Abstract
We report carbohydrate-anchored polyethylene sebacate (PES)-Gantrez® AN 119 Doxorubicin hydrochloride (Dox) nanoparticles (NPs) for enhanced anticancer efficacy. The carbohydrates Arabinogalactan (AGn), an adjuvant in anticancer chemotherapy and pullulan (Pul) reported to promote collagen synthesis, were selected as ligands. PES Dox NPs of an average size around 200 nm, greater than 20% w/w Dox loading and negative zeta potential were anchored with Pul, AGn, and Pul–AGn combination by simple incubation. Increase in particle size and zeta potential confirmed carbohydrate anchoring. FTIR confirmed ionic complexation of Dox and Gantrez® AN 119. DSC and XRD demonstrated amorphization of Dox. Higher Dox release in pH 5.5 as compared with pH 7.4 is beneficial for reduced systemic toxicity and enhanced drug release in tumors. Good in vitro serum stability and low hemolysis revealed suitability for intravenous administration. All NPs revealed circulation longevity in normal rats. Pul NPs revealed superior anticancer efficacy in vitro and an 11-fold enhancement in uptake in MCF-7 breast cancer cells. The greater efficacy in vivo is attributed to possible pullulan-mediated integrin receptor uptake and interaction with tumor collagen. Histopathology confirmed safety and suggested promise of Pul NPs in improved anticancer efficacy.
Introduction
The critical role played by tumor stroma in cancer progression is well documented (Bouzin & Feron, Citation2007; Engels et al., Citation2012; Chen et al., Citation2013; Narunsky et al., Citation2014). The extracellular matrix (ECM) of the tumor stroma is commonly deregulated and becomes disorganized in cancer with an upregulation of fibrillar collagens, proteoglycans, and various glycoproteins in the interstitial matrix (Matsumura, Citation2012). Monoclonal antibodies (mAbs) against collagen and fibrin extensively present in the stroma of solid tumors have been successfully developed (Matsumura, Citation2012). Matrix metalloproteinase (MMP) inhibitors are a class of anticancer agents that prevent collagen degradation and can thereby prevent metastasis (Overall & López-Otín, Citation2002). Targeting the abnormal ECM could, therefore, provide an effective avenue to combat cancer.
Carbohydrates are biomolecules that can interact with the extracellular matrix, interfere with the normal cell-recognition phenomenon, and inhibit tumor progression by interfering with angiogenesis, cell–cell and cell–matrix interactions, tumor invasion, and metastasis (Nangia-Makker et al., Citation2002). Carbohydrates are natural biomaterials with desirable features such as high stability, safety, hydrophilicity, and biodegradability and are metabolically removed from circulation (Scomparina et al., Citation2011). Being hydrophilic, they can provide stealth property to favor enhanced tumor accumulation (Liu et al., Citation2008). Surface modification by polysaccharides prolonged the in vivo circulation half life of nanoparticles in a manner similar to that of PEG (Gu et al., Citation2007). Moreover carbohydrates could also contribute in improving cancer therapeutics.
Arabinogalactan (AGn), a polysaccharide derived from the orchid, Anoectochilus formosanus, has potent innate immuno-modulatory and anti-cancer activity as demonstrated in CT26 colon cancer-bearing BALB/c mice (Yang et al., Citation2014). Larch arabinogalactan has been suggested as an adjuvant in cancer chemotherapy since it can stimulate natural killer (NK) cell cytotoxicity, augment immunity, and inhibit metastasis of tumor cells to the liver (Kelly, Citation1999). Pullulan and its derivatives are also explored for tumor delivery of anticancer drugs. Folic acid-anchored pullulan acetate nanoparticles of epirubicin exhibited high cytotoxicity toward human nasopharyngeal epidermal cancer cells (Zhang et al., Citation2010). pH-sensitive O-urocanyl pullulan nanoparticle of adriamycin efficiently delivered the drug to the nucleus of MCF-7/ADR cells and reversed the effect of drug resistance (Guo et al., Citation2014). Pullulan–PEI–ascorbic acid conjugate proved to be an efficient anticancer gene delivery vector with an intriguing property of promoting collagen synthesis (Ambattu & Rekha, Citation2015). Coating of liposomes with pullulan resulted in decreased uptake by the mononuclear phagocyte system (Woddle & Lasic, Citation1992; Allen, Citation1994).
In our earlier study, gamma scintigraphy revealed long circulating nature of pullulan functionalized Doxorubicin (Dox)-loaded polyethylene sebacate (PES)-Gantrez® AN 119 nanoparticles (PES Dox NPs) (Guhagarkar et al., Citation2010). Dox, an anthracycline antibiotic, is widely used to treat varied cancers including lymphoma, sarcomas, carcinomas, and melanoma (Elbialy et al., Citation2014). Poor clinical outcomes of Dox which arise from lack of selectivity and targeting ability to tumor sites, resistance to chemotherapy, and metastasis are circumvented by long circulating Dox NPs (Danhier et al., Citation2010; Du et al., Citation2014), which also help in decreasing cardiotoxicity and nephrotoxicity (Chang et al., Citation2014; Li et al., Citation2014).
Carbohydrates by enabling long circulation coupled with their possible role not only in targeting the components of tumor stroma but also in cancer chemotherapy could provide significant advantage in cancer (Buckway et al., Citation2013).
