Abstract
Context: Intraoral drug delivery as mucosal delivery pathway provides a huge platform in the pharmaceutical field.
Objective: Combining mucoadhesiveness and controlled release of thio-poly acrylic acid as advanced excipient for buccal drug delivery.
Materials and methods: Mediated by carbodiimide, cysteine was covalently attached to poly acrylic acid. This thiomer was assessed with regard to cytotoxicity, stability, mucoadhesion, and rheology as well as release behavior of Lidocaine.
Results: Stability assays of thio-poly acrylic acid were complying with United States Pharmacopeia requirements. Mucoadhesion assay such as tensile (total work of adhesion), bioadhesion, rotating cylinder revealed as this thiomer was superior in comparison to non-thiolated poly acrylic acid with 7.61-fold, 2.8-fold, 5.61-fold improvement, respectively without any toxic effect. The cell viability exhibited over 90% after incubation time of 3 h and 24 h respectively. Lidocaine release showed 1.98-fold more controlled release over 3 h in comparison to unmodified poly acrylic acid.
Conclusion: Taken the findings in consideration, thio-poly acrylic acid provides excellent stability, controlled release, and superior mucoadhesive features. The prolonged residence time of thio-poly acrylic acid represents a pillar in the buccal drug delivery.
Introduction
Oro-mucosal formulations are described in the European Pharmacopeia as “containing one or more active substances intended for administration to the oral cavity and/or the throat to obtain a local or systemic effect” (Preis et al., Citation2014). Lidocaine hydrochloride may have both – on the one hand local action as pain reliever – on the other hand is also known for its systemic action as antiarrhythmic drug. In literature local anesthetic could be found in transdermal and dermal bioadhesive films (Leopold et al., Citation2002) and as well in terms of local anesthesia for oral mucosal biopsies (Roller & Ship, Citation1975).
Intraoral mucosal delivery is associated with benefits such as ease of administration, avoiding acid hydrolysis in the GI tract and circumventing the first-pass effect (Laffleur, Citation2014). Taking these benefits together buccal route of administration rendered beneficial and useful for a variety of drugs (Campisi et al., Citation2010). Buccal delivery involves the administration of the drug through the buccal mucosal membrane lining of the oral cavity (Kraan et al., Citation2014). A promising access to overcome this absorption barrier might be the application of multifunctional polymers exhibiting mucoadhesive and controlled release properties.
Since the beginning of the 80s buccal drug delivery of multifunctional polymers has been subject of interest (Patel et al., Citation2011). Multifunctional polymers namely thiolated polymers or thiomers belong to a unique group of polymers (Laffleur & Bernkop-Schnurch, Citation2012). These polymers have excellent multifunctional capacities and impressive features (Laffleur & Bernkop-Schnurch, Citation2013). Thiomers bear thiol-function on their polymeric backbone. So far, thiolated cationic polymers such as chitosan as well as thiolated anionic polymers such as hyaluronic acid were evaluated regarding their mucoadhesive potential. Due to covalent attachment of thiol groups to the backbone, outstanding properties were generated such as enhanced stability, improved mucoadhesion, efflux pump inhibition (Palmberger et al., Citation2015), permeation enhancement, and controlled release (Sarti et al., Citation2013).
The approach within this study was to follow the thiomer strategy by combining mucoadhesive and controlled release ability of the thiomeric excipients in order to overcome the limitations of buccal mucosa. Limitations associated with the buccal delivery are short residence time at the site of action which depends to the size and type of dosage form. Furthermore, small surface area as well as continuous secretion of saliva, which is a major hurdle to the dosage forms.
Therefore, poly acrylic acid as promising polymer was thiolated, characterized, and assessed in terms of stability, mucoadhesion, controlled release, and potential candidate for intraoral drug delivery system.
Materials and methods
Poly acrylic acid 250 (PAA) (exclusively linear, MM: 250 kDa on average), 1-ethyl-3-(3-dimethyl-aminopropyl) carbodiimide (EDAC), l-cysteine (CYS), resazurin salt, 5,5′-dithiobis-(2-nitrobenzoic acid), diethylamine, 4-(1,1,3, 3-tetramethylbutyl)phenyl-polyethylene glycol (Triton™ X-100) and Lidocaine hydrochloride were obtained from Sigma-Aldrich, Vienna, Austria. All chemicals were of analytical grade and were used as received.
