Abstract
S-nitrosothiols are a class of NO-donors currently under investigation for the treatment of various diseases. In this study, we developed a novel NO-donor (S-nitrosoglutathione-alginate, SNA) by cross-linking alginate with S-nitrosothiols, which can deliver NO in a sustained manner. This compound can be further evaluated for oral delivery to treat Crohn’s disease. This new compound was prepared using a two-step procedure involving (I) linkage of reduced glutathione to alginate and (II) post-nitrosation with sodium nitrite (NaNO2). The amount of linked thiol moieties for the possible nitrosation was calculated using Ellman’s method, and the amount of NO abducted on the polymer was calculated using the Griess–Saville method. An ex vivo model (i.e. Ussing chamber) was used to investigate the permeation of this new NO-donor across the rat intestinal barrier. We obtained polymers with different numbers of abducted NOs (174 ± 21 μmol/g for SNA F1 and 468 ± 23 μmol/g for SNA F2) depending on the procedure used for nitrosation. In the ex vivo studies in the Ussing chamber, SNA F2 exhibited a sustained release for at least 10 h. The effect of pH on the stability of the new compound was also investigated, and the new compound was more stable at a mildly basic pH of 8.4 where 73% remained after 1 week. However, only 50% remained after 1 week at an acidic pH of 1.2. In the cytotoxicity studies (Caco2), this compound was nontoxic at concentrations of less than 200 μM.
Introduction
Nitric oxide (NO) is the smallest endogenous molecule and a free radical gas (Saraiva et al., Citation2011). NO was first introduced in 1987 (Ignarro et al., Citation1981, Jerca et al., Citation2002) and received medical and scientific interest in 1992 when it was nominated as molecule of the year (Friedman & Friedman, Citation2009). NO is produced by endothelial cells of the body and performs different physiological functions. NO is released as NO* or bound to -SH groups containing carrier molecules that stabilize its release (Moncada et al., Citation1991; Kilbourn, Citation1996).
To evaluate the biological and physiological functions of NO, exogenous sources of NO-donors are required that can be used both as a drug and a research tool (Saraiva et al., Citation2011). A variety of NO-donors are available and pharmacologically active as NO releasing drugs including S-nitrosothiols (RSNOs) (Saraiva et al., Citation2011). In fact, due to the ability to act as NO-donors, RSNOs have attracted considerable attention. RSNOs are involved in different reactions in the bioregulatory system based on NO transfer (Singh et al., Citation1996b). RSNOs are more stable than NO, which has a very short in vivo half-life (0.1–15 s). For example, S-nitrosoglutathione (GSNO) decomposes in hours (Josephy et al., Citation1984; Shin & George, Citation2001). Some RSNOs, such as GSNO and S-nitrosoalbumin, are endogenous NO carriers in mammals (Seabra et al., Citation2005). NO is covalently attached to a sulfur atom in the R-S-NO molecule and can be released via S-N bond cleavage to transfer NO to specific receptors for in vivo biological effects (Seabra et al., 2005).
Chemically, RSNOs have been synthesized by reacting NO with compounds possessing thiol groups, such as reduced L-glutathione (GSH) and albumin. Then, these compounds are transported to the reaction sites where its corresponding biological effect is achieved by release of NO (Singh et al., Citation1996a). The most commonly used RSNOs are GSNO and S-nitroso-N-acetyl-d, l-penicillamine (SNAP), which exhibit remarkable biological effects (e.g. GSNO offers significant protection to myocardial ischemia and SNAP is a powerful vasodilator (Ignarro et al., Citation1981)).
Several other NO-donors, such as nitroglycerine and isosorbide dinitrate, rapidly release NO but can cause oxidative stress and frequent tolerance. Therefore, to minimize these problems, research is now focused on innovative NO-donors such as RSNOs and especially GSNO. GSNO is considered a lead molecule due to its safety (NO released is accompanied by GSH production). Therefore, GSNO is used to investigate the biochemical and pharmacological mechanisms of NO and its therapeutic applications (Parent et al., Citation2013). S-nitroso compounds, such as GSNO, have a specific thiol coupling capability that allows it to store and carry NO and offers the advantage of preventing the development of tolerance in patients. These compounds can be used in different disease states, such as cardiovascular disorders (Everett et al., Citation2014), brain ischemia (Khan et al., Citation2009), cancer (Furuhashi et al., Citation2012), cystic fibrosis (Servetnyk et al., Citation2011), and wound healing (Georgii et al., Citation2011). In addition, these compounds may be beneficial in the treatment of Crohn’s disease (Savidge et al., Citation2007). In the recent years, GSNO has been proposed for the treatment of Crohn’s disease to prevent mucosal barrier failure.
Crohn’s disease is a type of inflammatory bowel disease which is thought to be a result of inappropriate inflammatory response to intestinal microbes in a genetically susceptible host (Abraham & Cho, Citation2009). It is an immune-related disease in which the immune system attacks the gastrointestinal tract. It is characterized by focal, asymmetric, transmural, and occasionally granulomatous inflammation, which primarily affects the gastrointestinal tract (Thomsen et al., Citation1998). In maximum cases the diagnosis reports in colonic and terminal ileal involvement (Freeman, Citation2009).