In the present study, we explore Dox nanoparticles anchored with the carbohydrates, Pul and AGn and Pul–AGn in combination. The specific objective of the study was to evaluate the role of carbohydrate anchoring on anticancer efficacy in vitro in the MCF-7 cell line and in vivo in the fibrosarcoma mouse model.
Experimental
Materials
Doxorubicin hydrochloride was obtained from Khandelwal Laboratories (Mumbai, India) as a gift sample. PES was synthesized in our laboratory (molecular weight: 9000–11 000). Gantrez® AN 119 (poly(methyl vinyl ether-co-maleic anhydride)) with an average molecular weight of 200 kDa) was obtained as a gift sample from Ashland India. Tween 80, glacial acetic acid AR, tetrahydrofuran AR (THF), acetone AR were purchased from s.d.fine Chemicals (Mumbai, India). Magnesium acetate tetrahydrate pure and sodium acetate trihydrate crystal pure was purchased from MERCK (Mumbai, India). Acetonitrile HPLC grade was supplied as gift sample by Azeocryst Organics Pvt. Ltd., Mumbai, India. Trehalose and pullulan (repeated glucose units; α-1,4;1,6-glucan, molecular mass of 200 kDa, Hayashibara, Japan) were supplied as gift samples by Gangwal Chemicals Pvt Ltd (Mumbai, India). Arabinogalactan (galactopyranose: arabinofuranose 6:1; 3,6-beta-D-galactan with β-galactose & β-arabinose side chains; average molecular weight ∼19 kDa) was purchased from Sigma, Mumbai, India. Tetrazolium MTT (3-(4,5-dimethylthiazolyl-2-yl)-2,5-diphenyltetrazolium bromide) were purchased from Sigma-Aldrich (St. Louis, MO). Filtered (0.45 μ membrane filter) doubled-distilled water was used for preparation of nanoparticles. All the other chemicals and reagents were either of spectroscopic or analytical grade.
Preparation of PES-Gantrez® and 119 Dox nanoparticles
PES-Gantrez Dox nanoparticles were prepared by modified nanoprecipitation technique using the polymers, PES and Gantrez® AN 119 as reported earlier (Guhagarkar et al., Citation2009,Citation2010). Briefly, the polymers were dissolved in organic phase (10 ml) of tetrahydrofuran (THF) and acetone (1:1). Aqueous phase (25 ml) consisted of Dox (10 mg) and tween 80 (10% v/v). Dropwise addition of organic phase to the aqueous phase under magnetic stirring followed by the addition of aqueous magnesium acetate tetrahydrate (1 ml of 0.5% w/v) resulted in the nanoparticle dispersion that was stirred for a period of 4 h to enable complete evaporation of the organic phase and then the dispersion was probe sonicated (DP120, Dakshin, Mumbai, India) for a period of 10 min with 15 s on/off cycle. Isolation of nanoparticles was done by centrifugation (14 000 rpm for 40 min), and the pellet was dispersed in aqueous phase. The ligands, pullulan (Pul), arabinogalactan (AGn), and Pul–AGn combination (nanoparticles:ligand ratio 1:1) were added to the nanoparticle dispersion, cyclomixed, allowed to stand for 10 min and probe sonicated (10 min with 15 s on/off cycle).
Physicochemical characterization of nanoparticles
To determine the entrapment efficiency, nanoparticles were separated from the dispersion by centrifugation at 14 000 rpm for 40 min. The supernatant obtained after centrifugation was suitably diluted and analyzed for free Dox by UV spectrophotometry (Shimadzu, Tokyo, Japan) at 478 nm. The % EE was calculated as follows:
(1)
Dox loading was calculated based on the weight ratio of the loaded amount of Dox to the amount of nanoparticles.
Particle size was determined by photon correlation spectroscopy using N4 plus submicron particle size analyzer (Beckman Coulter, Brea, CA) at 25 °C with all the measurements taken by scattering the light at 90°. Zeta potential of nanoparticles was determined using Zeta sizer Nano ZS (Malvern Instruments Ltd, Malvern, UK). For SEM, a drop of nanoparticle dispersion was deposited onto a carbon tape and dried under vacuum. The samples were sputtered with platinum using an auto fine coater and further observed for morphology (JSM-6380-LA, JEOL, Tokyo, Japan).
Fourier transform infrared (FTIR) spectroscopy
FTIR spectrum of PES, Gantrez® AN 119, Dox, Pul, AGn, and the nanoparticles was recorded with Perkin Elmer Infrared Spectrophotometer (Perkin Elmer Inc., Waltham, MA) following KBr pellet technique. The freeze dried powders were mulled with KBr, compressed to form a thin transparent pellet and subjected to FTIR analysis.
Differential scanning calorimetry (DSC) and X-ray diffraction (XRD)
Samples accurately weighed (5 mg) in aluminum pans were sealed and subjected to differential scanning calorimetry under nitrogen flow using a Perkin Elmer Pyris 6 DSC thermal analysis instrument (Perkin Elmer Inc., Waltham, MA). Thermograms were recorded by heating samples from 40 °C to 300 °C at a heating rate of 10 °C min − 1. Empty aluminum pan served as the reference.
XRD spectra of the freeze dried nanoparticles were recorded at room temperature using Philips Pro Expert diffractometer (Philips Electronics North America Corporation, Andover, MA), with nickel filtered Cu Kα radiation at a voltage of 3 kV, 5 mA current, 4°/min scanning speed, and 5°–40° (2θ) range.