Synthesis of thio-poly acrylic acid
Thio-poly acrylic acid was synthesized by carbodiimide-mediated synthesis. l-Cysteine was covalently attached to poly acrylic acid by the formation of amide bonds between primary amino group of cysteine and a carboxylic acid group of anionic polymer poly acrylic acid. Briefly, 1% (m/v) PAA solution in water was prepared and pH was adjusted to 6 by the addition of 5M NaOH (Sarti et al., Citation2013). 1 g 1-ethyl-3-(3-dimethylaminopropyl) carbodiimide hydrochloride (EDAC) was added to the polymer solution in order to activate carboxylic acid moieties. Water soluble EDAC is commonly used for coupling reactions. After 20 min incubation time under stirring at room temperature, in the next synthesis step, 1 g l-cysteine was added to the poly acrylic acid. Subsequently, pH was readjusted to 6 by the addition of 5M NaOH. The reaction mixture was incubated for 3 h at room temperature under stirring. The second step was a purification process, where unbound or excess l-cysteine was removed via dialysis in cellulose membrane tubings Nadir® dialysis tubes (Carl Roth GmbH, Karlsruhe, Germany). The purification took place at 10 °C in the dark twice against 0.2 mM HCl, twice against 0.2 mM HCl and 1% NaCl and at the end exhaustively against water. After purification, pH of the polymer solution was adjusted to 4 and was frozen at −80 °C. Finally, the frozen aqueous polymer solution was lyophilized (primary drying conditions of 23 h, beginning with 3 h at −30 °C, augmenting to −15 °C for 3 h, up to −10 °C for 2 h, following 17 h at 0 °C; secondary conditions of 8 h, starting with 1 h at +20 °C, and 7 h at +25 °C) and stored at 4 °C for further use. Controls were prepared in exactly the same way but omitting EDAC during the reaction.
Characterization of thio-poly acrylic acid
The amount of covalently attached cysteine with free thiol groups on the conjugates was determined with Ellman’s reagent 5,5′-dithio-bis(2-nitrobenzoic acid) (DTNB). Ellman’s buffer was prepared (1.871 g KH2PO4 and 84.613 g Na2HPO4 × 12 H2O dissolved in 500 ml purified water, pH 8) (Netsomboon et al., Citation2015). 1 mg of the thiolated polymer was dissolved in 500 μl Ellman’s buffer. Calibration reference solution of l-cysteine dissolved in Ellman’s buffer was prepared enabling evaluation of thiol group content. 500 μl freshly prepared Ellman’s reagent (3 mg DTNB dissolved in 1 ml Ellman’s buffer) was added to the samples. Samples were incubated in the dark at room temperature for 90 min. Afterwards, spectrophotometrical measurement (absorbance 450 nm) was completed by using the Tecan infinite M200 (Grödig, Austria).The principle of the Ellman’s assay is based on mixed disulfide bonds which were obtained due to thiol-disulfide exchange reaction. First, colorless Ellman’s reagent DTNB develops during building of mixed thiol-disulfide reaction yellow colored TNB (5-thio-2-nitrobenzoic acid). Second, recalculations according to the amount of TNB were performed by spectrophotometrically detection at 450 nm (Grabovac et al., Citation2015).
Furthermore, thio-poly acrylic acid was characterized by attenuated-total-reflectance Fourier transform infrared spectroscopy (ATR-FT-IR). FT-IR characterization was accomplished to prove successfully synthesized thio-conjugate. The spectra were recorded with a Perkin Elmer Spectrum 100 ATR-FTIR spectrometer (Perkin Elmer, Waltham, MA, USA) linked with a Spectrum software version 6.3.1.0134 (Perkin Elmer, Waltham, MA, USA). The polymer samples were separated in eight subsamples. The samples were recorded at 22 °C. The total of eight scans in a range from 4000 cm−1 to 600 cm−1 and a resolution of 4 cm−1 were detected (Laffleur et al., Citation2015a).
Cell viability
Cell viability studies were performed by resazurin assay. In order to evaluate the toxicity of the synthesized polymers in Caco-2 cells, 1 × 105 Caco-2 cells were seeded per well in 24-well plates (Laffleur et al., Citation2013a). Cells were incubated in a humidified chamber at 37 °C, 5% CO2. The cytotoxicity of the tested thio-poly acrylic acid and unmodified poly acrylic acid was determined at concentration 0.5% (m/v), respectively. Minimum essential medium (MEM) without phenol red and 2% (v/v) Triton X-100 served as low and high control, respectively. After an incubation period of 12 h with the testing mixtures, the medium was replaced from each well with 250 μl of 44 μM of resazurin solution. In the following step, cells were incubated at 37 °C, 5% CO2 for 3 and 24 h, respectively. Afterwards, fluorescence was measured at 540 nm excitation and 590 nm emission wave length with a microplate fluorescence using a Spectrophotometer DU® Series 600 (Jennings et al., Citation2007). Viability of cells was calculated based on the following equation:
Evaluation of intraoral disk
Lyophilized unmodified poly acrylic acid and lyophilized thio-poly acrylic acid were compressed to flat disks, respectively. The lyophilized conjugates, poly acrylic acid and thio-poly acrylic acid weight 30 mg and were of 5 mm diameter. The compaction was performed with a single punch eccentric press (Paul Weber, Remshalden, Germany) and a constant compaction pressure of 12 kN. The pH of the discs’ surface was neutral.
