Abstract
In this study, mesoporous silica particles with a hexagonal structure (SBA-15) were synthesized and modified with (3-aminopropyl) triethoxysilane, and used as a carrier for anti-inflammatory drug, betamethasone sodium phosphate. Drug-loaded silica particles were grafted on the cotton fabric surface using chitosan and polysiloxane reactive softener as a soft and safe fixing agent to develop an antibacterial cotton fabric with drug delivery properties. Cytometry assays revealed that synthesized silica have no cytotoxicity against human peripheral blood mononuclear cells. Accordingly, the produced drug-loaded nanostructures can be applied via different routes, such as wound dressing. Drug delivery profile of the treated fabrics were investigated and compared. The drug release rate followed the conventional Higuchi model. The treated cotton fabrics were tested and evaluated using scanning electron microscope images, bending length, air permeability, washing durability and anti-bacterial properties. It was found that the chitosan-/softener-treated fabrics compounded with drug-loaded silica particles have a good drug delivery performance and exhibited a powerful antibacterial activity against both Escherichia coli and Staphylococcus aureus even after five washing cycles. The produced antibacterial cotton fabric with drug delivery properties could be proposed as a suitable material for many medical and hygienic applications.
Introduction
Recent strategies focused on the acceleration of wound healing using biomaterials such as chitosan (Cui et al., Citation2011; Jayakumar et al., Citation2011; Moura et al., Citation2013). Chitosan is a polysaccharide composed of glucosamine and N-acetyl-glucosamine units well known for antibacterial properties. Chitosan in the bulk form has found numerous applications as an antibacterial coating for textiles due to biodegradability, biocompatibility and lack of toxicity (Öktem, Citation2003; Zhang et al., Citation2003; El-Talawy et al., Citation2005; Ye et al., Citation2006; Chun & Gamble, Citation2007; Tayel et al., Citation2011; Hebeish et al., Citation2013; Perelshtein et al., Citation2013). Lou prepared chitosan-coated tencel/cotton nonwoven fabric as a wound dressing (Lou, Citation2008). Rouhani Shirvan et al. have focused on introducing an easy and cost-effective method to fabricate an antibacterial cotton fabric suitable for the medical and hygienic product by means of chitosan (Shirvan et al., Citation2014). Gupta et al. found that chitosan-coated cotton gauze is effective against micro-organisms as quite suitable for wound dressing (Gupta et al., Citation2010). Chitin and chitosan have been examined in a wide variety of biomedical applications, surgical thread, bone healing, and especially wound dressing (Cetin et al., Citation2007; du Plessis et al., Citation2010; Zhang et al., Citation2012). Chitosan accelerates wound healing by speeding the fibroblastic synthesis of collagen in the first few days (Chung et al., Citation1994) and achieves hemostasis and allows the promotion of normal tissue regeneration (William & Herbert, Citation1985). Besides, the biodegradable chitin or chitosan provide bacteriostatic and fungistatic activities (Tomihata & Ikada, Citation1997; Chatterjee et al., Citation2014). Chitosan has been considered as one of the most important biomaterials for wound management in recent years (Lou, Citation2008; Shirvan et al., Citation2014). The excellent properties, together with the very safe profile, make chitosan an exciting and promising compound for the pharmaceutical industry in present and future applications. The chitosan formulation with drugs possibly has dual therapeutic effects thanks to the bioactivities of chitosan itself (Chen & Zhu, Citation2012; Ravi Kumar, Citation2000). Shanmugasundaram et al. have prepared drug-loaded chitosan-coated bamboo gauze. The drug-loaded samples exhibited an excellent antibacterial activity and showed good drug release characteristics (Shanmugasundaram & Mahendra Gowda Citation2011). Additionally, the combination of chitosan with inorganic nanoparticles was an efficient approach to produce antibacterial materials with improved functional properties (Perelshtein et al., Citation2013). Over the past decade, inorganic materials such as silicon have attracted much attention due to their ability to tolerate harsh process conditions (Rajendran et al., Citation2010). Mesoporous silica particles have a high surface area, and are a biocompatible form of silicon (Hong et al., Citation2016), widely employed in biomedical applications including controlled drug delivery, cell tracking bone tissue regeneration and immobilization of proteins or enzymes (Heikkilä et al., Citation2007; Hashemikia et al., Citation2015). They have been proposed as carriers for drug delivery in 2001 (Vallet-Regí et al., Citation2001). However, silanol groups on the pure SBA-15 channel walls simply formed weak intermolecular hydrogen bonds with drugs and released the drug rapidly in the aqueous medium, and need to be modified chemically using processes such as silanization and coating with polymers for controlling drug delivery (Anglin et al., Citation2008; Mohamadnia et al., Citation2015). The combination of polymers with silicon-based materials has generated a large range of novel hybrid materials tailored to apply in localized and systemic drug delivery (Kafshgari et al., Citation2015). Song et al. (Citation2007) loaded bovine serum albumin (BSA) into amino-modified SBA-15 and then encapsulated the micro-particles, via electrostatic assembly, with poly (acrylic acid) (PAA). Trotter et al. (Citation2005) reported the deposition of poly (diallylmethylammonium chloride) and poly (sodium 4-styrenesulfonate) on the surface of negatively charged silica microparticles allowing formation of stable polyelectrolyte films (Trotter et al., Citation2005). The encapsulation can be performed by electrostatic or physiosorption techniques and the drug-release rates can be tuned by the addition of multiple polymeric layers (McInnes & Voelcker, Citation2009). Chen and Zhu wrapped chitosan outside SBA-15 mesoporous silica particles and applied them to control the released drugs (Chen & Zhu, Citation2012).
