % pectin, % sodium alginate, % calcium chloride. Formulation F5 has shown better results, i.e. less diameter size 3.092 ± 0.16 µm and low PDI 0.252. Furthermore, formulations F7 and F8 of biochanin A loaded microparticles, i.e. BAMP, were optimized for % pectin, % sodium alginate, % calcium chloride. Formulation F7 has shown less diameter size, i.e. 3.36 ± 0.17, low PDI 0.290 and 98.94% entrapment efficiency. Formulations F9 and F10 of ECMPs were optimized for % pectin, % sodium alginate, % calcium chloride. Formulation F9 has shown less diameter size, i.e. 4.94 ± 0.47 µm, lower PDI 0.25 and 94% entrapment efficiency ().
In vitro studies
As shown in , it can be concluded that with increase in concentration of hydrophobic polymer, percentage drug release was decreased after 48 h (Kuksal et al., Citation2006). F9 (core:coat 1:3) exhibited nearly 84.1 ± 5.7% drug release within 48 h of incubation in dissolution medium. There is great impact of concentration of both the polymers in drug release. F10 also shows nearly same drug release as that of F9 within 48 h of incubation in dissolution medium. This is due to the fact that with increase in concentration of ethyl cellulose, i.e. hydrophobic polymer, viscosity goes on increasing, which leads to large particle size and less surface area for drug to expose in release medium, which decreases drug release in first 5 h (Balkrushna et al., Citation2012); thus, showed delayed release. Another reason might be decreasing the wet ability of microparticles in medium due to increased concentration of hydrophobic polymer, i.e. ethyl cellulose around the drug. Thus, dissolution rate of drug decreases (Grattard et al., Citation2002).
While, with increase in concentration of pectin, it creates pores around drug at pH 6.8 during first 5 h. Thus, on exposing it in release medium, medium gets diffuse inside particles and drug starts releasing faster from that pores. But, due to the presence of ethyl cellulose, 50% of drug was released during first 24 h, while 84 ± 5.7% of drug was released during 48 h.
At pH 6.8, pectin starts dissolving and whole drug was released from matrix due to the absence of any delayed release polymer, i.e. ethyl cellulose (Liu et al., Citation2003). Thus, 84 ± 5.7% of drug was released during 48 h. This type of formulation is suitable for targeting in small intestine ().
In vivo pharmacokinetic study
Enteric coating of microparticles has been reported to increase circulation in blood, which ultimately increases vascular-protective action of drug in body (Agnihotri et al., Citation2004). Pharmacokinetic analysis of optimized formulation F9 was compared with pure drug. Final formulation F9 shows significant increase in circulation time as compared to pure drug. Formulation F9 drug was detectable in plasma up to 12 h, which shows an increase in circulation time of drug. It also correlates well with obtained in vitro release data. The results show that ECMP had significantly improved the exposure, reduced the clearance and raised the oral bioavailability of drug (biochanin A) ( and ).
Table 4. Pharmacokinetic parameters of ECMP of biochanin A and pure drug (biochanin A) calculated by using plasma concentration time data using Thermo Kinetica, Version 5.0 (Thermo Fisher Scientific, Waltham, MA).
Effect of pharmacological interventions on systolic, diastolic and mean arterial pressure
Significant increase in systolic, diastolic and mean arterial pressure has been observed in hypertensive ovariectomized rats as compared to sham control rats. Administration of BCA (10 mg/kg, p.o.), ECMP (10 mg/kg, p.o.) and lisinopril (10 mg/kg, p.o.) decreases systolic, diastolic and mean arterial pressure in Ovx-HT rats. ECMP (10 mg/kg, p.o.) more significantly decreases systolic, diastolic and mean arterial pressure in Ovx-HT rats as compared to plain BCA. However, L-NAME (10 mg/kg, p.o.) attenuates the protective effect of ECMP ().
Figure 5. Effect of pharmacological interventions on systolic blood pressure in ovariectomized hypertensive rats.
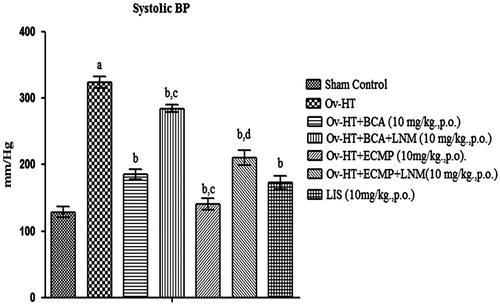
Effect of pharmacological interventions on TBARS level
Significant increase in TBARS level has been observed in hypertensive ovariectomized rats. Administration of BCA (10 mg/kg, p.o.), ECMP (10 mg/kg, p.o.) and lisinopril (1 mg/kg, p.o.) reduces TBARS level in ovariectomized hypertensive rats. ECMP (10 mg/kg, p.o.) more significantly decreases TBARS as comparison to plain BCA. L-NAME (10 mg/kg, p.o.) attenuates the protective effect of ECMP ().