In vitro drug release studies
The release of Dox was determined by the dialysis bag method using USP dissolution apparatus I at a stirring speed of 50 rpm. The dissolution medium was 500 mL phosphate buffer pH 7.4 and acetate buffer, pH 5.5 maintained at 37° ± 0.5 °C.
Dialysis membrane (HIMEDIA®, molecular weight cut off 12 000–14 000 Da) were cut, tied at one end, and hydrated in water prior to use. Dox solution and nanoparticulate dispersion (equivalent to 4 mg Dox) were introduced into the dialysis bag, another end of the bag tied, and placed into basket and dissolution commenced. Dox released was monitored by UV spectrophotometry at 478 nm.
Stability evaluation
The freeze-dried NPs packed in glass vials, stoppered with rubber closure, and crimped with aluminum overseal were stored at different conditions of temperature and humidity as per ICH guidelines namely 4 °C, 40°± 2 °C/75 ± 5% RH, and 30°± 2 °C/60 ± 5% RH. The nanoparticles were examined for change in particle size, PI, drug content, and physical changes.
In vitro hemolytic assay and serum stability
A previously reported method was followed for hemolytic assay and serum stability (Guhagarkar et al., Citation2010). The nanoparticles equivalent to Dox concentrations 0.05, 0.1, 0.2, 0.3, 0.4, and 0.5 mg/mL were prepared in phosphate buffer saline. Each dilution of 1.45 mL was incubated with 50 μL of rat whole blood at 37 °C for 60 min, centrifuged at 5000 rpm for 10 min, and the released hemoglobin was quantified spectrophotometrically at a wavelength of 540 nm. PBS served as a negative control and distilled water as a positive control. The % hemolysis was calculated as shown below:
(2)
In order to evaluate serum stability, particle size of the nanoparticles upon incubation with rat serum (50% v/v) in a 1:1 ratio at 37 ± 0.5 °C was monitored over 6 h.
Pharmacokinetic study
Female Sprague–Dawley rats (200–250 g) fasted for 12 h were divided into five groups (n = 6): Group I – Dox solution, Group II – NPs, Group III – Pul NPs, Group IV – AGn NPs, and Group V – Pul–AGn NPs. The formulations with Dox equivalent to 6 mg/kg body weight were administered to the rats by tail vein. At 5 min, 30 min, 1, 2, 6, 10, and 24 h post dosing, blood samples withdrawn from retro-orbital plexus were collected in tubes containing 4.1% EDTA. Plasma was separated by centrifuging blood at 5000 rpm for 10 min and stored at −20°C until analysis. Dox was quantified by HPLC. To 200 μl of plasma, 200 μl of acetonitrile was added, vortex mixed, and sonicated for 5 min each, followed by centrifugation at 20 000 rpm for 20 min. The supernatant was injected into the HPLC system (Jasco LC 1500, Jasco Tools, Inc., Rochester, NY) coupled with UV detector (Jasco UV/VIS 1570/1575, Jasco Tools, Inc., Rochester, NY) and Rheodyne injector model fitted with 100 μl sample loop. Chromatography was performed on a Waters Spherisorb® S5 ODS2 (250 × 4.6 mm i.d., 5 μm particle size) column (Waters Inc., Milford, MA) at room temperature under isocratic conditions at a flow rate of 1 mL/min with UV detection at a λmax of 254 nm. The mobile phase comprised of acetonitrile:methanol:acetate buffer pH-5.0 (20:30:50v/v). The pharmacokinetic parameters were calculated using BASICA software (Basic Systems, Marion, IN).
In vitro cell cytotoxicity
The human breast adenocarcinoma cell line MCF-7 was procured from National Center for Cell Sciences (NCCS), Pune, India. MCF7 cells were cultured in DMEM medium supplied with 10% fetal bovine serum (FBS), 1% penicillin–streptomycin solution at 37 °C in humidified environment of 5.0% CO2. Every 24 h medium was changed till cells exhibited confluency. Subsequently, the cells were washed with PBS and harvested with 0.125% Trypsin–EDTA solution.
Cells harvested in a logarithmic growth phase were seeded on a 96-well plate at a cell density of 1 × 105 cells per ml, incubated with various concentrations of Dox solution and NPs from 0.78125 to 100 μg/mL for 2/12/24/48 h. The % cell viability was determined by the MTT assay (Garg et al., Citation2012). At selected time points, the medium was discarded, and wells were washed twice with PBS. Cells were incubated with MTT reagent (5 mg/mL in PBS) for 3–4 h. The formazan crystals formed due to reduction of MTT by viable cells was dissolved in DMSO and absorbance in each well was analyzed by the microplate reader at 570 nm. The cell uptake of Dox was determined after processing the cells in the same way as for cytotoxicity. The cells were incubated with Dox solution or nanoparticles equivalent to 50 μg/mL for 2 h at 37 °C. At 2 h, supernatant was collected and cells were lysed using 0.5% w/v sodium lauryl sulfate solution. After cell detachment enabled by trypsin-EDTA solution, Dox content in the cell lysate was evaluated by developed HPLC method.