Textile substrates act as a reservoir of therapeutic agents and released them gradually at the affected site for a period of time. In this article, a novel design of an antibacterial cotton fabric with drug delivery properties compounded with the betamethasone-loaded mesoporous silica particles and chitosan were introduced. Betamethasone sodium phosphate (BSP) is an anti-inflammatory synthetic corticosteroid drug with broad applications in the treatment of itching, redness, dryness, crusting, scaling, inflammation and discomfort of various skin conditions (Arthur et al., Citation2004). To the best of our knowledge, there is no report on chitosan-treated cotton fabric containing mesoporous silica particles loaded with BSP. Several properties of the treated fabrics were studied including drug delivery, bending length, permeability to the air, tensile strength and antibacterial properties.
Experimental
Materials
Tetraethyl orthosilicate (TEOS) and 3-aminopropyl triethoxysilane (APTES), toluene, n-hexane, ethanol, acetic acid and dichloromethane were purchased from Merck Co. (Kenilworth, NJ). Poly (ethylene glycol)-block-poly (propylene glycol)-block-poly (ethylene glycol) [Pluronic P123, [(EO)20(PO)70(EO)20]] and chitosan (MW ∼100000-300000 Da, DD=85%) was provided from Sigma-Aldrich (St. Louis, MO). BSP was obtained from Daroupakhsh Distribution Co. (Tehran, Iran). Polysiloxane reactive softener [Adrasil (P-836)] was obtained from Arghavan Resin (Iran). All the chemicals were used as received without any further purification. A 100% cotton fabric (plain weave, 98 g/m2, Yazdbaf, Tehran, Iran) was used as the substrate for coating. The chemical structures of BSP, and polysiloxane reactive softener are shown in .
Instruments
A thermal oven (Binder, Tuttlingen, Germany) was used to dry and cure the fabric samples. The finishing compounds were dispersed using homogenizer (220 V, 800 W, 40 kHz, Istanbul, Turkey). The scanning electron microscope (SEM) images were taken by a SEM (Philips XL30; Eindhoven, Netherlands). The Shirley stiffness tester was employed to examine the bending length according to ASTM D 1388-96. The tensile strength of the selected fabric was measured in the warp direction using the Instron 5566 tensile testing machine system (MA, USA). Air permeability was measured using the Shirley instrument. A testing head of 5 cm2 was selected for testing the fabric samples on the air permeability tester. A constant air pressure of 100 kPa was maintained for fabric testing on the tester.
Synthesis and functionalization of SBA-15
Mesoporous SBA-15 particles were prepared by using the structure-directing agent of pluronic 123 in acidic condition as described previously (Hashemikia et al., Citation2015; Ghasemnejad et al., Citation2015). The synthesized SBA-15 was then modified through post-grafting with APTES named SBA-15-NH2 (Ghasemnejad et al., Citation2015). The textural parameters of the synthesized SBA-15-NH2 revealed the presence of nanometric pores in the structure of SBA-15-NH2 particles () (Ghasemnejad et al., Citation2015).
Table 1. Structural parameters of SBA-15-NH2 (Ghasemnejad et al., Citation2015).
Drug loading in SBA-15-NH2
BSP, an anti-inflammatory synthetic corticosteroid drug was loaded in optimum conditions via post-impregnation as 30 mg SBA-15-NH2 was poured in 3 ml HCl/KCl buffer (pH = 1.8) containing 51 mg drug [drug (mg)/SBA-15-NH2 (mg) = 1.7]. Finally, the drug-loaded particles were centrifuged at 4000 rpm for 15 min and washed with distilled water followed by drying at 60 °C (Ghasemnejad et al., Citation2015). The loading percentage was measured with UV-Vis absorbance at 243 nm (Ghasemnejad et al., Citation2015). The percentage of drug loading into SBA-15-NH2 was optimized by response surface methodology according to Ghasemnejad et al. (Citation2015). The loading of 33% BSP was achieved under the optimized loading conditions (pH = 1.8 for 3.54 h and drug/silica ratio = 1.7) and the resultant sample was named SBA-15-NH2-BSP (Ghasemnejad et al., Citation2015). The drug loading percentage of the washed sample (DL %) was calculated using Equation (Equation1(1) ).