Effect of pharmacological interventions on reduced GSH
Significant decrease in reduced GSH level has been observed in hypertensive ovariectomized rats. Administration of BCA (10 mg/kg, p.o.), ECMP (10 mg/kg, p.o.) and lisinopril (1 mg/kg, p.o.) significantly improved reduced GSH level concentration in the respective groups of ovariectomized hypertensive rats. ECMP (10 mg/kg, p.o.) showed significant results as compared to plain BCA. However, L-NAME (10 mg/kg, p.o) attenuates the protective effect of ECMP ().
Effect of pharmacological interventions on serum nitrite level
Serum nitrite level was significantly decreased in hypertensive ovariectomized rats as compared to sham control rats. BCA (10 mg/kg, p.o.), ECMP (10 mg/kg, p.o.) and lisinopril (1 mg/kg, p.o.) significantly improve serum nitrite/nitrate concentration in the respective groups of Ovx-HT rats. ECMP shows significant results than plain BCA. L-NAME (10 mg/kg, p.o) with ECMP attenuates the protective effect of ECMP ().
Effect of pharmacological interventions on TNF-α level
Significant increase in TNF-α level has been observed in hypertensive ovariectomized rats. Administration of BCA (10 mg/kg, p.o.), ECMP (10 mg/kg, p.o.) and lisinopril (1 mg/kg, p.o.) decreases TNF-α in Ovx-HT rats. ECMP showed more significant results as compared to plain BCA. L-NAME (10 mg/kg, p.o) with ECMP attenuates the protective effect of ECMP ().
Histology
Group A: Sham Control, Group B: Ovx-HT, Group C: Ovx-HT + BCA (10 mg/kg, p.o.), Group D: Ovx-HT + BCA (10 mg/kg, p.o.) + L-NAME (10 mg/kg, p.o.), Group E: Ovx-HT + ECMP (10 mg/kg, p.o.), Group F: Ovx-HT + ECMP (10 mg/kg, p.o.) + L-NAME (10 mg/kg, p.o.), Group G: Ovx-HT + Lisinopril (1 mg/kg, p.o.) ().
Discussion
In postmenopausal women, estrogen replacement therapy markedly reduces the risk of cardiovascular disease (Wang et al., Citation2005; Yang & Reckelhoff, Citation2011). However, the utilization of hormone replacement therapy as a cardioprotective strategy is greatly limited owing to carcinogenic effects in women and feminizing effects in men. It has been shown that dietary soy-derived estrogens are vasoactive and have valuable cardioprotective effects (Belcher & Zsarnovszky, Citation2001). BCA is naturally occurring phytoestrogens obtained from plants and it is nonsteroidal estrogens, which exerts estrogen actions (Belcher & Zsarnovszky, Citation2001; Sumien et al., Citation2013). Systemic arterial compliance has been documented to be improved by biochanin A in menopausal women (Teede et al., Citation2003). It has also been shown to relax the rabbit basilar artery (Torregrosa et al., Citation2013). Furthermore, Wang et al. subsequently confirmed that biochanin A induces endothelium-independent relaxation in rat aortic rings by blockage of Ca2+ entry through the cell membrane and activation of K+ channels. In spontaneously hypertensive rats, biochanin A-induced vasorelaxation was increased than in normotensive rats, which was mediated by the release of endothelium-derived substances that may open voltage-dependent and Ca2+ activated K channels in the vascular smooth muscle (Wang et al., Citation2006). BCA is an isoflavanoid and it has also been revealed that flavonoids could be incorporated into the plasma membrane and cytosol of endothelial cells and are beneficial for scavenging ROS (Youdim et al., Citation2000).
However, the oral bioavailability of biochanin A has been documented to be only 4.6% due to high clearance and a large apparent volume of distribution. BCA is a BCS CLASS II drug due to its poor water solubility. Moreover, this drug is associated with the certain drawbacks like poor oral bioavailability due to extensive hepatic first-pass metabolism and susceptibility to hydrophilic degradation in GI tract. Reason for the causes of the poor oral bioavailability of biochanin A had been explored to be using microsomal, cellular and animal models consistently indicate that rapid conjugation, especially glucuronidation is primarily responsible for the poor bioavailability of phenolics, although other factors such as stability and solubility might also be involved sometimes (Singh et al., Citation2011).