GSNO significantly promotes human intestinal mucosal barrier function, while this effect was lacking in patients with no inflammatory bowel disease conditions (Savidge et al., Citation2007). This result may be due to the enteric glial cell network being disrupted in non-inflamed Crohn’s disease intestinal mucosa. Therefore, the tissue GSNO concentration levels might be lower in these patients (Shah et al., Citation2015). NO-donors can also be chemically linked to biopolymers, which can provide site-specific delivery of NO (Saraiva et al., Citation2011). Therefore, the aim of our study was to synthesize a new stable polymer by linking GSNO to alginate, and this compound was evaluated for the treatment of Crohn’s disease.
Alginates are naturally occurring polyanionic polysaccharides and are isolated from brown seaweed (Phaeophyceae (Tonnesen & Karlsen, Citation2002)), macrocystis pyrifera, laminaria hyperborea, and ascophyllum nodsum (Sosnik, Citation2014). Alginic acid, sodium and potassium alginates (ALG) are among the most extensively explored mucoadhesive biomaterials having good cytocompatibility, biocompatibility, biodegradation, and chemical versatility, which make them suitable for further modifications to its properties (Sosnik, Citation2014). In a study reported by Bernkop Schnurch et al, a mucoadhesive thiolated polymer was synthesized and characterized by linking alginate to cysteine. Since, alginate displays carboxylic acid groups; the sulfhydryl compound cysteine was easily covalently attached. This study showed that the thiolated alginate is a useful excipient for various drug delivery systems providing an improved stability and prolonged residence time (Bernkop-Schnurch et al., Citation2001).
In a study reported by Katsumi et al. (Citation2005), an NO-donor was synthesized by conjugating SATA (N-Succinimidyl-S-acetylthioacetate) with polyethylene glycol and bovine serum albumin. More recently, a new NO-donor was synthesized by the nitrosation of chitosan-GSH conjugate (SNOC) with a good value of linked NO to the polymer backbone (Shah et al., Citation2015).
Materials and methods
Materials
GSH, sodium alginate, Ellman’s reagent (5,5′-dithiobis (2-nitro benzoic acid), 2,3-diaminonaphthalene (DAN), N-1-(naphthyl)ethylenediamine (NED), 1-ethyl-3-(3-dimethylaminopropyl)carbodiimide (EDAC) and sulfanilamide were obtained from Sigma-Aldrich (Saint Quentin Fallavier, France). Sodium nitrite (NaNO2) and potassium hydrogen phosphate were purchased from Merck (Darmstadt, Germany), and “total nitric oxide and nitrate/nitrite kit” was obtained from R&D systems (Abingdon, UK). All of the other chemicals were of analytical or chromatographic grade.
Synthesis and purification of the alginate-GSH conjugate
GSH was covalently attached to sodium alginate via amide bonds between the carboxylic acid groups of the polymer and the amine groups of GSH () according to a previously reported method (Bernkop-Schnurch et al., Citation2001). Briefly, 10 g of sodium alginate was hydrated in 1000 ml of ultrapure demineralized water. For the amidation reaction, the carboxylic groups of the polymer were activated by addition of EDAC in a final concentration of 50 mM. The reaction was allowed to continue for 45 min at room temperature. After this, 5 g of GSH (in a polymer to drug ratio of 2:1) was added, and the pH was adjusted to 4 with HCl (1 M). This mixture was continuously stirred at 200 rpm for 2 h at room temperature. The pH was readjusted to 6 with NaOH (1 M), and the reaction was allowed to continue for further 1 h.
Figure 1. Reaction scheme for the synthesis of SNA. 1st step: synthesis of an alginate-glutathione conjugate. 2nd step: nitrosation of alginate-glutathione conjugate.
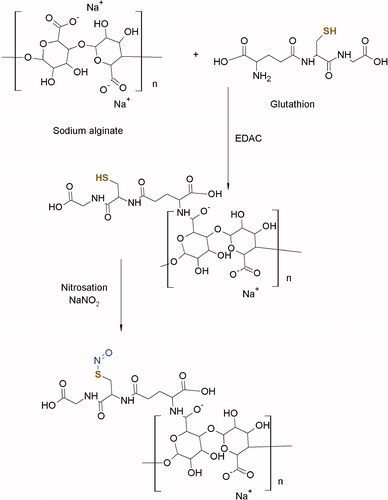
The resulting conjugate was dialyzed in tubing (molecular weight cutoff 12 kDa, Spectra/Por, Spectrum Laboratories, Paris, France) against HCl (1 mM), twice against HCl (1 mM) containing 1% NaCl and at the end against HCl (1 mM) for 5 h. The controls were prepared in the same way except using EDAC for the coupling reactions. The resulting conjugate was freeze-dried at −50 °C and 0.01 mbar followed by storage at 4 °C prior to use.
Determination of the thiol group content of various fractions of thiols after synthesis (Ellman’s method)
The thiol group content was determined using previously reported methods with slight modifications (Atyabi et al., Citation2008; Yousefpour et al., Citation2011). Briefly, 0.5 mg of the polymer conjugate was hydrated in 0.35 ml of deionized ultrapure water for 30 min and diluted to a final volume of 1 ml by adding 0.65 ml of Tris-HCl. This solution was labeled solution-1.