(1)
The characterization of the SBA-15-NH2 particles has been studied by Ghasemnejad et al. (Citation2015).
Flow cytometry
Flow cytometry provides a rapid and reliable method to quantify viable cells in a cell suspension. Determination of cell viability is critical when evaluating the response to cytotoxic drugs or other environmental factors. Cell viability assay was performed by Annexin-PI kit (Sc-45552, Santa Cruz, TX) according to manufacture recommendation for suspension cells. Briefly, 105 human peripheral blood mononuclear cells (PBMCs) were treated with 50 μg/ml SBA-15-NH2, SBA-15-NH2-BSP and were incubated with 10 µl propidium iodide (PI). The samples were incubated at room temperature for 24 h and then 400 µl 1 × assay buffer was added to each sample that was conducted using a single laser emitting light at 488 nm for FITC.
Treatment of cotton fabric
The bleached cotton fabric samples were washed with 1 g/l nonionic detergent at 60 °C for 20 min, rinsed with cold water and dried at 100 °C for 10 min. The samples have been prepared according to . SBA-15-NH2-BSP particles were first dispersed using an ultrasonic bath (L:G = 50:1), and then, cotton fabric was immersed in the prepared bath for 5 min, padded with a wet pick-up of 100% and dried at 70 °C. The silica-loaded fabrics were then treated with polysiloxane reactive softener and/or chitosan dried at 100 °C for 30 min followed by curing at 150 °C for 5 min. Samples S2, S3 and S4 were prepared with two-step processing. In order to study the effect of two-step process, samples S5 and S6 were prepared through one-step processing and all the reagents have been applied on them in a one bath. Furthermore, to study the effect of SBA-15-NH2 on drug delivery properties of the cotton, samples S7 and S8 has been treated with BSP without SBA-15-NH2 particles.
Table 2. Preparation of various fabric samples.
In vitro release profile
The release profile of SBA-15-NH2-BSP particles and treated cotton fabrics was determined dynamically. The samples were put in an interpenetrating membrane (D9527 dialysis tubing cellulose membrane, MWCO 14 000, Sigma–Aldrich Co.) and immersed in 80 ml phosphate buffer saline solution in pH ∼ 7.2–7.4 at 37 °C under stirring with 100 rpm. At appropriate time intervals, 4 ml aliquots were taken and replaced with the same volume of the fresh medium. Drug concentrations were determined using UV-Vis at 243 nm.
Anti-bacterial activity
Quantitative anti-bacterial efficiency of the treated fabric was determined against Staphylococcus aureus (ATCC 6538) as Gram-positive and Escherichia coli (ATCC 8739) as Gram-negative bacteria according to AATCC100. The antibacterial efficiency was calculated according to Equation (Equation2(2) ) (Harifi & Montazer, Citation2014).
(2)
where R is the reduction rate, A and B are the number of bacterial colonies from the control and treated samples.
Results and discussions
Cytotoxicity assay
Ten thousand PBMCs were gated by the FACS Calibur instrument (BD Biosciences, Haryana, India) and data were analyzed using FCS express software (BD Biosciences, Haryana, India) for PI exclusion as a marker of cell viability for three groups including control, 50 μg/ml SBA-15-NH2 and SBA-15-NH2-BSP using flow cytometry (FACS Calibur). After 24-h incubation with the particles, PBMCs were found to be viable 100% relative to the control cells at the particle concentration of 50 μg/ml indicating that the synthesized particles, have no cytotoxicity against PBMCs (). There were no significant differences between two treatments related to the control group using ANOVA and LSD multiple comparison tests (α ≤ 0.05). Thus, the produced drug-loaded nanostructures are safe and can be used for drug delivery systems as demonstrated by others (López et al., Citation2006; de Sousa Azevedo et al., Citation2012; Ghasemnejad et al., Citation2015).
Drug release
The release profile of BSP from various samples in phosphate buffer saline (PBS) solution at pH∼7.2–7.4 was analyzed using UV-Vis spectra (). The results of sample S5 and S3 confirmed the importance of the two-step processing as the particles were first dispersed in n-hexane to avoid the drug release in processing. However, in one-step processing, SBA-15-NH2-BSP particles have been dispersed in the solution of chitosan/acetic acid. In the two-step processing, the particles with higher polarity than nonpolar solvent (n-hexane) tend to attach to the cotton surface with a higher polarity rather than n-hexane. However, in one-step processing, the particles have low tendency to attach to the cotton surface and remain in the treatment bath. Thus, the drug content of the two-step treated samples (S3 and S4) was higher than the one-step treated samples (S5 and S6).
Figure 3. (a) Drug release profile of treated cotton samples and (b) Higuchi model of BSP release from sample S2 and S4.