Therefore, in the present study, ECMPs of biochanin A were prepared to increase its intestinal absorption, aqueous solubility and oral bioavailability. Formulation of BCA microparticles was prepared by ionic-gelation method. The optimized formulation constitutes of pectin 6%, sodium alginate 2% and calcium chloride 6%. Coating of biochanin A microparticles was done by ethyl cellulose using coacervation-phase separation technique. Ratio of core (BAMP):coat (ethyl cellulose) in coated microparticles was 1:3. For optimization of method of coating of microparticles of biochanin A, parameters employed were speed of stirrer and pectin ratio.
Polymer concentration has significant impact on average diameter of the prepared microparticles. With increasing ethyl cellulose concentration, particle size was increased, while with increasing pectin concentration, particle dimension was decreased. Therefore, the fact is that with increase in ethyl cellulose concentration, medium viscosity also increases, ensuing in the formation of large solvent droplet and consequently, large-sized microparticles (Balkrushna et al., Citation2012). But in case of increasing pectin medium viscosity is lower in comparison to ethyl cellulose at same concentration, resulting in the formation of small-sized droplet, and subsequently, small-sized microparticles. F7 and F9 were found to be optimized formulation on the basis of particle size, PDI and entrapment efficiency. Zetasizer, PDI and entrapment efficiency of drug-loaded microparticle, i.e. BAMP (F7) formulation, were found to be 3.36 µm, 0.290, 98.94%, respectively, and zetasizer, zeta potential, PDI and percentage of entrapment of ECMP was found to be 4.96 µm, −0.18 mV, 0.25, 94%, respectively. Particle size is determined by the zeta-sizer. Polymers basically agglumerate the particle and make a thick clog by attracting or attaching the particles with each other. So, stirring speed should keep constant and between 1000 and 1200 rpm to overcome the effect of polymer and stirring speed on size and shape of particles.
Increase in concentration of span 80 from 0.5% to 2% resulted in decrease in the particle size. This is due to inability of low concentration of surfactant to cover the entire droplet surface. Therefore, some droplets form agglomerate resulted in increase in particle size (Balkrushna et al., Citation2012). But with increase in surfactant conc. up to 2%, interfacial tension between two phases decreases, resulted in formation of stable emulsion with relatively smaller droplets and particle size. After particular concentration, particle size again goes on increasing. This may be due to aggregation of particles. From the optimized zeta potential, zetasizer and FTIR, formulation F9 (ethyl cellulose-coated microparticles) was prepared.
Further prepared ECMPs were characterized on the basis of FTIR. The FTIR spectrum of biochanin A in formulation revealed the presence of peaks at 3600–3200 cm−1 for phenolic OH stretching, 1716 and 1657 cm−1 for C = O stretching and 1000–1300 cm−1 for C–O stretch, respectively, indicating that there was no interaction between the drug and excipients used in the study.
In vitro release of ECMP of biochanin A was carried out in simulated intestinal fluid (SIF – pH 6.8) and simulated gastric fluid (SGF – pH 1.2). ECMP showed up to 84% release in SIF and up to 20% release within 24 h. From these results, it was concluded that by enteric coating with ethyl cellulose stomach degradation of biochanin A was prevented. Therefore, ethyl cellulose-coated microparticles prevent the drug from stomach and start drug release and are absorbed from intestine. The formulation was further evaluated for drug release kinetics. From the HPLC data, it was observed that ECMP increases the oral bioavailability up to 6 folds as compared to plain biochanin A.
Furthermore, the effect of formulation was investigated in ovariectomized hypertensive rats. DOCA was administered to ovariectomized rats to produce hypertension clinically mimicking postmenopausal hypertension and shielding effect on vasculature is lost by estrogen paucity. DOCA is a synthetic mineralocorticoid that causes salt retention consequently leading to volume overload hypertension (Waleska & Marcelo, Citation2011). Removal of ovaries decreases the level of estrogen, which attenuates the protective effect of estrogen and causes hypertension.
Postmenopausal hypertension leads to increase in the production of free radicals and reduces the antioxidant enzymatic activity. GSH is involved in the synthesis and repair of DNA, assists the recycling of vitamin C and vitamin E, blocks free radical damage, enhances antioxidant activity of vitamin C, facilitates the transport of amino acids and plays a critical role in detoxification. Thus, in the ovariectomized hypertensive rats, reduced GSH and elevated LPO levels were observed.