Reduced thiols
To a 100 μl of solution-1, 900 μl of phosphate buffer (0.1 M, pH 7.5) and 200 μl of Ellman’s reagent (0.3 mg/ml) were added and incubated for 15 min in the dark at room temperature. By using a UV-Visible spectrophotometer (Cary® 50 UV-Vis, Varian, Les Ulis, France), the absorbance was measured at 412 nm. The reduced thiol content was determined from a standard calibration curve of GSH with concentrations ranging from 3.25 to 32 μM.
Total thiols
For the determination of the total thiol groups either in their reduced form or disulfide bond form, 1 ml of NaBH4 (4% solution w/v) was added to 1 ml of solution-1 followed by incubation at 37 °C for 1 h. Then, this solution was neutralized with 200 μl of HCl (5 M) and allowed to incubate for further 10 min. After this, 1 ml of phosphate buffer (0.1 M, pH 7.5) was added followed by the addition of 200 μl of Ellman’s reagent. The solution was incubated in the dark for 15 min at room temperature, and the amount of total thiol groups was determined using a UV-Visible spectrophotometer (Cary® 50 UV-Vis, Varian, Les Ulis, France).
Thiol groups directly linked to alginate
The amount of thiol groups directly linked to alginate was determined using the same procedure that was mentioned above for the total thiol content. After a reducing pretreatment (1 ml of solution-1 with 1 ml of NaBH4) and neutralization, the polymer was dialyzed in tubing (molecular weight cutoff 12 kDa) for 4 h in distilled water to remove the free thiol groups. The amount of free thiol groups were determined by subtracting the number of thiol groups directly linked to the polymer from the total number of thiol groups.
Nitrosation of alginate-GSH conjugates (SNA)
NO was linked to the alginate-GSH conjugate via nitrosation with NaNO2 under acidic condition. Two methods were used to achieve nitrosation.
SNA-F1
Briefly, 100 mg of the alginate-GSH conjugates were hydrated in 45 ml of HCl (0.5 M) for 30 min. To this alginate-GSH conjugate solution, 1 ml of a NaNO2 solution (equimolar to the reduced thiols in alginate-GSH conjugates) in ultrapure water was added. The reaction was continued for 2 h at 4 °C in the dark and then neutralized with a NaOH solution (40% w/v).
SNA-F2
This method includes the nitrosation of all of the thiol groups (free and linked thiols) present in the alginate-GSH conjugate. Prior to nitrosation of the polymer, the disulfide bonds were broken by the addition of NaBH4 followed by treatment with HCl (5 M) to remove the unwanted NaBH4. Briefly, 100 mg of the alginate-GSH conjugate was wetted in 8.75 ml of ultrapure water and then diluted with 16.25 ml of Tris-HCl for 30 min to achieve complete hydration. To this solution, 5 ml of NaBH4 (4% w/v) was added and incubated for 1 h at 37 °C to break the disulfide bonds (R-S-S-R) present between the thiol groups in the conjugate solution. After 1 h of incubation, the remaining NaBH4 was eliminated by addition of 4 ml of HCl (5 M) followed by incubation for an additional 15 min under the same conditions. The final solution was cooled by adding 25 ml of HCl (0.5 M, 4 °C). To this solution, an equimolar NaNO2 amount (equal to total amount of thiol groups present in the conjugate solution) was added, and this solution allowed to stand in the dark at 4 °C for 2 h. The reaction was terminated by neutralizing it by adding NaOH (40% w/v). This solution was dialyzed for 4 h in ultrapure water using dialysis tubing (molecular weight cutoff 12 kDa) followed by storage at 4 °C in the dark prior to use.
Determination of the NO/NO2− concentration (Griess–Saville method)
An indirect method was employed for the determination of NO/NO2−, as described by Griess and Saville in 1958 (Heines, Citation1958; Saville, Citation1958). In a two-step diazotation reaction, dinitrogen trioxide (N2O3, NO-derived agent) generated from the acid-catalysed formation of nitrous acid from nitrite reacts with sulfanilamide to produce a diazonium ion. Then, this diazonium ion couples to N-(1-naphthyl)ethylenediamine to form a chromophoric azo product that can be detected at 540 nm. The concentration of the NO moieties was calculated from standard calibration curves of NaNO2. The limit of detection for these analytical experiments was 0.25 μmol/l, the limit of quantification was 0.77 μmol/l and the correlation coefficient was 0.999. The method described by Saville (Citation1958) is based on a pretreatment using mercuric chloride (HgCl2) that induces cleavage of RSNOs to form nitrite. This method allows for the determination of the total amount of NO/NO2−. For these experiments, the limit of detection was 0.11 μmol/l, the limit of quantification was 0.35 μmol/l, and the correlation coefficient was 0.999.