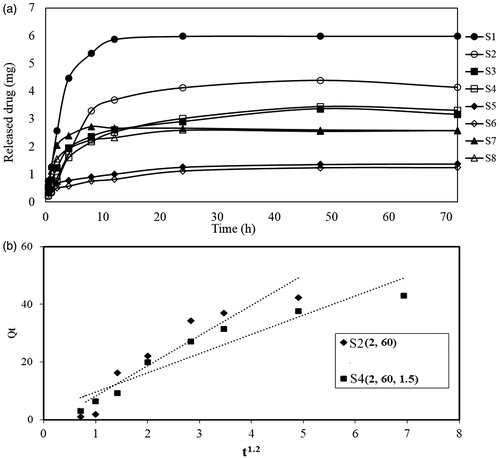
The results in reveal that application of softener and chitosan inhibit the burst release of the drug from mesoporous silica. Chen & Zhu (Citation2012) demonstrated that the layer of chitosan on nano-carriers is adsorbed by hydrogen bonding between chitosan, SBA-15-NH2 and hydroxyl groups of cotton fabric. Through enclosing the mesoporous silica particles, chitosan inhibits the drug release from the mesoporous channels of silica. Further, silicone-based softener forms a breathable cross-linked film on the surface of the cotton fabric and reacts with the free hydroxyl groups of the cellulose, silica particles and chitosan (Montazer & Hashemikia, Citation2012). Thus, application of chitosan and softener lead to a lower release rate of BSP from mesoporous silica. Comparing the release profile of samples S3 and S5 with S7 confirm the positive effect of SBA-15-NH2 on the release profile of the treated samples. The treatment without SBA-15-NH2 particles leads to a high release rate of BSP and prolonged for 24 h only.
The sustained drug release properties of the biocompatible mesoporous silica can be useful for application in medical textile. A significant drug has been released from sample S3 and S4 for 48 h as demonstrated in . Thus, treatment of cotton fabric with chitosan and drug-loaded mesoporous silica particles leads to develop a cotton fabric with anti-inflammatory drug delivery properties.
The drug loading in SBA-15-NH2-BSP particles was 33% and the add-on% of SBA-15-NH2-BSP particles on samples in the prime processing was 4.6%. Based on Equation (Equation1(1) ), 10.9 mg betamethasone was loaded on 1 g treated cotton fabric. Accordingly, from the release profile of sample S1, S2 and S4, it is estimated that 54%, 40% and 32% of the loaded drug has been released from the samples, respectively.
The possible reactions of chitosan, silicon softener with synthesized silica particles and/or cellulose, and the reaction of cellulose with silica particles by means of silicon softener are presented in reactions 1–4.
Most of the works on the drug release kinetics from mesoporous carriers are explained using the Higuchi model due to chemical and physical entrapment of the drug (Wang, Citation2009). Thus, the drug release from mesoporous materials is diffusion control similar to other drug delivery systems such as biopolymers or hydrogels (Hashemikia et al., Citation2015). Higuchi was the first to derive an equation to describe the release of a drug from an insoluble matrix as the square root of a time-dependent process based on Fickian diffusion (Equation 5).
(3)
where Qt is the drug released in time t and KH is the release rate constant for the Higuchi model (Hashemikia et al., Citation2015).
Here, the kinetic parameters of BSP release from cotton fabric are evaluated by the Higuchi model. The results of Higuchi model for sample S2 and S4 agreed with the experimental data for drug release as shown in and . The value of KH revealed higher release rate of sample S2 compared with S4. The results confirm the inhibition effect of chitosan on drug delivery rate of treated cotton fabric.
Table 3. BSP release kinetic parameters for Sample S2 and S4.
Bending length
The fabric handle can be assessed by measuring the bending length of the fabric. The lower bending length means lower rigidity or better softness and fabric drape. From , the bending length of sample S1 treated with SBA-15-NH2-BSP without softener is slightly higher than the control one. Sample S2 has lower bending length. This is because, application of silicone softener leads to lower bending length due to the flexibility of the siloxane chain and free rotation around Si–O bonds (Salemone, Citation1996; Montazer & Hashemikia, Citation2012). Silicone finishes are widely recognized as the best material for increasing fabric softness, special unique hand and elastic resilience. In order to improve the fabric handle, a silicone-based softener was employed to form cross-linked soft film on the fiber surfaces. The positive effect of the silicone softener is more relevant for sample S4 treated with chitosan and softener. Sample S4 has a lower bending length compared with sample S3 treated with chitosan without softener. Therefore, introducing a silicone-based softener to the chitosan-treated cotton fabric containing silica particles prevents the hardening effect of the chitosan and particles entrapping them within the cross-linked formed layer.
Table 4. Bending length of the treated samples.
Scanning electron microscope images
indicates a rope-like morphology with a relative length of ∼1 μm for SBA-15-NH2-BSP particles (Ahmadi et al., Citation2014). The SEM image of the control sample shows the clean cotton fibers surface without impurities (). reveals the presence of considerable amounts of SBA-15-NH2-BSP particles on the surface of sample S2 and S4.