Efficacy study in fibrosarcoma tumor-bearing mice
Fibrosarcoma mouse model was used to evaluate the anti-cancer efficacy of Dox solution and nanoparticles. Mice with fibrosarcoma obtained from Bhabha Atomic Research Center (BARC), Mumbai, India, were euthanized, tumor mass excised, and further homogenized in sterile PBS. Albino mice weighing 20–30 gm was induced with tumor by subcutaneous dorsal injection of fibrosarcoma cell suspension (0.2 mL). When tumor diameter reached 8–10 mm, the animals were divided into seven groups (n = 6). Group-I was the control group without any treatment and Group-II was the control group with placebo PES nanoparticles; Group-III, Group-IV, Group-V, Group-VI, and Group-VII received Dox solution, NPs, Pul NPs, AGn NPs, and Pul–AGn NPs, respectively, in 5% Dextrose (0.2 mL). The mice were treated on days 1, 4, and 7 by intravenous (i.v.) injections through tail vein of either Dox solution (2.5 mg/kg) or NPs (equivalent to Dox 2.5 mg/kg) (Akao et al., Citation2010; Khandekar et al., Citation2014; Benival & Devarajan, Citation2015). The animals were maintained under standard laboratory conditions (14 h: 10 h dark/light cycle and a temperature of 22 ± 2 °C). Antitumor activity was evaluated by monitoring tumor growth rate and the tumor size was measured using slide calipers. The tumor surface area was calculated by approximation of the tumor solid to an ellipsoid, i.e. using the following equation:
(3)
where a, b, and c are length, width, and height (cm), respectively, s is the size (cm3) and k a constant (Khandekar et al., Citation2014).
At the end of 2 months, mice were euthanized, organs like heart, kidneys, liver, spleen, lungs, brain removed, and subjected to histopathology studies.
Statistical analysis
All values were expressed as mean value ± standard deviation (S.D.) of at least three independent experiments. Statistical analysis was performed using the one-way ANOVA with Dunnet’s test, Bonferroni t test, and Student’s t-tests. p < 0.05 was the criterion for statistical significance.
All animal experimental procedures were reviewed and approved by the Institutional Animal Ethics Committee (IAEC) of Institute of Chemical Technology, Department of Pharmaceutical Sciences and Technology, Mumbai, India (ICT/IAEC/2011/P72 and ICT/IAEC/2012/P12).
Results and discussion
PES is a biocompatible, safe, and biodegradable polymer (Guhagarkar et al., Citation2009; More et al., Citation2009). Application of PES in the design of drug-loaded nanoparticles is also demonstrated with silymarin (Guhagarkar et al., Citation2015), rifampicin (Date et al., Citation2013; Patel et al., Citation2013), nevirapine (Dalvi et al., Citation2015) and Dox (Guhagarkar et al., Citation2010). The long circulation exhibited by pullulan-anchored PES-Gantrez® AN 119 Dox NPs prompted us to explore nanoparticles anchored with pullulan and another carbohydrate AGn, and Pul–AGn combination for anticancer efficacy in vitro and in vivo.
Preparation and physicochemical characterization of PES-Gantrez® AN 119 Dox nanoparticles
PES-Gantrez® AN 119 Dox NPs were successfully prepared by the modified nanoprecipitation method in the size range of 205–230 nm. High entrapment of >70% and drug loading of around 20%w/w as reported earlier was achieved with all the nanoparticles (Guhagarkar et al., Citation2010). The nanoparticles revealed spherical morphology ().
Anchoring of ligands onto the surface by simple incubation is a promising and simple approach compared to complex chemical reactions. Stable ligand incorporation following such post insertion techniques is reported. Further the ligands exert no influence on the drug loading efficacy and release characteristics (Iden & Allen, Citation2001). Increase in the average size by about 28 nm (Jokerst et al., Citation2011) and in zeta potential from −27 mv for NPs to −22, −17, and −19 mv for Pul NPs, AGn NPs, and Pul–AGn NPs, respectively, due to shielding of the negative charge of the nanoparticles, confirmed anchoring of the carbohydrates (Lemarchand et al., Citation2004; Joshi & Devarajan, Citation2014). Importantly, negative zeta potential could favor reduced plasma protein adhesion and low non-specific cellular uptake (Zhang et al., Citation2012).
FTIR
A band appearing at 3327 cm− 1 in the IR spectrum of Dox in is due to O–H/N–H stretching vibrations, while the band observed at 2933 cm− 1 is due to the C–H stretching vibrations (Li et al., Citation2009; Wu et al., Citation2013). The carbonyl absorption bands of Gantrez® AN 119 appeared at 1716 cm− 1 and 1635 cm− 1. The absorption bands at 1728 cm − 1 seen in the nanoparticles can be assigned to the free carbonyl groups of Gantrez® AN 119. The disappearance of 1635 cm−1 band along with the absence of NH3+ stretching vibrations (3450 cm− 1) of Dox in the nanoparticles and the appearance of peak at 1560 cm− 1 of –CONH– confirmed ionic complexation between Dox and Gantrez® AN 119 (Cerchiara et al., Citation2005; Guhagarkar et al., Citation2010; Benival & Devarajan, Citation2012; Date et al., Citation2013). The intensive, broad hydroxyl band at 3000–3600 cm−1 along with C–O–C stretching at 1000–1260 cm−1 confirmed adsorption of the carbohydrates on the nanoparticles (Cheng et al., Citation2010).