Water uptake behavior
Water uptaken capacities of testing conjugates and controls were determined by a gravimetric method as described previously (Peh & Wong, Citation1999). Briefly, 30 mg weighted disks were fixed on a clip and incubated in phosphate buffered saliva fluid. At prescheduled time points, the swollen test disks were removed from the incubation medium, and the amount of water uptake was determined gravimetrically after removal of exceed water from the surface was the amount of water uptake determined according to the following equation:
Wt is the disc weight at a given time point. W0 is the initial weight of the disc (Laffleur et al., Citation2014).
Erosion behavior
Erosion study was conducted by following a previously published method (Laffleur et al., Citation2015b). Shortly, the maximum swollen disks were dried in a hot-air oven at 50 °C until constant weight was reached. Dry discs of unmodified and thio-poly acrylic acid were weighed and placed in a 15 ml containing simulated saliva fluid, respectively. After the maximum of water uptake was reached, the swollen disks were removed from the vessel and brought to dryness. After reweighting, percentage of erosion of discs was determined by following equation:
where Wt is the weight of matrix subjected to erosion, t is time, and Wi is the initial starting weight of the matrix.
Disintegration assay
The disintegration of thio-poly acrylic acid and corresponding unmodified one was analyzed in simulated saliva fluid, respectively. The test was performed with the disintegration test apparatus according to the European Pharmacopoeia at an oscillating frequency of 0.5 s−1 and 37 °C (Hauptstein et al., Citation2013).
Buccadhesive assays
Rotating cylinder assay
In order to evaluate the buccadhesive properties of the polymer conjugates the rotating cylinder method was accomplished. For this purpose, freshly native porcine buccal mucosa was attached to the rotating cylinder. The buccal mucosa was obtained from a local slaughterhouse, Josef Mayr, Natters, Austria. The rotating cylinder was set to 125 rpm and immersed in vessels containing 900 ml simulated saliva fluid (Millotti et al., Citation2014). The detachment of the tested polymers was determined visually over the time period.
Tensile assay
Thiolated and unmodified disks were attached to a stainless flat disc (10 mm diameter) hanging from a laboratory stand with a nylon thread (10 cm). Freshly excised porcine buccal mucosa was placed on glass platform using a cyanoacrylate adhesive. The glass platform was placed on a balance and was carefully raised by a mobile platform until the disk got in contact with the buccal mucosa. After incubation time of 20 min, mucosa was pulled down by moving the platform at a rate of 0.1 mm/s. Data points were collected every second by computer software (SartoCollect V1.0; Satorius AG, Germany). The total work of adhesion (TWA) representing the area under the force/distance curve and the maximum detachment force (MDF) were determined (Nowak et al., Citation2014).
Bioadhesion assay
According to the bioadhesion test, the amount of remaining thio-poly acrylic acid and corresponding unmodified poly acrylic acid was determined after incubation on the freshly excised porcine buccal mucosa. Hurler et al. described the bioadhesion method previously. First, testing disks were stored overnight in a desiccator and weighed. Second, disks of thiolated and unmodified poly acrylic acid were attached to freshly excised porcine buccal mucosa and were incubated for 6 h at 37 °C. In the following step, test disks were detached from the mucosa and brought to dryness in a hot-air oven at 50 °C overnight followed by exsiccation to constant weight. Polymeric disks were reweighed after reaching constant weight. The amount of mucosal-remaining polymers was calculated according to following equation:
W0 is the initial starting weight of the polymeric formulation. Wt is the weight after dryness.
Agar–mucus plate assay
In order to evaluate the adhesiveness of the synthesized thio-poly acrylic acid an agar plate of 10 cm in diameter was prepared. On the surface of the plate 2 ml mucus was placed. The agar–mucus mixture was kept at 4 °C for 3 h. After preparing the agar–mucus plate, disks of thio-poly acrylic acid and unmodified one, respectively, were attached to the mucus. The mounted plate was moved up and down in saliva buffer at 25 °C after 20 min incubation time. The adhesion potential was visually determined according to the residence time of the disks on the plate (Luppi et al., Citation2009).
Rheological measurements
Preparation of the polymer/mucus mixtures
Porcine mucus (4 g) was hydrated in 25 ml of demineralized water under continuous stirring overnight at 4 °C. The mucus solution was adjusted to pH 6.8 with 1M NaOH and diluted to a final volume of 50 ml with 0.1 M phosphate buffer pH 6.8. The mucus stock solution (8% m/v) was stored at 4 °C. Thio-poly acrylic acid and unmodified PAA were hydrated in demineralized water to obtain a 4% (m/v) solution. The solutions of polymer conjugates were added to an equal volume of mucus stock solution by mixing and adjusting the pH 6.8 with 1M NaOH (Mahmood et al., Citation2015).