Figure 4. SEM images of sample SBA-15-NH2-BSP particles (a), untreated cotton fabric (b), sample S2 (c and d) and sample S4 (e and f).
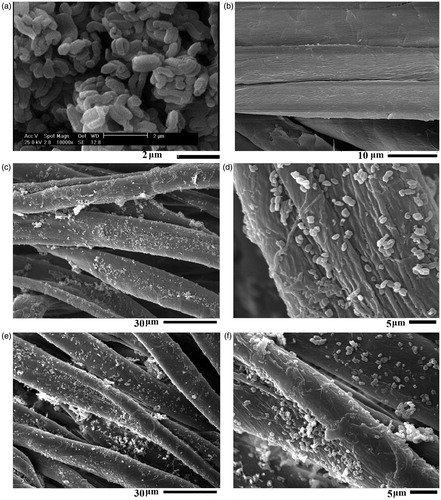
To evaluate the washing durability of the treated fabric, sample S4 was washed 5 times at 60 °C for 20 min with nonionic detergent. The SEM images of the washed fabric confirmed the washing durability of sample S4 after five washings (). The SEM image showed the presence of a significant amount of silica particles on the surface of the fibers after washing. The silicone softener acts as a soft resin and reacts with free hydroxyl groups of the cellulose, chitosan and silica particles (reactions 1–4) to bind the particles to the fabric surface preventing the separation from the fabric (Montazer & Hashemikia, Citation2012). Cytotoxic assays revealed the nontoxic properties of SBA-15-NH2 particles against the blood cell. Thus, they are not harmful if silica particles are released from the textile fabric.
Tensile strength
Skin is a viscoelastic material which is permanently subjected to slight stress. The tensile strength of human skin is reported to be in the region of 1.8 (N/mm2) (Uppal et al., Citation2011). Any wound dressing developed ought to have tensile strength higher than this value. Otherwise, with slight stress at the near vicinity of the wound, the dressing is likely to rupture (Chellamani et al., Citation2013, Citation2014).
To evaluate fabric resistance to stretching or pulling force, the fabric strip test investigated the effect of treatment on fabric breaking. The tensile strength of the fabrics was measured in the warp direction and the results are indicated in . The higher tensile strength belongs to the untreated and S1 samples. Comparing the results of sample S1 and S3 with S2 and S4 revealed the application of softener resulted in a loss of maximum load due to reduction of the friction between the fibers in a yarn and between fibers within the fabric which caused more slippage between the fibers and decreased the load capacity (Chattopadhyay & Vyas, Citation2010). Further, considering the pH of silicone softener ∼5.5 and curing at 150 °C on the cotton fabric leads to cellulosic chain degradation in acidic media at high temperature producing lower fabric tensile strength (Nazari et al., Citation2009; Reddy et al., Citation2009; Nazari et al., Citation2010; Hashemikia & Montazer, Citation2012). In the case of the chitosan-treated sample (S3, S4) owing to the damage to cotton resulting from the acid media finishing, the tensile strength of the treated cotton samples decreased (Chung et al., Citation1998; Ye et al., Citation2006). However, all the fabric samples still maintained at least 75% of the original tensile strength (Chung et al., Citation1998).
Table 5. Tensile strength of different sample.
Air permeability
The air permeability is a quantitative measurement of how well a material allows the passage of air through it. The air permeability is one of the important parameters of wound dressing, which prevents maceration and gives better comfort to the patients. In a recent in vivo study on wound healing and antibacterial performance of electrospun nanofiber membranes, researchers concluded that the porosity and air permeability characteristics have a strong influence on facilitating the wound healing, especially at the early healing stages (Liu et al., Citation2010; Uzun et al., Citation2013). For faster healing, wounds have to be well oxygenated, which depends upon the air permeability of the dressing (Vittoria, Citation1999; Ghosh & Jassal, Citation2002; Uzun et al., Citation2013). Thus, a dressing with more air permeability increases the efficacy of the dressing (Uppal et al., Citation2011). The results of air permeability of the treated fabrics are shown in . The air permeability of the treated fabrics is lower than the control one. Chitosan, softener and silica particles cover the fabric pores and inhibit the air passage through the fabric. Therefore, a decrease in air permeability reveals the presence of the reagent on the treated fabrics. However, Uppal et al. (Citation2011) revealed that the air permeability of the gauze with Vaseline is 322 m3/h/m2. Accordingly, the decrease in air permeability of the treated fabrics was not high to inhibit the air passage.
Table 6. Air permeability of different sample.