DSC and XRD
Disappearance of the melting endotherm of Dox which appeared at around 222°C from Dox-loaded nanoparticles () implied complete amorphization or disordered crystalline phase of Dox in the nanoparticles. XR diffractograms which revealed a halo patterned spectrum with the Dox peaks missing correlated with the DSC analysis data for the nanoparticles () and confirmed the amorphous nature of Dox in NPs (Kalaria et al., Citation2009).
In vitro drug release
The nanoparticles demonstrated a biphasic drug release pattern with an initial burst release, followed by sustained release attributed to the polymeric matrix imparting a barrier to drug release. Under acidic conditions, protonation of the carboxyl groups of Gantrez® AN 119 (pKa 2.5) and the amine group of Dox (NH3+) (pKa 8.46) resulted in weaker interaction between Gantrez® AN 119 and Dox enabling faster release of Dox at pH 5.5 (t50%=15 h) compared with pH 7.4 () (Khandekar et al., Citation2014). Similar results are reported for Dox/γ-Polyglutamic acid ionic complex (Manocha & Margaritis, Citation2010). This could imply decreased release in blood plasma (pH 7.4) and normal tissues and thereby reduced systemic exposure of Dox. The higher release in pH 5.5, the tumor pH due to the Warburg effect could provide high drug localization in tumors (Wang et al., Citation2014). The kinetics of Dox release from nanoparticles was fitted to various kinetic models such as zero-order, first-order, Higuchi equation, and Korsmeyer–Peppas equation (). Dox release was considered to follow the Korsmeyer–Peppas model as the correlation coefficient (r2 values) for the nanoparticles in the graph of log % cumulative release versus log % time were close to unity with the release component value n > 0.5 suggesting anomalous (non-Fickian) diffusion mechanism (Sahoo et al., Citation2012).
Figure 4. In vitro release of Dox from various Dox formulations (mean ± S.D.; n = 3). (A) Phosphate buffer, pH 7.4. (B) Acetate buffer, pH 5.5.
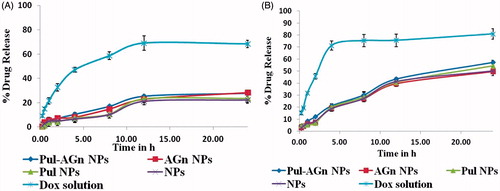
Table 1. Kinetic models for in vitro drug release.
Stability evaluation
The nanoparticles at 40°± 2 °C/75 ± 5% RH showed an increase in particle size (317 ± 25 nm) and PI (0.856 ± 0.04) at the end of 6 months. However, nanoparticles stored at 30°± 2 °C/60 ± 5% and 4 °C showed good physical and chemical stability as no significant change in particle size, PI, drug content, and good redispersibility was observed up to 12 months.
In vitro hemolytic assay and serum stability
Examination of hemolytic potential of intravenously administered nanoparticles is crucial as interaction of nanoparticles with erythrocytes can lead to adverse reactions (Naahidi et al., Citation2013). As in vitro percent hemolysis from 5 to 25% is accepted as “no concern’ (Dobrovolskaia et al., Citation2008), the nanoparticles which exhibited hemolysis of <15% () are considered safe for intravenous administration. Further no significant change in size (p > 0.05) in serum over 6 h suggests no alterations in disposition in vivo (Yallapu et al., Citation2015). It also indicates minimal interaction with serum proteins and is attributed to the negative zeta potential of the nanoparticles ().
Pharmacokinetic study
The plasma concentration time profiles after intravenous administration of Dox solution and Dox-loaded nanoparticles are shown in . A biphasic pattern with an initial rapid drug distribution phase followed by a slower elimination phase was observed. However, while Dox solution was rapidly cleared from circulation, Dox concentrations from nanoparticles were significantly higher (p < 0.001) at all time points. Dox nanoparticles showed significantly longer t1/2, higher AUC0–24 h (∼10 fold), a lower rate of clearance and a smaller volume of distribution in comparison with Dox solution (). Moreover, even at the end of 24 h, plasma Dox concentration was sustained with the Dox concentration significantly higher (p < 0.001) than that of Dox solution. High t1/2 seen even in the absence of the ligands is attributed to the hydrophilic and anionic nature of Gantrez® AN 119 that averts interaction with plasma proteins and RES components. Such circulation longevity could play a key role in enabling site-specific delivery to tumors by enhanced permeability and retention effect.
Figure 6. Plasma concentration versus time profiles of Dox following intravenous administration of Dox formulations (mean ± S.D.; n = 6).
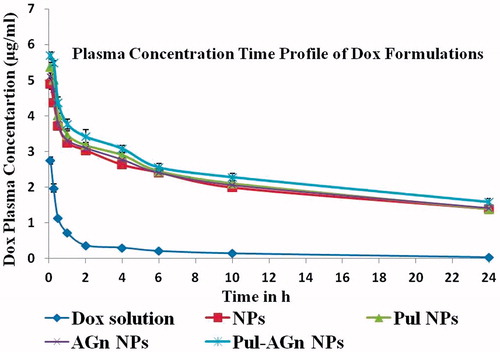
Table 2. Pharmacokinetic parameters of Dox solution, NPs, and carbohydrate-anchored NPs.