Rheological assay
The measurement was conducted with a plate-plate rheometer (Haake Mars Rheometer, 379-0200, Thermo Electron GmbH, Karlsruhe, Germany; Rotor: C35/1°, D = 35 mm) in order to evaluate the viscoelastic characteristics of the PAACYS and unmodified PAA. The experimental set up was determined to a shear stress at a range of 0.5–500 Pa and the temperature at 37 ± 0.1 °C. First, 160 mg of the modified and unmodified PAA, respectively, were hydrated in 800 μl of demineralized water for 30 min and measured. In the second measurement mucus–polymer mixture as described above were measured. 160 mg mucus–polymer mixtures were incubated for 0 h, 2 h, and 4 h, respectively. Preliminary strain sweep measurements were performed to determine the linear viscoelasticity range. Dynamic oscillatory tests within the linear viscoelastic range were performed with 1 ml aliquots of the samples at predetermined time points (0, 1 h, 2 h, and 4 h). According to oscillating measurements, phase shift angle (δ), the shear deformation (γ), and the shear stress (τ) were obtained. The elastic modulus (G′), the viscous modulus (G″), and the dynamic viscosity (η*) were calculated using the equations given below, the angle frequency (ϖ) was kept stable at 6283 rad/s.
Phase shift or phase angle (δ) is defined by δ = tan-1 G′/G″ and displays whether a material is liquid-like component or solid-like component. In rheological terms, a gel is defined when G′ and G’’ are frequency independent and tan δ is greater than 1. If G′ is equal to G′ at the crossover point, it means the polymer has as much elastic as viscous components (Partenhauser et al., Citation2015).
Lidocaine release
Lidocaine 1 mg was incorporated in the polymeric disks (30 mg). Lidocaine release was evaluated for unmodified poly acrylic acid disks and thio-poly acrylic acid disks. Therefore, PAACYS and PAA disks were immersed in a vessel containing 20 ml of release medium. The vessels were placed on an oscillating water bath at 37 °C maintaining sink conditions during the study (Laffleur et al., Citation2013b). At predetermined time points, aliquots of 200 μl were withdrawn for 180 min and replaced by 200 μl fresh release medium. The amount of released Lidocaine was determined by HPLC analysis and calculated by interpolation with a standard curve. For the HPLC analyses, a mobile phase containing acetonitrile and phosphate buffer pH 8 was prepared. By using the column NUCLEODUR C-18, 4.6 × 250 mm, and 5 μm column and detector of UV at 210 nm, at a flow rate of 1.0 ml/min the amount of permeated Lidocaine was determined. Cumulative corrections were made for previously removed samples. HPLC analyses.
Statistical analysis
Statistical data analyses were performed using the t-test and nonparametric tests like Mann–Whitney U test with p < 0.05 as the minimal level of significance. Statistical data analyses were performed by using the software GraphPad Prism version 5.01.
Results and discussion
Synthesis and characterization of thio-poly acrylic acid
The pathway for the synthesis of thio-poly acrylic acid is a simple one-step reaction. The obtained thio-poly acrylic acid was synthesized via amide bond formation between the primary amino group of the amino acid and a carboxylic acid group of the polymeric backbone. The synthesis process is displayed in . The amount of thiol groups was quantified via Ellman’s reagent. PAACYS exhibit 168.17 ± 49.58 μmol/g polymer immobilized thiol groups. The lyophilized thio-poly acrylic acid appeared as white, odorless, and of fibrous structure. Conjugates were hydratable in aqueous solutions.
Figure 1. Synthesis of thio-poly acrylic acid mediated by carbodiimde via amide bond formation between poly acrylic acid and cysteine. 1% (m/v) PAA solution, pH 6 was incubated with 1 g EDAC, after 20 min incubation time, 1 g cysteine was added and pH readjusted to 6. The reaction mixture was stirred for 3 h.

The FT-IR spectra of unmodified poly acryl acid and thio-poly acrylic acid are shown in . The characteristic band at 3 363 cm−1 is declared for –NH2 and –OH groups stretching vibration as well as and at 3000–2500 cm−1. The bands for carboxylic acid can be seen in the infrared spectrum of poly acrylic acid. In case of FT-IR spectra of thio-poly acrylic acid, the characteristic band from 4000 to 1600 cm−1 is similar to unmodified poly acrylic acid. However, at 1650 cm−1, a new peak appears. This peak is pointed out to amide bond, which provides evidence for the succeeded covalent attachment of l-cysteine to the polymeric backbone via amide bond formation.
Safety consideration
The poly acrylic acid was introduced to the pharmaceutical literature as biocompatible and safe to use. Within this study thio-poly acrylic acid was investigated for its harmful effect on cell culture. For this purpose, resazurin assay was conducted with testing polymer mixtures for an incubation time of 3 and 24 h, respectively, as shown in . Cell viability values of 95.61 ± 4.24% (3 h) and 91.78 ± 3.81% (24 h) in terms of unmodified poly acrylic acid and 95.28 ± 2.41% and 93.18 ± 4.83% (24 h) in presence of thio-poly acrylic acid after incubating, respectively, proved that the synthesized thio-poly acrylic acid showed not at all a harmful effect on the cells.