Anti-bacterial properties
Chitosan has been examined to be used in a wide variety of biomedical applications, such as drug delivery carriers and wound dressing (William & Herbert, Citation1985; Chung et al., Citation1994; Cetin et al., Citation2007; du Plessis et al., Citation2010; Zhang et al., Citation2012). Chitosan accelerates the resistance of wound by speeding the fibroblastic synthesis of collagen in the first few days of wound healing (Öktem, Citation2003) and allows the promotion of normal tissue regeneration (William & Herbert, Citation1985). Besides, chitosan provides bacteriostatic and fungistatic activities (Tomihata & Ikada, Citation1997; Chatterjee et al., Citation2014). Therefore, it is one of the most important biomaterials for wound management.
The effect of treatment on the antibacterial activity against E. coli as Gram-negative bacteria and S. aureus as Gram-positive bacteria are shown in . The antibacterial activity was evaluated by assessing the reduction in bacterial growth on the treated cotton fabrics ( and ). Comparing anti-bacterial properties of control, S1 and S2 with S3 and S4 indicated the anti-bacterial properties of chitosan against E. coli and S. aureus related to the physicochemical properties of chitosan (López et al., Citation2006; Harifi & Montazer, Citation2014; Kim et al., Citation2015). Samples S1 and S2 have no antibacterial efficiency and the antibacterial properties of samples S3 and S4 are related to chitosan treatment. Three antibacterial mechanisms have been proposed for chitosan in the literatures: (i) the ionic surface interaction resulting in wall cell leakage, (ii) the inhibition of mRNA and protein synthesis via the penetration of chitosan into the nuclei of the microorganisms and (iii) formation of an external barrier, chelating metals and provoking the suppression of essential nutrients to microbial growth. It is likely that all the mentioned events occur simultaneously, however at different intensities (Goy et al., Citation2009).
Table 7. Anti-bacterial properties of control and samples S1, S2, S3, S4 and S4 after five washing cycles against E. coli and S. aureus.
To investigate the washing fastness, anti-bacterial properties of S4 after five washings were tested. The results of anti-bacterial properties of the washed samples confirmed the washing durability of chitosan even after five washings.
Conclusion
Textile substrates act as reservoir of therapeutic agents and release them gradually at the affected site for a period of time, however relatively few commercially wound dressings are available incorporated with these materials.
The functional cotton fabric was prepared by modification with betamethasone-loaded SBA-15-NH2 particles, chitosan and silicone softener. SEM images confirmed the presence of silica particles on the surface of cotton fibers. The profile of BSP release from the treated cotton fabrics in PBS buffer media revealed that chitosan and softener covering the surface of mesoporous channels of SBA-15-NH2-BSP particles led to a gradual release profile within 48 h. Further, the drug release rate followed the conventional Higuchi model. The chitosan-treated fabrics exhibited excellent anti-bacterial properties against E. coli and S. aureus preserved after five washings. Accordingly, although the application of chitosan lead to decrease in drug release content of the treated fabric, the great antibacterial properties and lower drug release rate (until 48 h) of the chitosan-treated fabrics justified the reason of application of this biopolymer on the cotton fabric. The tensile strength and air permeability decrease indicate the presence of silica/chitosan/softener coating on the fabric surface. Further, using the silicone softener reduced the fabric stiffness along with lower fabric bending length. Therefore, by formation of a breathable self-cross-linked film on the fabric surface, the silicone-based softener, preventing the hardening effect of the particles and chitosan, entrapping them within the cross-linked formed layer and increasing the washing durability.
Acknowledgements
The authors wish to thank Dr. Abbas Bahari, Assistant Professor of Biotechnology at Research Institute of Modern Biological Techniques, University of Zanjan, Iran, for his kind help in Cytotoxicity studies.
Declaration of interest
The authors report no conflicts of interest. The authors alone are responsible for the content and writing of this article.