In the experiment, 20 μl of the sample was added to an acetic acid buffer (pH 2.5), and the final volume was adjusted to 200 μl. To determine the nitrite concentration, 40 μl of sulfanilamide (free nitrites) or sulfanilamide + HgCl2 (total nitrites) was added and incubated in the dark for 3 min. After incubation, 10 μl of a NED solution was added and incubated in the dark for an additional 5 min. The nitrite concentration was determined using a microplate reader (Elx800 Absorbance Microplate Reader, BioTek, Colmar, France) or UV-Visible spectrophotometer (Cary® 50 UV-Vis, Varian, Les Ulis, France). The concentration of the RSNOs (GSNO and S-nitrosoglutathione-alginate, SNA) was calculated using the following formula:
Stability of SNA F1 and SNA F2 in the Ussing chamber
The effect of the experimental conditions (temperature and O2/CO2) on the stability of SNA-F1 and SNA-F2 was investigated in one compartment of the Ussing chamber (CHM8, 0.5 cm2 WPI, Hitchin, UK). A 100 μM SNA solution in Hank’s buffer was added to the donor compartment of the Ussing chamber that was separated from the accepter compartment with a folded plastic film, consisting of several layers, to prevent flow to the other side. The temperature was adjusted to 37 °C, and the solution was circulated by a constant flow of O2/CO2. The samples were withdrawn at regular time intervals (i.e. 0, 0.5, 1, 1.5, 2, 3, 4, 5, 6, 7, 8, 9, and 10 h) and analyzed using a UV-Visible spectrophotometer.
Effect of pH on the storage conditions of SNA F2
The SNA F2 solution was stored at 3 different pH values (acidic pH 1.2, neutral pH 7.0, and basic pH 8.4) and 4 °C in the dark. The effect of pH was assessed every 2 d by calculating the amount of free and linked nitrites. The results were compared to the initial concentration of SNA F2 and are expressed as percentages.
Intestinal permeation studies in the Ussing chamber
Preparation of intestinal segments
Male Wistar rats (RjHan: WI, 400 g, 8–10 weeks old, males, Charles Rivers) were killed with 5% halothane in oxygen gas. Segments from the distal portion of the ileum were excised and washed in a beaker containing Krebs’s bicarbonate Ringer (KBR, 4 °C) bubbled with an O2/CO2 mixture to maintain membrane viability. These segments were flushed internally with approximately 20 ml of KBR and slid onto glass rods with tapered points wetted with KBR. These rods with intestinal segments were placed in buffer chilled in an ice bath at 3–4 °C. The serosa and muscularis externa were removed with tweezers according to the method described by Wolfe et al. (Citation1973). The segments were opened along the mesenteric border and mounted on the Ussing chamber (CHM8, 0.5 cm2 WPI, Hitchin, UK) equipped with a water jacket (37 °C).
Permeability studies through the rat intestine (Ussing chamber)
Eight milliliters of Hank’s BSS buffer without phenol red was added to both the donor and acceptor compartments of the Ussing chamber with the intestinal segment mounted between them. The temperature was maintained at 37 °C, and the flow in both chambers was controlled by O2/CO2. The trans-mucosal electrical resistance (TER) was monitored by current electrodes (WPI, Hitchin, UK) fixed in the two compartments. The tissues having TER <30 Ωcm2 were discarded. A 100 μl sample was withdrawn from the donor and acceptor compartments at appropriate time intervals (starting from 0 to 6 h) to calculate the SNA concentrations using the Griess–Saville method. Nitrite and nitrate ions, which are degradation products of SNA, were measured by Griess and Saville method and by “Total nitric oxide and nitrate/nitrite kit” (R&D systems), respectively.
Cytotoxicity study on the Caco2 cell line
The cytotoxic activity of SNA F2 was tested on the Caco2 cell line. The Caco2 cell assay is based on the reduction of tetrazolium dye MTT by mitochondrial succinate dehydrogenase to its insoluble form (i.e. formazan), which has a purple color and is quantified spectrophotometrically at 570 and 630 nm using a microplate reader. Briefly, the Caco2 cells at a concentration of 20.103 cells per well in 200 μl of medium were seeded into a 96-well culture plate. After 24 h, various concentrations of SNA F2 (1–200 μM) were added to the culture plates and incubated for an additional 24 h at 37 °C in a 5% CO2 humidified atmosphere. Afterwards, 50 μl of MTT (5 mg/ml stock solution in HBSS buffer) was added to each well in the culture plate and incubated for an additional 3 h at 37 °C. The medium was removed, and the formazan crystals were dissolved in 50 μl of dimethyl sulfoxide. Then, the culture plate was agitated for 5 min at 500 rpm. The absorbance was measured by a microplate reader. The experiments were carried out in triplicate, and the results are expressed as a percentage of viable cells compared to the control group.
Statistical data analysis
Statistical analyses were performed using the U-test with p < 0.05 as the minimum level of significance (Mann & Whitney, Citation1947) or the Kruskal–Wallis test.
Results and discussion
Synthesis of alginate-glutathione conjugates
The lyophilized form of alginate-GSH consists of a creamy white powder with a fibrous structure. Alginate was the first anionic biodegradable polymer, and this polymer has been chemically modified by covalent attachment to thiol (-SH) groups by Bernkop-Schnurch et al. (Citation2001). Following this method, alginate was successfully attached to GSH, and the number of thiol groups attached to alginate was quantified using Ellman’s method. The alginate-GSH conjugate contained 711 ± 24 μmol thiol groups per g of polymer, where 481 ± 22 μmol thiol groups were directly linked to the alginate polymer (). GSH consists of three amino acids, i.e. glutamate, cysteine, and glycine. Due to the sulfhydryl (-SH) groups in cysteine, GSH is present in a reduced form and chemically active for a variety of reactions (e.g. antioxidant reactions). GSH is also involved in direct reactions with a variety of oxidants in a non-enzymatic manner that results in its conversion to its dimeric oxidized form (i.e. glutathione disulfide, GSSG) (Appenzeller-Herzog, Citation2011). By breaking the disulfide bonds with NaBH4, all of the available thiol (-SH) groups can be converted to their reduced form (), which provides an opportunity to link a large quantity of NO molecules (e.g. the nitrosation process ()).