In vitro cell cytotoxicity
PES Gantrez® AN 119 NPs revealed 99.54% cell viability even after 48 h confirming their biocompatibility. Both Dox solution and the nanoparticles revealed significantly high and comparable cell viability of > 70% at 2 h (Khandekar et al., Citation2014). Further, although a significant decrease in cytotoxicity was seen at 12 h, it was comparable for Dox solution and the nanoparticles. Significant enhancement in cytotoxicity was evident with NPs and Pul NPs at 24 h and 48 h (). Maximum uptake of Dox in MCF 7 cell line seen with Pul NPs (45.66%) is responsible for the high cytotoxicity. Although comparable cytotoxicity was seen with AGn NPs, Pul–AGn NPs, and Dox solution, uptake obtained with NPs (29.65%), AGn NPs (28.69%), and Pul–AGn NPs (31.2%) was comparable while uptake of Dox solution was significantly lower (4.34%). Hence the comparable cytotoxicity is probably due to sustained release of Dox from nanoparticles as seen in . Similar observations for HPMA copolymer Dox conjugate nanoparticles are reported (Yang et al., Citation2013). Such low Dox uptake from solution is attributed to possible Pgp efflux which limits intracellular Dox concentrations (Dong & Mumper, Citation2010). The very high Dox uptake of Pul NPs (11-fold enhancement compared with Dox solution) was possibly mediated by endocytosis through integrin receptors known to be expressed on MCF 7 cells (Silva et al., Citation2014; Singh et al., Citation2015).
Efficacy study in fibrosarcoma tumor-bearing mice
The untreated control showed unabated increase in tumor volume necessitating termination of the animals within 19–21 days after initiation of treatment. All the treatment groups, however, showed regression in tumor volume with comparative reduction in tumor volume till 23 days (). Nevertheless, while sustained regression was observed with the nanoparticle groups, the Dox solution-treated group revealed significant increase in tumor volume suggesting rapid elimination resulting in re-emergence of tumor growth. The long circulating nature of the nanoparticles favored enhanced tumor accumulation with retention explained by the enhanced permeability and retention (EPR) effect (Matsumura & Maeda, Citation1986). A good correlation between in vitro cell uptake and in vivo efficacy was evident with the Pul-anchored NPs revealing significantly enhanced reduction in tumor volume compared with the other groups (p < 0.05). Comparable reduction in tumor volume was seen with NPs, AGn NPs, and Pul–AGn NPs. However, beyond 50 days, AGn NPs and Pul–AGn NPs showed significantly enhanced efficacy compared with NPs (p < 0.05) suggesting possible interaction of the carbohydrates with the tumor matrix. Further the superior efficacy of Pul NPs implies that in addition to the EPR effect, the affinity to integrin receptors expressed on cancer cells (Singh et al., Citation2015) could have played a role. Moreover, fibrosarcoma tumors are known to secrete collagen in large quantities and manipulation of collagen by modification or reorganization is proposed as a promising strategy for anticancer efficacy (Karin Eisinger-Mathason et al., Citation2013), with systems which can promote collagen production or prevent degradation playing a critical role in preventing cancer metastasis. The ability of Pul to promote collagen synthesis (Ambattu & Rekha, Citation2015) could also, therefore, be a contributing factor. Among the carbohydrates, therefore, Pul reflects great promise for enhanced anticancer efficacy in the fibrosarcoma model.
The Dox solution-treated groups revealed minimal degree diffuse granular degeneration in liver, kidney with focal cortical chronic glomerulonephritis, and mild degree cystic dilations of tubules. The distribution of Dox solution to non-target cells leads to cytotoxic effects on heart, kidney, and liver. Although minimally multifocal mild degree cystic dilations of tubules in kidney were observed with the NPs, no abnormality of heart, liver, lungs, spleen, and brain tissue observed with the all NPs and comparable with control group (untreated) suggests their possible safety ().
Conclusion
The carbohydrate-anchored PES Dox NPs show great promise for improved anticancer efficacy with the pullulan-anchored NPs being particularly effective in tumors overexpressing collagen.
Acknowledgements
The authors wish to acknowledge Dr. H. D. Sarma, Bhabha Atomic Research Center (BARC), Mumbai, India, for providing fibrosarcoma tumor-induced mouse and Tata Institute of Fundamental Research, Mumbai, India for XRD.
Declaration of interest
The authors report no declaration of interest. Sandhya Pranatharthiharan and Mitesh D. Patel are thankful to University Grants Commission (UGC-SAP) and Department of Biotechnology (DBT, India) for grant and fellowship.