Intraoral disk evaluation
Water uptake behavior plays an important role is a major part of mechanism responsible for adhesive and cohesive properties as well as stability during drug release and disintegration. Furthermore, absorbing, capillarity processes, and swelling explain the adhesion between the polymer and the mucus layer (Mortazavi & Smart, Citation1993). Results of water uptake study are presented in showing covalent attachment of a thiol ligand leading to a pronounced increase of water uptake and swelling behavior. Water uptake of thio-poly acrylic acid was 2.6-fold higher in comparison with unmodified PAA. Besides the elevated abilities of water uptake, the stability of thiolated matrix tablets exceeded the cohesive properties of unmodified poly acrylic acid discs.
Figure 4. Water uptake study of unmodified PAA (open dot) and PAACYS (gray dot) discs in artificial saliva pH 6.75 at 37 °C. Indicated values are means (±SD) of at least three experiments.
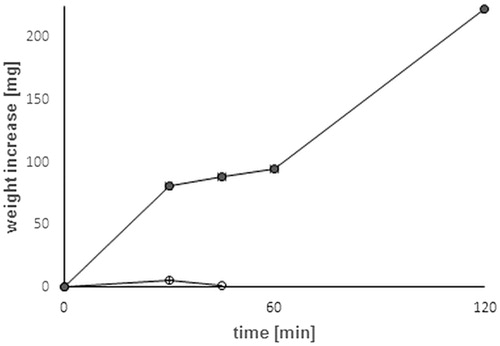
In hydrophilic polymeric matrix systems, the carrier on the surface of the matrix initially hydrates during dissolution to generate an outer viscous layer. This phase is then sequentially followed by matrix bulk hydration, swelling, and erosion. Therefore, the overall dissolution rate and ultimately the drug availability are inter alia controlled by matrix erosion (Roy & Rohera, Citation2002). The outcome of the matrix erosion studies are shown in elucidating the amount of polymer dissolved and the erosion of matrix during the maximum time of swelling process. The percent weight loss of thiolated polymeric matrix was 5.7-fold lower than the one of unmodified poly acrylic acid tablets.
Figure 5. Erosion study of unmodified PAA and thio-poly acrylic acid. Indicated values are means (±SD) of at least three experiments (*p< 0.05 compared to control).
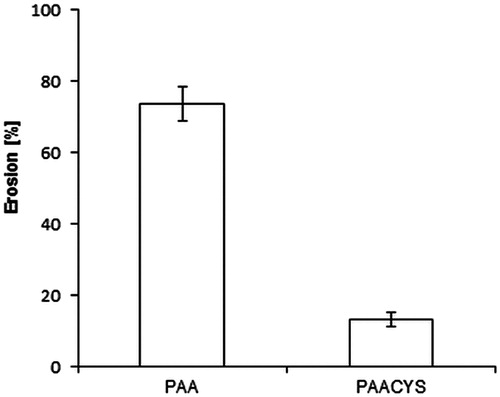
The intermolecular or intramolecular forces, the water uptake (swelling behavior) and the cohesiveness of a hydrophilic polymer exhibit a great impact on mucoadhesion. In case of low cohesive forces, the adhesive bond will be arranged by a connection between the polymer and the mucus layer rather than within the polymer itself. As a consequent the disintegration profile of tablets containing hydrophilic polymers is shown as a suitable indicator for their cohesive properties (Shahnaz et al., Citation2010). Disintegration studies were accomplished in order to evaluate the cohesiveness of the thio-poly acrylic acid in comparison with unmodified poly acrylic acid. As presented in studies revealed a 4.79-fold higher stability of tablets based on thiolated polymer in comparison to unmodified PAA.
Buccadhesive assays
Buccal drug dosage forms are controlled by different influencing factors limiting therapeutic success due to low mucoadhesive properties. The application of mucoadhesive systems is needed to maintain intimate and extended contact of the formulation with the oral mucosa allowing prolonged residence time at the active site of action (Patel et al., Citation2011). The rotating cylinder assay is supposed to correlate properly with the physiological conditions by imitating the adhesive and cohesive properties of the polymeric matrix. Physiological aspects of the oral cavity including pH, fluid volume, enzyme activity, and the permeability of the oral mucosa play significant role in this process. Limiting factors such as structure and turnover of the mucosal surface are a determinant of successful performance for drug delivery systems designed for prolonged and controlled release. Mucoadhesive properties of PAACYS dominate the mucoadhesiveness of the unmodified PAA about 5.61-fold as shown in . Unmodified PAA attached almost 80 min to buccal mucosa whereas thio-poly acrylic acid polymer revealed an adhesive maximum of almost 450 min reflecting high mucoadhesiveness reached by thiolation.
Figure 7. Comparison of mucoadhesive properties according to rotating cylinder assay. Test discs (30 mg) based on unmodified and thio-poly acrylic acid. Indicated values are the means (±SD) of at least five experiments (*p< 0.05 compared to control).