Table 1. Amount of glutathione (SH groups) determined by Ellman’s test (precursor of SNA F1).
Table 2. Amount of glutathione (SH groups) determined by Ellman’s test (precursor of SNA F2).
Table 3. Amount of nitrites determined by the Griess and Saville tests.
Nitrosation of the alginate-GSH conjugates
A pale yellowish solution was obtained after reacting the alginate-GSH conjugates with NaNO2 under acidic conditions (pH 1.2). The nitrosation process was performed using two different methods, and the amount of NO adducts to thiol moieties using both methods are shown in . SNA F2 has a greater number of linked NO groups compared with SNA F1 because SNA F1 is based on the nitrosation of only the reduced thiols present in the alginate-GSH conjugates, which are smaller in number compared with SNA F2 based on the nitrosation of all of the thiol groups present in the conjugates. In SNA F2, the disulfide bonds between the -SH groups were reduced by the addition of NaBH4 prior to nitrosation, which allows for the linkage of a larger number of thiol groups to NO. Comparatively, the amount of adducted NO to thiol groups was 174 ± 21 μmol per g in SNA F1. However, in SNA F2, this value was 468 ± 23 μmol per g, which suggests the suitability of SNA F2 for further experiments. Acidified NO2− can react with several functional groups on proteins, and two types of amino acid groups (i.e. cysteine and tryptophan) have been nitrosated in previous studies (Katsumi et al., Citation2004). The study also indicated that other amino acid residues including arginine, lysine, asparagine, glutamine, and tyrosine can also be nitrosated but it can result in unstable products. The nitrosation of the cysteine thiol group in the alginate-GSH conjugate resulted in a high concentration of abducted NO and good stability under different experimental conditions ( and ).
Stability of SNA F1 and SNA F2 in the Ussing chamber
The stabilities of SNA F1 and SNA F2 were evaluated in the Ussing chamber without intestine at 37 °C in Hank’s buffer with a constant O2/CO2 flow. As shown in , SNA F2 was more stable compared with SNA F1 and degraded into its degradation products after 10 h. This results may be due to the amount of abducted NO on each polymer because SNA F2 has a higher amount of NO, which makes it last longer. In our previous study (Shah et al., Citation2015), the stability of GSNO, which was studied at a basic pH of 7.4, was comparatively less stable than our new SNA compound.
Stability of SNA F2 under different storage conditions
The stability of SNA F2 was studied under various pH conditions at 4 °C in the dark for several weeks. The acidic condition consisted of 0.1 N HCl (pH 1.2), a neutral condition USP Phosphate buffer (pH 7.0), and a mildly basic conditions consisted of USP phosphate buffer at (pH 8.4). As shown in , at an acidic pH of 1.2, only 55 ± 4.9% of SNA F2 remained after 1 week, and all of the SNA F2 degraded within 2 weeks. The stability of SNA F2 did not improve at a neutral pH of 7.0 where 61 ± 2.8% remained after 1 week and 11 ± 2.9% remained after 2 weeks. As shown in , a mildly basic pH of 8.4 was more effective at decreasing the decomposition of RSNO where 73 ± 9.3% remained after 1 week and 43 ± 8.2% remained after 2 weeks. In addition, small amounts were still present until the 3rd week. The stability of RSNOs at various pH values was also evaluated in a previous study (Parent et al., Citation2013). In this study, the GSNO stability was evaluated at an acidic condition (pH 0.3) and a basic condition (pH 7.4) at a storage temperature of 4 °C. Based on the previous results, GSNO should be stored at a basic pH to ensure better storage conditions. A recent study conducted on the stability of RSNOs (i.e. GSNO, SNAC, and SN3MPA) suggested that RSNOs were more stable at mildly basic pH values (i.e. 8.4–8.8) after the exclusion of light at 4 °C, which are similar to our results (Hornyak et al., Citation2012). This new compound (i.e. SNA F2) is more stable than our previous compound S-nitrosoglutathione-oligosaccharide-chitosan (SNOC, Shah et al., Citation2015).
Permeability studies in the Ussing chamber
The ex vivo drug permeation studies were performed in an Ussing chamber across rat intestine. For the ex vivo study, only SNA F2 was selected due to its higher nitrosation values () and improved stability () compared with SNA F1. GSNO was also studied through the rat intestine for use as a control. The GSNO concentration remained for 5 h in the Ussing chamber ( and ) prior to being degraded into its two degradation products (i.e. nitrite (NO2−) and nitrate (NO3−) ions). The concentrations of the two degradation products were also calculated in the acceptor compartment, because these products were able to permeate the intestinal barriers. Nitrite ions were released in the first hours of the experiment and found at the end of the experiments. These nitrite ions can be considered a sign of NO formation (Schulz et al., Citation1999; Shah et al., Citation2015). Concentrations of nitrate ions, which can be considered the final products of RSNO degradation, increased throughout the experiments. Because equal amounts of medium were employed in both the donor and accepter compartments of the Ussing chamber, an equal distribution of nitrite and nitrate ions should be present in both the compartments, leading to a theoretical value of 50%. Nitrite and nitrate ions were believed to be inert end products of endogenous NO metabolism. However, recently reported results indicate that these products can be in vivo recycled and transformed back into NO, which represents an important unconventional source of NO outside of the classical L-arginine-NO synthase pathway (Lundberg et al., Citation2008). Based on the study by Khan et al. (Citation2011), both nitrite and nitrate ions may be significant precursors of NO and can increase the NO concentration in brain.