TNF-α is a pleotropic cytokine that is documented to be elevated in chronic inflammatory states such as hypertension and diabetes. Increase in TNF-α level has been associated with increased NaCl retention and hypertension. It also arbitrates organ damage by stimulating immune cell infiltration and cell death (Ramseyer & Garvin, Citation2013). Thus, in the present study, TNF-α level was assessed in aortic tissue.
Lisinopril is an angiotensin converting enzyme inhibitor and is well reported drug for hypertension. It is used as a positive control in the present study to improve vascular endothelial dysfunction and hypertension (Arora et al., Citation2010). Lisinopril significantly attenuates systolic, diastolic and mean arterial blood pressure in the present study.
Vascular endothelium releases nitric oxide, which stimulates vasorelaxation and preserves the integrity of vascular endothelium lining. Blood pressure is maintained by vasodilation that occurs by NO release by the blood vessels. Decrease in estrogen level causes dysfunction in vascular endothelium and dysfunction of vascular endothelium attenuates activity and expression of eNOS and consequently reduces release of nitric oxide consequently (Feletou & Vanhoutte, Citation2006). Moreover, in the current work, the observed decrease in expression of serum concentration of nitrate/nitrite in ovariectomized hypertensive rats observed maybe the consequences of vascular endothelium dysfunction.
BCA has been documented to stimulate eNOS and nitric oxide release. NO is vasodilatory and vasoprotective in nature. Microparticles of biochanin A significantly attenuated hypertension in ovariectomized rats as assessed in terms of decrease in systolic, diastolic and mean arterial blood pressure, decrease in oxidative stress (decrease TBARS and increased GSH level), TNF-α level and increase nitric oxide level. However, L-NAME an inhibitor of eNOS significantly prevented the ameliorative effect of biochanin A microparticles.
Conclusion
Thus, it may be concluded that ECMPs of biochanin A have shown delayed release capacity, increase in oral bioavailability up to 6 folds than plain biochanin A and exert antihypertensive effect in ovariectomized rats in an eNOS dependent manner.
Acknowledgements
We are thankful to Mr. Parveen Garg Honourable Chairman ISF College of Pharmacy, Moga for providing necessary facilities for the study.
Declaration of interest
Authors have no conflict of interest. Authors are solely responsible for the entire manuscript.
References
- Agnihotri SA, Mallikarjuna NN, Aminabhavi TM. (2004). Recent advances on chitosan-based micro- and nanoparticles in drug delivery. J Control Release 100:5–28
- Arora MK, Reddy BVK, Balakumar P. (2010). The low dose combination of fenofibrate and rosiglitazone halts the progression of diabetes-induced experimental nephropathy. Eur J Pharmacol 636:137–144
- Atyab F, Inanloo K, Dinarvand R. (2005). Bovin serum albumin-loaded pectinate beads as colonic peptide delivery system: preparation and in vitro characterization. Drug Deliv 12:367–75
- Balkrushna P, Vidhi M, Komal P, et al. (2012). Preparation and evaluation of ethyl cellulose microspheres prepared by emulsification - solvent evaporation method. Int J Res Manage Pharm (IJRMP) 1:82–91
- Belcher SM, Zsarnovszky A. (2001). Estrogenic actions in the brain: estrogen, phytoestrogens, and rapid intracellular mechanisms. J Pharmacol Exp Ther 299:408–14
- Cetin M, Sahin S. (2015). Microparticulate and nanoparticulate drug delivery systems for metformin hydrochloride. Drug Deliv 22:1–10
- Chen Q, Li P, Li B, et al. (2010). Simultaneous determination of formononetin, biochanin A, daidzein and genistein in Trifolium pratense (red clover) by HPLC. LC–GC Europe 23:406
- Chourasia MK, Jain SK. (2004). Polysaccharides for colon targeted drug delivery. Drug Deliv 11:129–48
- Ellman GL. (1959). Tissue sulfhydryl groups. Arch Biochem Biophys 82:70–7
- Feletou M, Vanhoutte PM. (2006). Endothelial dysfunction: a multifaceted disorder (the Wiggers Award Lecture). Am J Physiol Heart Circ Physiol 291:H985–1002
- Grattard N, Pernin M, Marty B, et al. (2002). Study of release kinetics of small and high molecular weight substances dispersed into spray-dried ethyl cellulose microspheres. J Control Release 84:125–35