References
- Ahmadi E, Dehghannejad N, Hashemikia S, et al. (2014). Synthesis and surface modification of mesoporous silica nanoparticles and its application as carriers for sustained drug delivery. Drug Deliv 21:164–72
- Anglin EJ, Cheng L, Freeman WR, Sailor MJ. (2008). Porous silicon in drug delivery devices and materials. Adv Drug Deliv Rev 60:1266–77
- Arthur KE, Wolff JC, Crrier DJ. (2004). Analysis of betamethasone, dexamethasone and related compounds by liquid chromatography/electrospray mass spectrometry. Rapid Commun Mass Spectrom 18:678–84
- Cetin M, Aktas Y, Vural I, et al. (2007). Preparation and in vitro evaluation of bFGF-loaded chitosan nanoparticles. Drug Deliv 14:525–9
- Chatterjee S, Chatterjee BP, Guha AK. (2014). A study on antifungal activity of water-soluble chitosan against Macrophomina phaseolina. Int J Biol Macromol 67:452–7
- Chattopadhyay DP, Vyas DD. (2010). Effect of silicone nano-emulsion softener on physical properties of cotton fabric. Indian J Fibre Text 35:68–71
- Chellamani KP, Vignesh Balaji RS, Veerasubramanian D. (2014). Quality evaluation methods for textile substrates based wound dressings. IJETAE 4:811–17
- Chellamani KP, Vignesh Balaji RS, Sathish J. (2013). Spunlace wound dressing using bamboo fibres. Asian Text J 22:69–79
- Chen F, Zhu Y. (2012). Chitosan enclosed mesoporous silica nanoparticles as drug nano-carriers: Sensitive response to the narrow pH range. Micropor Mesopor Mat 150:83–9
- Chun DTW, Gamble GR. (2007). Using the reactive dye method to covalently attach antibacterial compounds to cotton. J Cotton Sci 11:154–8
- Chung LY, Schmidt RJ, Hamlyn PF, et al. (1994). Biocompatibility of potential wound management products: fungal mycelia as a source of chitin/chitosan and their effect on the proliferation of human F1000 fibroblasts in culture. J Biomed Mater Res 28:463–9
- Chung YS, Lee KK, Kim JW. (1998). Durable press and antimicrobial finishing of cotton fabrics with a citric acid and chitosan treatment. Text Res J 68:772–5
- Cui F, Li G, Huang J, et al. (2011). Development of chitosan-collagen hydrogel incorporated with lysostaphin (CCHL) burn dressing with anti-methicillin-resistant Staphylococcus aureus and promotion wound healing properties. Drug Deliv 18:173–80
- de Sousa Azevedo RC, Soares DCF, de Sousa RG, et al. (2012). Multifunctional nanostructured materials applied in controlled radiopharmaceuticals release. Nanobiotechnology 3:163–8
- du Plessis LH, Kotzé AF, Junginger HE. (2010). Nasal and rectal delivery of insulin with chitosan and N-trimethyl chitosan chloride. Drug Deliv 17:399–407
- El-Talawy KF, El-Bendary MA, Elhendawy AG, Hudson SM. (2005). The antimicrobial activity of cotton fabrics treated with different crosslinking agents and chitosan. Carbohydr Polym 60:421–30
- Ghasemnejad M, Ahmadi E, Mohamadnia Z, et al. (2015). Functionalized silica nanoparticles as a carrier for betamethasone sodium phosphate: Drug release study and statistical optimization of drug loading by response surface method. Mat Sci Eng C 56:223–32
- Ghosh S, Jassal M. (2002). Use of polysaccharide fibers for modern wound dressing. Indian J Fiber Text Res 27:434–50
- Goy RC, de Britto D, Assis OBG. (2009). A review of the antimicrobial activity of chitosan. Polímeros 19:241–7
- Gupta B, Agarwal R, Alam MS. (2010). Textile based smart wound dressing. Ind J Fibre Text 35:174–87
- Harifi T, Montazer M. (2014). Photo-, bio-, and magneto-active colored polyester fabric with hydrophobic/hydrophilic and enhanced mechanical properties through synthesis of TiO2/Fe3O4/Ag nanocomposite. Ind Eng Chem Res 53:1119–29
- Hashemikia S, Hemmatinejad N, Ahmadi E, Montazer M. (2015). Optimization of tetracycline hydrochloride adsorption on amino modified SBA-15 using response surface methodology. J Colloid Interf Sci 443:105–14
- Hashemikia S, Montazer M. (2012). Sodium hypophosphite and nano TiO2 inorganic catalysts with citric acid on textile producing multi-functional properties. Appl Catal A-Gen 417–418:200–8
- Hebeish A, Sharaf S, Farouk A. (2013). Utilization of chitosan nanoparticles as a green finish in multifunctionalization of cotton textile. Int J Biol Macromol 60:10–17
- Heikkilä T, Salonen J, Tuura J, et al. (2007). Evaluation of mesoporous TCPSi, MCM-41, SBA-15, and TUD-1 materials as API carriers for oral drug delivery. Drug Deliv 14:337–47
- Hong S, Shen S, Cheng D, et al. (2016). High drug load, stable, manufacturable and bioavailable fenofibrate formulations in mesoporous silica: a comparison of spray drying versus solvent impregnation methods. Drug Deliv 23:316–23
- Jayakumar R, Prabaharan M, Sudheesh Kumar PT, et al. (2011). Biomaterials based on chitin and chitosan in wound dressing applications. Biotechnol Adv 29:322–37