Tensile strength assay represents a common method for the in vitro evaluation of mucoadhesive strength. The adhesive properties of PAA and PAACYS with regard to mucosal surface attachment were measured by quantification of the force being required to separate mucosal surfaces and modified or unmodified PAA. The findings are illustrated in . TWA as well as MDF indicate that PAA shows a lower affinity for the mucosa in comparison to PAACYS. The total values and tendencies for both TWA and MDF were in good correlation to each other. The total increase of thiol-poly acrylic acid-bearing thiol moieties as substantial augmentation in TWA was 7.61-fold and for MDF 1.46-fold compared to non-thiolated PAA. Taking a look at the difference between both polymers, again the total amount of attached thiol groups might be a likely explanation: PAACYS with attached thiol groups compared to the unmodified PAA is able to interact with the mucosal surface, e.g. mucus glycoproteins have cysteine-rich subdomains resulting in a greater force for the detachment of the mucosa pieces. As a result, the higher the amount of free thiol groups attached to the PAA, the more pronounced the mucoadhesive features are.
Figure 8. Evaluation of strength studies representing the mucoadhesiveness of PAA and PAACYS. Black bars represent the TWA and white bars represent the MDF, respectively. Indicated values are the means (±SD) of at least five experiments (*p< 0.05 compared to control).

An additional method carrying out mucoadhesive properties of thiolated and unmodified polymer was the evaluation of the mucosal strength connection. The bioadhesion assay was accomplished on freshly excised porcine buccal mucosa. The connection force being necessary to remove the tablets from the mucosa as well as the amount of lasting formulation on the mucosa was persisted. 16.36% of the PAACYS kept adhesive on the mucosal surface after detachment whereas remaining PAA counted only reflecting a 2.8-fold improvement of the bioadhesive strength with regard to thio-poly acrylic acid as shown in .
Figure 9. Bioadhesion assay. Mucoadhesive properties were studied according to bioadhesion assay. Test discs were attached to the buccal mucosa. Indicated values are the means (±SD) of at least five experiments (*p< 0.05 compared to control).
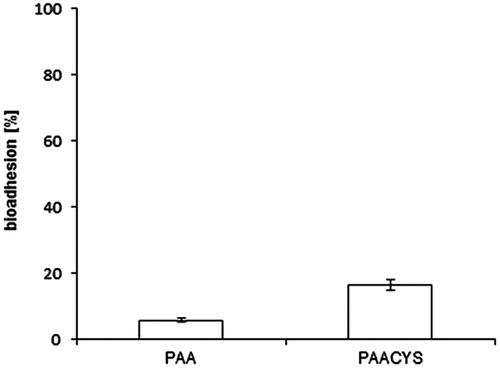
Furthermore, agar mucus assay was accomplished as shown in . Presented results were in good agreement with the performed mucoadhesive assay. During the agar mucus plate assay PAACYS demonstrated 4.4-fold improvement of mucoadhesive properties in comparison with unmodified PAA.
Figure 10. Residence time of unmodified (PAA) and modified (PAACYS) poly acrylic acid polymer discs (30 mg) on agar–mucus solution after incubating for 20 min. Using the modified tablet disintegration test apparatus in salvia buffer pH 6.75 at 25 °C. Indicated values are means (±SD) of at least three experiments (*p< 0.05 compared to control).

The rheological measurements provide disclosures about stress sweep and mucoadhesive connection with regard of increased viscosity. G′, G′′, η were determined at a constant angular frequency of 6.283 rad/s (=1 Hz) and were plotted as a function of time. The viscosity (η) at the beginning of the study showed 4.3-fold improvement in terms of PAACYS, at 2 h 4.6-fold higher values and after 4 h, 7.2-fold enhancement in viscosity in comparison to unmodified PAA, respectively, as seen in . The initial storage moduli of 0.2% PAACYS–mucus and the unmodified PAA–mucus showed a significant difference after 30 min. G′ of 0.2% PAACYS–mucus was more than 5-fold higher over the unmodified PAA–mucus (seen in ). The loss modulus of 0.2% PAACYS displayed a strong increase during the first 30 min. G’’ of 0.2% PAACYS–mucus was more than 3.4-fold increased compare to the unmodified PAA–mucus (). Unmodified PAA was found to have no interaction with mucus as their viscosity was remain constant over a time period of 4 h. PAACYS showed time-dependent increase in viscosity due to associative interactions with mucus glycoproteins and gradual oxidation of thiol moieties (Iqbal et al., Citation2012).