References
- Akao T, Kimura T, Hirofuji YS, et al. (2010). A poly(γ-glutamic acid)–amphiphile complex as a novel nanovehicle for drug delivery system. J Drug Target 18:550–6
- Allen TM. (1994). The use of glycolipids and hydrophilic polymers in avoiding rapid uptake of liposomes by the mononuclear phagocyte system. Adv Drug Deliv Rev 13:285–309
- Ambattu LA, Rekha MR. (2015). Collagen synthesis promoting pullulan-PEI-ascorbic acid conjugate as an efficient anti-cancer gene delivery vector. Carbohydr Polym 126:52–61
- Benival DM, Devarajan PV. (2012). Lipomer of doxorubicin hydrochloride for enhanced oral bioavailability. Int J Pharm 423:554–61
- Benival DM, Devarajan PV. (2015). In situ lipidization as a new approach for the design of a Self Microemulsifying Drug Delivery System (SMEDDS) of doxorubicin hydrochloride for oral administration. J Biomed Nanotechnol 10:913–22
- Bouzin C, Feron O. (2007). Targeting tumor stroma and exploiting mature tumor vasculature to improve anti-cancer drug delivery. Drug Resist Updates 10:109–20
- Buckway B, Wang Y, Ray A, Ghandehari H. (2013). Overcoming the stromal barrier for targeted delivery of HPMA copolymers to pancreatic tumors. Int J Pharm 456:202–11
- Cerchiara T, Luppi B, Chidichimo G, et al. (2005). Chitosan and poly(methyl vinyl ether-co-maleic anhydride) microparticles as nasal sustained delivery systems. Eur J Pharm Biopharm 61:195–200
- Chang JE, Yoon IS, Sun PL, et al. (2014). Anticancer efficacy of photodynamic therapy with hematoporphyrin-modified, doxorubicin-loaded nanoparticles in liver cancer. J Photochem Photobiol B: Biol 140:49–56
- Chen P, Cescon M, Bonaldo P. (2013). Collagen VI in cancer and its biological mechanisms. Trends Mol Med 19:410–17
- Cheng K, Demirci A, Catchmark JM. (2010). Effects of plastic composite support and pH profiles on pullulan production in a biofilm reactor. Appl Microbiol Biotechnol 86:853–61
- Dalvi BR, Shelke RU, Siddiqui EA, et al. (2015). Preparation, characterization and surface modification of nevirapine nanoparticles. Am J PharmTech Res 5:187–204
- Danhier F, Feron O, Préat V. (2010). To exploit the tumor microenvironment: passive and active tumor targeting of nanocarriers for anti-cancer drug delivery. J Control Release 148:135–46
- Date PV, Patel MD, Majee SB, et al. (2013). Ionic complexation as a non-covalent approach for the design of folate anchored rifampicin Gantrez nanoparticles. J Biomed Nanotechnol 9:765–75
- Dobrovolskaia MA, Clogston JD, Neun BW, et al. (2008). Method for analysis of nanoparticle hemolytic properties in vitro. Nano Lett 8:2180–7
- Dong X, Mumper RJ. (2010). Nanomedicinal strategies to treat multidrug-resistant tumors: current progress. Nanomedicine (Lond) 5:597–615
- Du JZ, Mao CQ, Yuan YY, et al. (2014). Tumor extracellular acidity-activated nanoparticles as drug delivery systems for enhanced cancer therapy. Biotechnol Adv 32:789–803
- Elbialy NS, Fathy MM, Khalil WM. (2014). Preparation and characterization of magnetic gold nanoparticles to be used as doxorubicin nanocarriers. Phys Med 30:843–8
- Engels B, Rowley DA, Schreiber H. (2012). Targeting stroma to treat cancers. Semin Cancer Biol 22:41–9
- Garg NK, Dwivedi P, Campbell C, Tyagi RK. (2012). Site specific/targeted delivery of gemcitabine through anisamide anchored chitosan/poly ethylene glycol nanoparticles: an improved understanding of lung cancer therapeutic intervention. Eur J Pharm Sci 47:1006–14
- Gu M, Yuan X, Kang C, et al. (2007). Surface biofunctionalization of PLA nanoparticles through amphiphilic polysaccharide coating and ligand coupling: evaluation of biofunctionalization and drug releasing behavior. Carbohydr Polym 67:417–26
- Guhagarkar SA, Malshe VC, Devarajan PV. (2009). Nanoparticles of polyethylene sebacate: a new biodegradable polymer. AAPS PharmSciTech 10:935–42
- Guhagarkar SA, Malshe VC, Gaikwad RV, et al. (2010). Polyethylene sebacate-Doxorubicin nanoparticles for hepatic targeting. Int J Pharm 401:113–22
- Guhagarkar SA, Shah D, Patel MD, et al. (2015). Polyethylene sebacate-silymarin nanoparticles with enhanced hepatoprotective activity. J Nanosci Nanotechnol 15:4090–3
- Guo H, Liua Y, Wang Y, et al. (2014). pH-sensitive pullulan-based nanoparticle carrier for adriamycin to overcome drug-resistance of cancer cells. Carbohydr Polym 111:908–17
- Iden DL, Allen TM. (2001). In vitro and in vivo comparison of immunoliposomes made by conventional coupling techniques with those made by a new post-insertion approach. Biochim Biophys Acta 1513:207–16
- Jokerst JV, Lobovkina T, Zare RN, Gambhir SS. (2011). Nanoparticle PEGylation for imaging and therapy. Nanomedicine (Lond) 6:715–28
- Joshi VM, Devarajan PV. (2014). Receptor-mediated hepatocyte-targeted delivery of primaquine phosphate nanocarboplex using a carbohydrate ligand. Drug Deliv Transl Res 4:353–64