Figure 4. Concentrations of GSNO


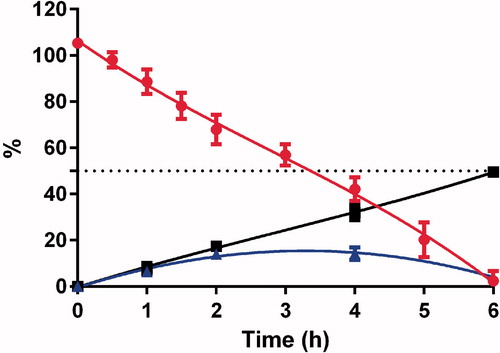
Figure 5. Concentrations of GSNO


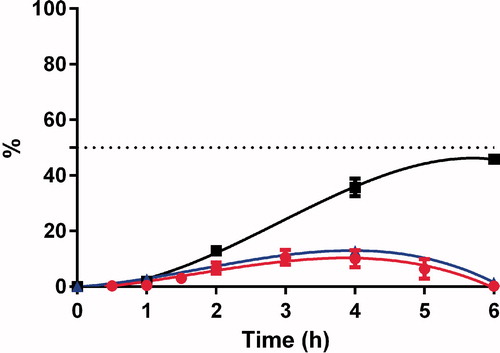
SNA F2 was comparatively more stable than GSNO and finally degraded to nitrite and nitrate ions after 10 h (). These results indicate that the conjugation of alginate to RSNOs can prolong the release of NO from RSNOs. This prolonged release of NO could have potential benefits for the treatment of various diseases. A number of studies have been performed to study the therapeutic benefits of RSNOs in different clinical settings. However, their therapeutic potential is limited due to the poor distribution to the targeted area and rapid release of NO. Therefore, for the development of a more effective NO delivery system, the release rate of NO from RSNOs and its tissue distribution must be properly controlled (Katsumi et al., Citation2005). SNA F2 cannot cross the intestinal barriers but can deliver nitrite and nitrate ions to systemic circulation ( and ).
Figure 6. Concentrations of SNA F2


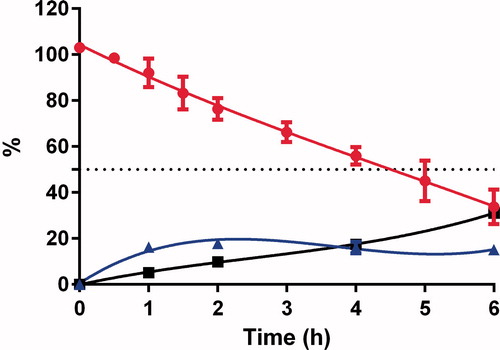
Figure 7. Concentrations of SNA F2


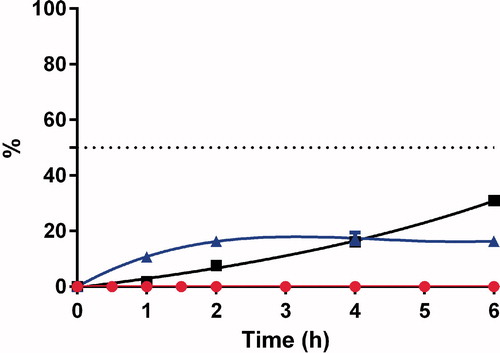
In the treatment of Crohn’s disease, a sustained release of NO is required in the colon, and the systematic absorption is considered a risk for cardiovascular side effects (e.g. hypotension).
Cytotoxicity study using the Caco2 cell line
Caco2 cells were incubated with specified concentrations of SNA F2 () under suitable conditions. The cells were observed before and after incubation with the drug. The MTT test indicated that SNA F2 with less than 50 μM NO exhibited a viability of more than 100% and 100 μM SNA F2 exhibited 86% viability. However, SNA F2 with 200 μM NO was cytotoxic, and the viability of cells decreases to 38%.
Figure 8. Cell viability of Caco2 cell line after exposure to different concentrations of alginate, alginate-GSH conjugate and SNA F2 (n = 3, mean ± SD). For SNA F2, the concentrations are expressed in μM of NO; for the other polymers, corresponding concentration are used. *Kruskal–Wallis test (comparison with alginate): p < 0.05.