Figure 11. (a) Comparison of the η* of unmodified (triangle) and thio-poly acrylic acid (square) at 0 h, 2 h, 4 h at 37 °C. Indicated values are means (±SD) of at least three experiments (*p< 0.05 compared to control). (b) Storage modulus G′ of 0.2% unmodified (PAA) and modified (PAACYS)–mucus after 30 min. Indicated values are means (±SD) of at least three experiments (*p < 0.05 compared to control). (c) Loss modulus G′′ of 0.2% unmodified (PAA) and modified (PAACYS)–mucus after 30 min. Indicated values are means (±SD) of at least three experiments (*p< 0.05 compared to control).

Release study with Lidocaine
A sustained drug release is necessary for a prolonged therapeutic level of drugs, reducing consequently dosage frequency in order to improve the patients’ compliance. A simple diffusion process is shown for the release of the drugs out of the polymeric carrier systems. The efficacy of such a delivery system has been limited by a too accelerated disintegration and/or erosion of the polymeric matrix. In use of thiolated polymers, the limiting instability and consequently this essential shortcoming can be overwhelmed. For this reason, a controlled and prolonged drug release for numerous hours is guaranteed.
For this study, thiolated and unmodified polymer comprising 1 mg Lidocaine was evaluated in terms of drug release. In vitro release rate was analyzed by dissolution study and the amount of released Lidocaine was quantified by a HPLC method developed by Kang et al. (Citation1999).
As depicted in , the result provides evidence for a controlled prolonged and constant release profile of thio-poly acrylic acid compared to unmodified polymer, where drug release happens rapidly followed by descending.
Figure 12. Comparison of release profile of Lidocaine from unmodified (black rhomb) and thio-poly acrylic acid (open square) discs. Release studies were performed in simulated saliva fluid buffer pH 6.75 at 37 °C. The indicated release time represents an average of at least three experiments (±SD) (*p< 0.05 compared to control).
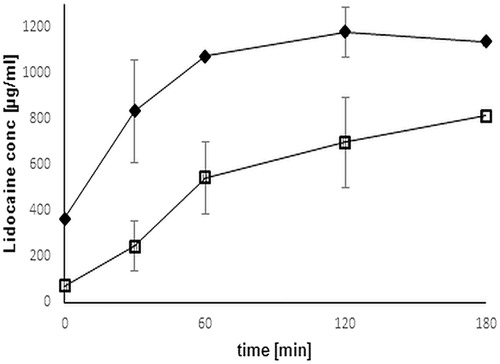
Conclusion
Presenting study showed the investigation of thio-poly acrylic acid regarding intraoral drug delivery of Lidocaine. Thio-poly acrylic acid was synthesized and evaluated in terms of stability, mucoadhesiveness, and release behavior. Due to the covalent attachment of thiol bearing ligand to the polymeric backbone, the multifunctionality of this commonly used poly acrylic acid could be improved as proved by mucoadhesive assays, stability assay. As far as the mucoadhesive features of the modified poly acrylic acid are concerned, the TWA and MDF seem to be in direct correlation with the thiol coupling efficiency. Thio-poly acrylic acid shows a significantly prolonged retention time in comparison to the unmodified control. The findings of this study point out that the thiolation of poly acrylic acid provides excipients with enhanced gelling, stability, mucoadhesive, and controlled features. So, regarding the advanced mucoadhesiveness of thio-poly acrylic acid commercially available formulations might be substantially improved due to a prolonged retention time on the mucosal surfaces. According to the results, the synthesized thio-poly acrylic acid being biocompatible and safe to use, opens the door for improved buccal formulations with controlled drug release.
Acknowledgements
The authors wish to thank the slaughterhouse Josef Mayr in Natters for providing the porcine mucosa.
Declaration of interest
The authors have no relevant affiliations or financial involvement with any organization or entity with a financial interest in or financial conflict with the subject matter or materials discussed in the manuscript. This includes employment, consultancies, honoraria, stock ownership or options, expert testimony, grants or patents received or pending, or royalties. No writing assistance was utilized in the production of this manuscript.
References
- Campisi G, Paderni C, Saccone R, et al. (2010). Human buccal mucosa as an innovative site of drug delivery. Curr Pharm Des 16:641–52.
- Grabovac V, Laffleur F, Bernkop-Schnurch A. (2015). Thiomers: influence of molecular mass and thiol group content of poly(acrylic acid) on efflux pump inhibition. Int J Pharm 493(1-2):374–9.
- Hauptstein S, Muller C, Dunnhaupt S, et al. (2013). Preactivated thiomers: evaluation of gastroretentive minitablets. Int J Pharm 456:473–9.
- Iqbal J, Shahnaz G, Dunnhaupt S, et al. (2012). Preactivated thiomers as mucoadhesive polymers for drug delivery. Biomaterials 33:1528–35.
- Jennings P, Koppelstaetter C, Pfaller W, et al. (2004). Assessment of a new cell culture perfusion apparatus for in vitro chronic toxicity testing. Part 2: toxicological evaluation. ALTEX, 21(2), 61–6.
- Kang L, Jun HW, Mccall JW. (1999). HPLC assay of Lidocaine in plasma with solid phase extraction and UV detection. J Pharm Biomed Anal 19:737–45.