- Harini R, Ezhumalai M, Pugalendi KV. (2012). Antihyperglycemic effect of biochanin A, a soy isoflavone, on streptozotocin-diabetic rats. Eur J Pharmacol 676:89–94
- Khajuria DK, Razdan R, Mahapatra DR. (2012). Description of a new method of ovariectomy in female rats. Rev Bras Reumatol 52:466–70
- Kuksal A, Tiwary AK, Jain NK, Jian S. (2006). Formulation and in vitro, in vivo evaluation of extended-release matrix tablet of Zidovudine: influence of combination of hydrophilic and hydrophobic matrix formers. AAPS Pharm Sci Tech 7:E1–9
- Kumar L, Verma S, Jamwal S, et al. (2014). Polymeric microparticles-based formulation for the eradication of cutaneous candidiasis: development and characterization. Pharm Dev Technol 19:318–25
- Liu LS, Fishman ML, Kost J, Hicks KB. (2003). Pectin-based systems for colon-specific drug delivery via oral route. Biomaterials 24:3333–43
- Lorenzo-Lomosa ML, Remunan-Lopez C, Villa-Zato JL, et al. (1998). Design of microencapsulated Chitosan microspheres for colonic drug delivery. J Control Release 52:109–18
- Paharia A, Yadav AK, Rai G, etal. (2007). Eudragit-coatedpectinmicrospheresof5-fluorouracilforcolontargeting. AAPS Pharm Sci Tech 8:12
- Patel P, Dhake A. (2012). Design and development of colon specific microspheres for chronotherapy of hypertension. J Pharm Bioallied Sci 4:S33–4
- Patel B, Modi V, Patel K, Patel M. (2012). Preparation and evaluation of ethyl cellulose microspheres prepared by emulsification-solvent evaporation method. Int J Res Manage Pharm 1:82–91
- Perez MH, Lamprecht A, Ubrich N, et al. (2000). The preparation and evaluation of poly(ε-caprolactone) microparticles containing both a lipophilic and a hydrophilic drug. J Control Release 65:429–38
- Ramseyer VD, Garvin JL. (2013). Tumor necrosis factor-α: regulation of renal function and blood pressure. Am J Physiol Renal Physiol 304:F1231–42
- Sastry KVH, Moudgal RP, Mohan J, et al. (2002). Spectrophotometric determination of serum nitrite and nitrate by copper–cadmium alloy. Anal Biochem 306:79–82
- Singh SP, Wahajuddin, Jain GK. (2011). Intravenous pharmacokinetics and oral bioavailability of biochanin A in female rat. Med Chem Res 20:1627–31
- Sumien N, Chaudhari K, Sidhu A, Forster MJ. (2013). Does phytoestrogen supplementation affect cognition differentially in males and females? Brain Res 1514:123–7
- Tanaka M, Nakaya S, Wantanabe M, et al. (1997). Effect of ovariectomy and estrogen replacement in aorta ACE in rats. Jpn J Pharmacol 73:361–3
- Teede HJ, McGrath BP, DeSilva L, et al. (2003). Isoflavones reduce arterial stiffness: a placebo-controlled study in men and postmenopausal women. Arterioscler Thromb Vasc Biol l23:1066–71
- Torregrosa G, Burguete MC, Perez-Asensio FJ, et al. (2003). Pharmacological profile of phytoestrogens in cerebral vessels: in vitro study with rabbit basilar artery. Eur J Pharmacol 482:227–34
- Waleska CD, Marcelo ES. (2011). Animal models for the study of arterial hypertension. J Biosci 36:731–7
- Wang HP, Gao Q, Mei RH, et al. (2006). Mechanisms underlying biochanin A-induced relaxation of the aorta differ between normotensive and hypertensive rats. Clin Exp Pharmacol Physiol 33:802–7
- Wang HP, Mei RH, Li XY, et al. (2005). Endothelium-independent vasorelaxant effect of the phyto-estrogen biochanin A on rat thoracic aorta. Conf Proc IEEE Eng Med Biol Soc 3:2244–7
- Wanjari BE, Gaikwad NJ. (2012). Development and evaluation of verapamil hydrochloride xanthan gum microcapsules as controlled drug delivery system. IJPSR 3:3296–303
- Wienen W, Richard S, Champeroux P, Audeval-Gerard C. (2001). Comparative antihypertensive and renoprotective effects of telmisartan and lisinopril after long-term treatment in hypertensive diabetic rats. J Renin Angiotensin Aldosterone Syst 2:31–6
- Wills ED. (1966). Mechanisms of lipid peroxide formation in animal tissues. Biochem J 120:1147–54
- Yang XP, Reckelhoff JF. (2011). Estrogen, hormonal replacement therapy and cardiovascular disease. Curr Opin Nephrol Hypertens 20:133–8
- Youdim KA, Martin A, Joseph JA. (2000). Incorporation of the elderberry anthocyanins by endothelial cells increases protection against oxidative stress. Free Radic Biol Med 29:51–60