- Kafshgari MH, Delalat B, Tong WY, et al. (2015). Oligonucleotide delivery by chitosan-functionalized porous silicon nanoparticles. Nano Res. 8:2033–46
- Kim JO, Noh J-K, Thapa RK, et al. (2015). Nitric oxide-releasing chitosan film for enhanced antibacterial and in vivo wound-healing efficacy. Int J Biol Macromol 79:217–25
- Liu X, Lin T, Fang J. (2010). In vivo wound healing and antibacterial performance of electrospun nanofibre membranes. J Biomed Mater Res A 94:499–508
- López T, Basaldella EI, Ojeda ML, et al. (2006). Encapsulation of valproic acid and sodic phenytoin in ordered mesoporous SiO2 solids for the treatment of temporal lobe epilepsy. Optica Materials 29:75–81
- Lou CW. (2008). Process technology and properties evaluation of a chitosan-coated tencel/cotton nonwoven fabric as a wound dressing. Fiber Polym 9:286–92
- McInnes SJ, Voelcker NH. (2009). Silicon-polymer hybrid materials for drug delivery. Future Med Chem 6:1051–74
- Mohamadnia Z, Ahmadi E, Ghasemnejad M, et al. (2015). Surface modification of mesoporous nanosilica with [3-(2-aminoethylamino) propyl] trimethoxysilane and its application in drug delivery. Int J Nanosci Nanotechnol 11:167–77
- Montazer M, Hashemikia S. (2012). Application of polyurethane/citric acid/silicone softener composite on cotton/polyester knitted fabric producing durable soft and smooth surface. J Appl Polym Sci 124:4141–8
- Moura LI, Dias AM, Carvalho E, de Sousa HC. (2013). Recent advances on the development of wound dressings for diabetic foot ulcer treatment -- a review. Acta Biomater 9:7093–114
- Nazari A, Montazer M, Rashidi A, et al. (2009). Nano TiO2 photo-catalyst and sodium hypophosphite for cross-linking cotton with poly carboxylic acids under UV and high temperature. Appl Catal A-Gen 371:10–16
- Nazari A, Montazer M, Rashidi A, et al. (2010). Optimization of cotton cross-linking with polycarboxylic acids and nano TiO2 using central composite design. J Appl Polym Sci 117:2740–8
- Öktem T. (2003). Surface treatment of cotton fabrics with chitosan. Color Technol 119:241–6
- Perelshtein I, Ruderman E, Perkas N, et al. (2013). Chitosan and chitosan–ZnO-based complex nanoparticles: formation, characterization, and antibacterial activity. J Mater Chem B 1:1968–76
- Rajendran R, Balakumar C, Ahammed HAM, et al. (2010). Use of zinc oxide nano particles for production of antimicrobial textiles. Int J Eng Sci Tech 2:202–8
- Ravi Kumar MNV. (2000). A review of chitin and chitosan applications. React Funct Polym 46:1–27
- Reddy N, Li Y, Yang Y. (2009). Alkali-catalyzed low temperature wet crosslinking of plant proteins using carboxylic acids. J Biotechnol 25:139–46
- Salemone JC. Polymeric materials encolpedia, 2nd edn, Vol. 1. Boca Raton: CRC Press; 1996, 218
- Shanmugasundaram OL, Mahendra Gowda RV. (2011). Development and characterization of bamboo gauze fabric coated with polymer and drug for wound healing. Fiber Polym 12:15–20
- Shirvan AR, Hemmatinejad N, Bashari A. (2014). Antibacterial finishing of cotton fabric via the chitosan/TPP self-assembled nano layers. Fiber Polym 15:1908–14
- Song SW, Hidajat K, Kawi S. (2007). pH controllable drug release using hydrogel encapsulated mesoporous silica. Chem Commun 14:4396–8
- Tayel AA, Moussa SH, El-Tras WF, et al. (2011). Antimicrobial textile treated with chitosan from Aspergillus niger mycelial waste. Int J Biol Macromol 49:241–5
- Tomihata K, Ikada Y. (1997). In vitro and in vivo degradation of films of chitin and its deacetylated derivatives. Biomaterials 18:567
- Trotter H, Zaman A, Partch R. (2005). Preparation and characterization of polymer composite multilayers on SiO2. J Colloid Interf Sci 286:233–8
- Uppal R, Ramaswamy GN, Arnold C, et al. (2011). Hyaluronic acid nanofiber wound dressing–production, characterization, and in vivo behavior. J Biomed Mater Res B 97:20–9
- Uzun M, Anand SC, Shah T. (2013). In vitro characterization and evaluation of different types of wound dressing materials. Int J Biomed Eng Technol 1:1–7
- Vallet-Regí M, Ramila A, Del Real RP, Perez-Pariente J. (2001). A new property of MCM-41: Drug delivery system. Chem Mater 13:308–11
- Vittoria PL. (1999). Principles of selecting the right wound dressing. Medsurg Nurs 8:267–70
- Wang S. (2009). Ordered mesoporous materials for drug delivery. Micropor Mesopor Mat 117:1–9
- William GM, Herbert JQ. (1985). U.S. Patent, 4532134
- Ye W, Xin JH, Lee PLKLD, Kwong TL. (2006). Durable antibacterial finish on cotton fabric by using chitosan-based polymeric core-shell particles. J Appl Polym Sci 102:1787–93
- Zhang Z, Chen L, Ji J, et al. (2003). Antibacterial properties of cotton fabrics treated with chitosan. Text Res J 73:1103–6
- Zhang J, Wang C, Wang J, et al. (2012). In vivo drug release and antibacterial properties of vancomycin loaded hydroxyapatite/chitosan composite. Drug Deliv 19:264–9