- Kraan H, Vrieling H, Czerkinsky C, et al. (2014). Buccal and sublingual vaccine delivery. J Control Release 190:580–92.
- Laffleur F. (2014). Mucoadhesive polymers for buccal drug delivery. Drug Dev Ind Pharm 40:591–8.
- Laffleur F, Bernkop-Schnurch A. (2012). Thiomers: promising platform for macromolecular drug delivery. Fut Med Chem 4:2205–16.
- Laffleur F, Bernkop-Schnurch A. (2013). Strategies for improving mucosal drug delivery. Nanomedicine (Lond) 8:2061–75.
- Laffleur F, Fischer A, Schmutzler M, et al. (2015a). Evaluation of functional characteristics of preactivated thiolated chitosan as potential therapeutic agent for dry mouth syndrome. Acta Biomater 21:123–31.
- Laffleur F, Hintzen F, Rahmat D, et al. (2013a). Enzymatic degradation of thiolated chitosan. Drug Dev Ind Pharm 39:1531–9.
- Laffleur F, Roggla J, Idrees MA, Griessinger J. (2014). Chemical modification of hyaluronic acid for intraoral application. J Pharm Sci 103:2414–23.
- Laffleur F, Shahnaz G, Islambulchilar Z, Bernkop-Schnurch A. (2013b). Design and in vitro evaluation of a novel polymeric excipient for buccal applications. Future Med Chem 5:511–22.
- Laffleur F, Wagner J, Mahmood A. (2015b). In vitro and ex vivo evaluation of biomaterials’ distinctive properties as a result of thiolation. Future Med Chem 7:449–57.
- Leopold A, Wilson S, Weaver JS, Moursi AM. (2002). Pharmacokinetics of lidocaine delivered from a transmucosal patch in children. Anesth Prog 49:82–7.
- Luppi B, Bigucci F, Mercolini L, et al. (2009). Novel mucoadhesive nasal inserts based on chitosan/hyaluronate polyelectrolyte complexes for peptide and protein delivery. J Pharm Pharmacol 61:151–7.
- Mahmood, A, Bonengel S, Laffleur F, et al. (2015). Can thiolation render a low molecular weight polymer of just 20-kDa mucoadhesive? Drug Dev Ind Pharm. [Epub ahead of print]. doi:10.3109/03639045.2015.1061538.
- Millotti G, Laffleur F, Perera G, et al. (2014). In vivo evaluation of thiolated chitosan tablets for oral insulin delivery. J Pharm Sci 103:3165–70.
- Mortazavi SA, Smart JD. (1993). An investigation into the role of water-movement and mucus gel dehydration in mucoadhesion. J Control Release 25:197–203.
- Netsomboon K ,Laffleur F, Bernkop-Schnurch A. (2015). P-glycoprotein inhibitors: synthesis and in vitro evaluation of a preactivated thiomer. Drug Dev Ind Pharm. [Epub ahead of print]. doi:10.3109/03639045.2015.1075025.
- Nowak J, Laffleur F, Bernkop-Schnurch A. (2014). Preactivated hyaluronic acid: a potential mucoadhesive polymer for vaginal delivery. Int J Pharm 478:383–9.
- Palmberger TF, Laffleur F, Greindl M, Bernkop-Schnurch A. (2015). In vivo evaluation of anionic thiolated polymers as oral delivery systems for efflux pump inhibition. Int J Pharm 491:318–22.
- Partenhauser A, Laffleur F, Rohrer J, Bernkop-Schnurch A. (2015). Thiolated silicone oil: synthesis, gelling and mucoadhesive properties. Acta Biomater 16:169–77.
- Patel VF, Liu F, Brown MB. (2011). Advances in oral transmucosal drug delivery. J Control Release 153:106–16.
- Peh KK, Wong CF. (1999). Polymeric films as vehicle for buccal delivery: swelling, mechanical, and bioadhesive properties. J Pharm Pharm Sci 2:53–61.
- Preis M, Woertz C, Schneider K, et al. (2014). Design and evaluation of bilayered buccal film preparations for local administration of lidocaine hydrochloride. Eur J Pharm Biopharm 86:552–61.
- Roller NW, Ship II. (1975). Lidocaine topical film strip for oral mucosal biopsies. J Oral Med 30:55–8.
- Roy DS, Rohera BD. (2002). Comparative evaluation of rate of hydration and matrix erosion of HEC and HPC and study of drug release from their matrices. Eur J Pharm Sci 16:193–9.
- Sarti F, Muller C, Iqbal J, et al. (2013). Development and in vivo evaluation of an oral vitamin B12 delivery system. Eur J Pharm Biopharm 84:132–7.
- Shahnaz G, Perera G, Sakloetsakun D, et al. (2010). Synthesis, characterization, mucoadhesion and biocompatibility of thiolated carboxymethyl dextran-cysteine conjugate. J Control Release 144:32–8.