- Kalaria DR, Sharma G, Beniwal V, Ravi Kumar MN. (2009). Design of biodegradable nanoparticles for oral delivery of doxorubicin: in vivo pharmacokinetics and toxicity studies in rats. Pharm Res 26:492–501
- Karin Eisinger-Mathason TS, Zhang M, Qiu Q, et al. (2013). Hypoxia-dependent modification of collagen networks promotes sarcoma metastasis. Cancer Discov 3:1190–205
- Kelly GS. (1999). Larch arabinogalactan: clinical relevance of a novel immune-enhancing polysaccharide. Altern Med Rev 4:96–103
- Khandekar SV, Kulkarni MG, Devarajan PV. (2014). Polyaspartic acid functionalized gold nanoparticles for tumor targeted doxorubicin delivery. J Biomed Nanotechnol 10:143–53
- Lemarchand C, Gref R, Couvreur P. (2004). Polysaccharide decorated nanoparticles. Eur J Pharm Biopharm 58:327–41
- Li Q, Lv S, Tang Z, et al. (2014). A co-delivery system based on paclitaxel grafted mPEG-b-PLG loaded with doxorubicin: preparation, in vitro and in vivo evaluation. Int J Pharm 471:412–20
- Li S, Ma Y, Yue X, et al. (2009). One-pot construction of doxorubicin conjugated magnetic silica nanoparticles. New J Chem 33:2414–8
- Liu Z, Jiao Y, Wang Y, et al. (2008). Polysaccharides-based nanoparticles as drug delivery systems. Adv Drug Deliv Rev 60:1650–62
- Manocha B, Margaritis A. (2010). Controlled release of doxorubicin from doxorubicin/γ-polyglutamic acid ionic complex. J Nanomater 2010:1–9. Article ID 780171
- Matsumura Y, Maeda H. (1986). A new concept for macromolecular therapeutics in cancer chemotherapy: mechanism of tumoritropic accumulation of proteins and the antitumor agent smancs. Cancer Res 46:6387–92
- Matsumura Y. (2012). Cancer stromal targeting (CAST) therapy. Adv Drug Deliv Rev 64:710–19
- More AB, Chilgunde SN, Kamble JC, et al. (2009). Polyethylene sebacate: genotoxicity, mutagenicity evaluation and application in periodontal drug delivery system. J Pharm Sci 98:4781–95
- Naahidi S, Jafari M, Edalat F, et al. (2013). Biocompatibility of engineered nanoparticles for drug delivery. J Control Release 166:182–94
- Nangia-Makker P, Conklin J, Hogan V, Raz A. (2002). Carbohydrate-binding proteins in cancer, and their ligands as therapeutic agents. Trends Mol Med 8:187–92
- Narunsky L, Oren R, Bochner F, Neeman M. (2014). Imaging aspects of the tumor stroma with therapeutic implications. Pharmacol Ther 141:192–208
- Overall CM, López-Otín C. (2002). Strategies for MMP inhibition in cancer: innovations for the post-trial era. Nat Rev Cancer 2:667–72
- Patel MD, Date PV, Gaikwad RV, et al. (2013). Comparative evaluation of polymeric nanoparticles of rifampicin comprising Gantrez and poly(ethylene sebacate) on pharmacokinetics, biodistribution and lung uptake following oral administration. J Biomed Nanotechnol 10:687–94
- Sahoo S, Chakraborti CK, Behera PK. (2012). Development and evaluation of gastroretentive controlled release polymeric suspensions containing ciprofloxacin and carbopol polymers. J Chem Pharm Res 4:2268–84
- Scomparina A, Salmasoa S, Bersani S, et al. (2011). Novel folated and non-folated pullulan bioconjugates for anticancer drug delivery. Eur J Pharm Sci 42:547–58
- Silva MCC, de Paula CAA, Ferreira JG, et al. (2014). Bauhinia forficata lectin (BfL) induces cell death and inhibits integrin-mediated adhesion on MCF7 human breast cancer cells. Biochim Biophys Acta 1840:2262–71
- Singh RS, Kaura N, Kennedy JF. (2015). Pullulan and pullulan derivatives as promising biomolecules for drug and gene targeting. Carbohydr Polym 123:190–207
- Wang J, Cui S, Bao Y, et al. (2014). Tocopheryl pullulan-based self assembling nanomicelles for anti-cancer drug delivery. Mater Sci Eng C Mater Biol Appl 43:614–21
- Woddle MC, Lasic DD. (1992). Sterically stabilized liposomes. Biochim Biophys Acta 1113:171–99
- Wu S, Zhao X, Li Y, et al. (2013). Adsorption properties of doxorubicin hydrochloride onto graphene oxide: equilibrium, kinetic and thermodynamic studies. Materials 6:2026–42
- Yallapu MM, Chauhan N, Othman SF, et al. (2015). Implications of protein corona on physico-chemical and biological properties of magnetic nanoparticles. Biomater 46:1–12
- Yang Y, Pan D, Luo K, Gu LLZ. (2013). Biodegradable and amphiphilic block copolymer doxorubicin conjugate as polymeric nanoscale drug delivery vehicle for breast cancer therapy. Biomater 34:8430–43
- Yang L, Hsieh C, Lu T, Lin W. (2014). Structurally characterized arabinogalactan from Anoectochilus formosanus as an immuno-modulator against CT26 colon cancer in BALB/c mice. Phytomedicine 21:647–55
- Zhang C, Wang W, Liu T, et al. (2012). Doxorubicin-loaded glycyrrhetinic acid-modified alginate nanoparticles for liver tumor chemotherapy. Biomater 33:2187–96
- Zhang HZ, Li XM, Gao FP, et al. (2010). Preparation of folate-modified pullulan acetate nanoparticles for tumor-targeted drug delivery. Drug Deliv 17:48–57