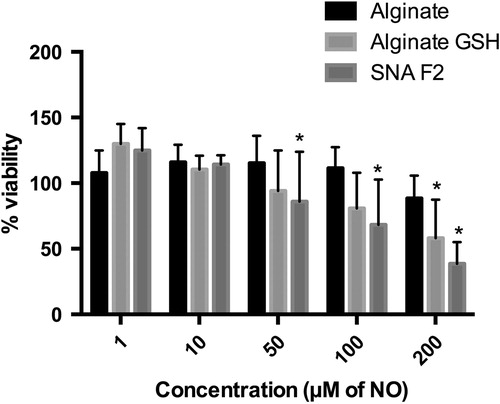
Based on our results, alginate alone increased the viability of the cells even in the column “100 μM of NO”. In addition, the alginate-GSH conjugate exhibits 100% viability in the column “50 μM of NO”. However, its viability decreased at higher concentrations. Therefore, based on this MTT assay, our polymer was not toxic at 10 μM NO and exhibits 100% metabolic activity in the cells after 24 h of incubation. Similar results were obtained in a study performed by Ronzani et al. (Citation2014) and Safar et al. (Citation2015), where empty nanoparticles consisting of Eudragit RL were seeded into the human THP-1 monocytic cell line, and a significant increase in the viability of the cells up to 40% was observed compared with the control. The cell viability increased due to an increase in the metabolic activity and growth of the cells after contact with the nanoparticles, and this phenomenon was related to an augmentation of the mitochondrial volume and activity. The mitochondrial activity may be related to cell proliferation, which leads to an increase in the mitochondrion number. The upregulation of genes involved in metabolism and proliferation may be related to this increased mitochondrial activity, which belongs to “transcription”, “nucleus”, “chromosomal part”, “metabolic process”, and “proliferation” (Safar et al., Citation2015).
Conclusions
Based on our results, a novel polymeric NO-donor was successfully synthesized by linking alginate to GSH followed by a nitrosation step to link NO. The new donor was able to carry a significant amount of pharmacologically active NO with an interesting “extended release” effect. A stable NO-donor under physiologically acceptable pH conditions was achieved, and this donor has the potential for long-term therapeutic effects. Alginate is a good candidate for the long-term stability and controlled delivery of NO. The low toxicity of this new compound suggests future in vivo studies in animal models should be performed. This type of compound would be beneficial for the treatment of various types of diseases including Crohn’s disease. The release of NO may differ under physiological conditions, especially due to the concentration of O2, which is necessary for tissue viability. This concentration may be lower in the colonic lumen.
We believe that our compound can be encapsulated in gastro-resistant soft gelatine capsules for oral delivery. Microparticles of SNA with chitosan can also be prepared (Marcato et al., Citation2013). In addition, a polyelectrolyte complex of alginate with chitosan and Eudragit® L100-55 can also be prepared (Calija et al., Citation2013). Animal models will be used in future to evaluate the in vivo potential of the new compound and to study its pharmacokinetics. Furthermore, this type of conjugation will be tried for other biodegradable polymers.
Acknowledgements
SU Shah wishes to acknowledge funding support by the Higher Education Commission of Pakistan.
Declaration of interest
The authors have no conflict of interest.
References
- Abraham C, Cho JH. (2009). Inflammatory bowel disease. N Engl J Med 361:2066–78
- Appenzeller-Herzog C. (2011). Glutathione- and non-glutathione-based oxidant control in the endoplasmic reticulum. J Cell Sci 124:847–55
- Atyabi F, Moghaddam FA, Dinarvand R, et al. (2008). Thiolated chitosan coated poly hydroxyethyl methacrylate nanoparticles: synthesis and characterization. Carbohydr Polym 74:59–67
- Bernkop-Schnurch A, Kast CE, Richter MF. (2001). Improvement in the mucoadhesive properties of alginate by the covalent attachment of cysteine. J Control Release 71:277–85
- Calija B, Cekic N, Savic S, et al. (2013). pH-sensitive microparticles for oral drug delivery based on alginate/oligochitosan/Eudragit(®) L100-55 “sandwich” polyelectrolyte complex. Colloids Surf B Biointerfaces 110:395–402
- Everett TR, Wilkinson IB, Mahendru AA, et al. (2014). S-Nitrosoglutathione improves haemodynamics in early-onset pre-eclampsia. Br J Clin Pharmacol 78:660–9
- Freeman HJ. (2009). Long-term natural history of Crohn’s disease. World J Gastroenterol 15:1315–18
- Friedman A, Friedman J. (2009). New biomaterials for the sustained release of nitric oxide: past, present and future. Expert Opin Drug Deliv 6:1113–22
- Furuhashi S, Sugita H, Takamori H, et al. (2012). NO donor and MEK inhibitor synergistically inhibit proliferation and invasion of cancer cells. Int J Oncol 40:807–15
- Georgii JL, Amadeu TP, Seabra AB, et al. (2011). Topical S-nitrosoglutathione-releasing hydrogel improves healing of rat ischaemic wounds. J Tissue Eng Regen Med 5:612–19
- Heines SV. (1958). Peter Griess – discoverer of diazo compounds. J Chem Educ 35:187
- Hornyak I, Marosi K, Kiss L, et al. (2012). Increased stability of S-nitrosothiol solutions via pH modulations. Free Radic Res 46:214–25
- Ignarro LJ, Lippton H, Edwards JC, et al. (1981). Mechanism of vascular smooth muscle relaxation by organic nitrates, nitrites, nitroprusside and nitric oxide: evidence for the involvement of S-nitrosothiols as active intermediates. J Pharmacol Exp Ther 218:739–49
- Jerca L, Jerca O, Constantinescu I. (2002). Mechanism of action and biochemical effects of nitric oxide (NO). J Prev Med 10:35–45
- Josephy PD, Rehorek D, Janzen EG. (1984). Electron spin resonance spin trapping of thiyl, radicals from the decomposition of thionitrites. Tetrahedron Lett 25:1685–8
- Katsumi H, Nishikawa M, Ma SF, et al. (2004). Physicochemical, tissue distribution, and vasodilation characteristics of nitrosated serum albumin: delivery of nitric oxide in vivo. J Pharm Sci 93:2343–52
- Katsumi H, Nishikawa M, Yamashita F, et al. (2005). Development of polyethylene glycol-conjugated poly-S-nitrosated serum albumin, a novel S-nitrosothiol for prolonged delivery of nitric oxide in the blood circulation in vivo. J Pharmacol Exp Ther 314:1117–24
- Khan M, Im YB, Shunmugavel A, et al. (2009). Administration of S-nitrosoglutathione after traumatic brain injury protects the neurovascular unit and reduces secondary injury in a rat model of controlled cortical impact. J Neuroinflamm 6:32. doi:10.1186/1742-2094-6-32
- Khan M, Sakakima H, Dhammu TS, et al. (2011). S-nitrosoglutathione reduces oxidative injury and promotes mechanisms of neurorepair following traumatic brain injury in rats. J Neuroinflamm 8:78. doi: 10.1186/1742-2094-8-78
- Kilbourn RG. (1996). Nitric oxide: moving towards the clinic. Mol Med Today 2:324
- Lundberg JO, Weitzberg E, Gladwin MT. (2008). The nitrate-nitrite-nitric oxide pathway in physiology and therapeutics. Nat Rev Drug Discov 7:156–67
- Mann HB, Whitney DR. (1947). On a test of whether one of two random variables is stochastically larger than the other. Ann Math Statist 18:50–60
- Marcato PD, Adami LF, de Melo Barbosa R, et al. (2013). Development of a sustained-release system for nitric oxide delivery using alginate/chitosan nanoparticles. Curr Nanosci 9:1–7
- Moncada S, Palmer RM, Higgs EA. (1991). Nitric oxide: physiology, pathophysiology, and pharmacology. Pharmacol Rev 43:109–42
- Parent M, Dahboul F, Schneider R, et al. (2013). A complete physicochemical identity card of S-nitrosoglutathione. Curr Pharm Anal 9:31–42
- Ronzani C, Safar R, Diab R, et al. (2014). Viability and gene expression responses to polymeric nanoparticles in human and rat cells. Cell Biol Toxicol 30:137–46
- Safar R, Ronzani C, Diab R, et al. (2015). Human monocyte response to S-nitrosoglutathione-loaded nanoparticles: uptake, viability, and transcriptome. Mol Pharm 12:554–61
- Saraiva J, Marotta-Oliveira SS, Cicillini SA, et al. (2011). Nanocarriers for nitric oxide delivery. J Drug Deliv 2011:936438
- Savidge TC, Newman P, Pothoulakis C, et al. (2007). Enteric glia regulate intestinal barrier function and inflammation via release of S-nitrosoglutathione. Gastroenterology 132:1344–58
- Saville B. (1958). A scheme for the colorimetric determination of microgram amounts of thiols. Analyst 83:670–2
- Schulz K, Kerber S, Kelm M. (1999). Reevaluation of the Griess method for determining NO/NO2− in aqueous and protein-containing samples. Nitric Oxide 3:225–34
- Seabra AB, da Silva R, de Oliveira MG. (2005). Polynitrosated polyesters: preparation, characterization, and potential use for topical nitric oxide release. Biomacromolecules 6:2512
- Servetnyk Z, Jiang S, Hjelte L, et al. (2011). The effect of S-nitrosoglutathione and L-cysteine on chloride efflux from cystic fibrosis airway epithelial cells. Exp Mol Pathol 90:79–83
- Shah SU, Martinho N, Socha M, et al. (2015). Synthesis and characterization of S-nitrosoglutathione-oligosaccharide-chitosan as a nitric oxide donor. Exp Opin Drug Deliv 12:1209–23
- Shin HY, George SC. (2001). Microscopic modeling of NO and S-nitrosoglutathione kinetics and transport in human airways. J Appl Physiol 90:777–88
- Singh RJ, Hogg N, Joseph J, et al. (1996a). Mechanism of nitric oxide release from S-nitrosothiols. J Biol Chem 271:18596–603
- Singh SP, Wishnok JS, Keshive M, et al. (1996b). The chemistry of the S-nitrosoglutathione/glutathione system. Proc Natl Acad Sci USA 93:14428–33
- Sosnik A. (2014). Alginate particles as platform for drug delivery by the oral route: state-of-the-art. ISRN Pharm 2014:17
- Thomsen OO, Cortot A, Jewell D, et al. (1998). A comparison of budesonide and mesalamine for active Crohn’s disease. International Budesonide-Mesalamine Study Group. N Engl J Med 339:370–4
- Tonnesen HH, Karlsen J. (2002). Alginate in drug delivery systems. Drug Dev Ind Pharm 28:621–30
- Wolfe DL, Forland SC, Benet LZ. (1973). Drug transfer across intact rat intestinal mucosa following surgical removal of serosa and muscularis externa. J Pharm Sci 62:200–5
- Yousefpour P, Atyabi F, Dinarvand R, et al. (2011). Preparation and comparison of chitosan nanoparticles with different degrees of glutathione thiolation. Daru 19:367–75