Abstract
The structure and composition of the round window membrane (RWM) make it a particularly effective pathway for drug delivery to the inner ear. Therefore, predicting the efficiency of RWM transport would provide useful information for enhancing local application. In the present study, a mathematical model was established to achieve this goal. A series of drugs with different physicochemical properties were introduced in the inner ear cavity of guinea pigs via RWM by intratympanic application. The perilymphatic drug concentration (C) data were used to calculate the permeability coefficient (Kp) of different drugs diffusing through the RWM. The experimental data were fitted using the Matlab software to set up the numerical model based on Fick’s diffusion law and the single-compartment model following extravascular administration, which facilitated the prediction of the permeation profiles of different drugs while trans-RWM. In summary, this mathematical model is a contribution toward developing potentially useful RWM administration simulating tools.
Introduction
Systemic drug delivery cannot adequately treat numerous inner ear disorders. The blood–labyrinth barrier (BLB), which is physiologically similar to the blood–brain barrier (BBB), limits the concentration and size of molecules that can be transported from the blood circulation to the inner ear cell (McCall et al., Citation2010; Liu et al., Citation2013). There is an increasing interest in the use of topical applications for the treatment of inner ear disorders. Local administration may enable drugs to bypass the BLB and gain direct access to the inner ear, thereby increasing the drug concentrations there, and reduce systemic absorption and toxicity (Salt & Plontke, Citation2005). Topical administration may be achieved by either the intratympanic or intracochlear routes. However, intracochlear administration not only requires complex surgical techniques, but also carries significant risks such as possible histological damages, infection, and toxicity (Staecker et al., Citation2010; El Kechai et al., Citation2015; Kim et al., Citation2015). In contrast, intratympanic administration, which was introduced in clinics more than half a century ago, has proven to be an effective route for inner ear drug delivery with minimum damages (Duan & Chen, Citation2009). It is performed by the injection or perfusion of drugs into the middle ear with the aim of achieving drug diffusion through the round window membrane (RWM; McCall et al., Citation2010). The RWM is the only soft tissue structure located at the end of the scale tympani and separates the middle ear and the inner ear. It consists of three basic layers: an outer epithelium layer, a middle core of connective tissue, and an inner epithelium (Hellstrom et al., Citation1989). Animal studies have shown that this triple-layered membrane absorbs or secretes substances in the inner ear, which makes it the main portal for drug delivery to the inner ear (Juhn et al., Citation1989; Goycoolea & Lundman, Citation1997). For further development and translation of this promising therapeutic strategy to controlled clinical trials, it is necessary to conduct preclinical studies to establish pharmacokinetic and toxicological dose–effect relationships. Therefore, determining and monitoring drug pharmacokinetics following topical administration in the inner ear is critical to the successful therapeutic outcome.
Experimental studies of drug pharmacokinetics are technically challenging due to limited size and difficulty in accessing the inner ear. Human inner ear fluids cannot be safely sampled without damaging the inner ear (Plontke & Salt, Citation2003,Citation2006). The alternative is to predict drug levels from results of animal experiments. However, sampling the inner ear fluid in animal models is also difficult and requires special techniques such as cochlear fenestration. Moreover, continuous sampling cannot be achieved, resulting in the use of large numbers of animals and their euthanization. Criticism of animal experiments from the viewpoint of animal welfare is also a concern, which has persisted and greatly increased over the last decade (Donnellan, Citation2007). According to press release, the European Union (EU), South Korea, and India have already banned animal experiments in the development and production of imported cosmetic formulations permanently (Pauwels & Rogiers, Citation2007; Anonymous, Citation2013; McDodgall, Citation2015). This should impress on researchers the need to reduce the number of animals used in the experiments and unnecessary euthanization of animals. Therefore, the development of a method to simulate inner ear pharmacokinetics and reduce the number of animals used in research is desirable. The computer simulation software – Cochlear Fluids Simulator (latest version 3.083) – has already been programed by Plontke and Salt to predict drug distribution in the cochlea (Plontke & Salt, Citation2003). In this simulation software, the permeability of the drug transported via RWM is regarded as an important and necessary parameter for calculating the drug pharmacokinetics of the inner ear (Plontke, Citation2015). However, to date no mathematical model has been developed to quantify drug entry in terms of round window permeability although studies have documented the passage of solutes through the RWM.
In this current study, we have established a preliminary mathematical model to predict the permeability coefficient (Kp) of the drug diffusing through the RWM, which was analyzed in our previous in vivo experiment. This numerical model aims to estimate the RWM permeability of different drugs based on their physicochemical properties.
Theoretical
Analyzing local trans-RWM drug transport profiles
The inner ear cavity can be considered as a closed system because of the BLB which ensures that drugs barely reach or leave the inner ear cavity. Furthermore, the RWM is the main passage for drug delivery from the middle ear cavity to the inner ear. Therefore, the process of locally administering drugs via the RWM can be regarded as a single-compartment model with extravascular administration when the drug loss in the middle ear is not considered.
In a single-compartment model, a drug is delivered from the experimental side (in this case the middle ear cavity) to the inner ear after topical administration, and is considered to distribute swiftly into a unique compartment (in this case the perilymph).
The RWM acts like a semipermeable membranous interfaces (Duan & Chen, Citation2009; Liu et al., Citation2013; El Kechai et al., Citation2015) and low-molecular weight substances such as various kinds of aminoglycoside antibiotics and corticosteroids are rapidly transported through it (Juhn et al., Citation1989). Passive diffusion was generally considered to be the main mechanism by which drug migrates from the middle ear cavity to the inner ear through the triple-layered RWM (Salt & Plontke, Citation2005,Citation2009). Fick’s Laws of Diffusion specify that passive diffusion occurs in favor of the concentration gradient, and therefore, moves from a higher to lower concentration (Couto et al., Citation2014; Yu et al., Citation2015). This principle is applied in describing drug absorption through the RWM in this mathematical model.
For instance, let us consider that Q is the amount of a drug absorbed, and a specific portion of that drug will flow in a short time through a small surface. We can consider the small surface, A, as the area of the RWM where the diffusion occurs, over a period of time t, ΔC as the concentration gradient across the middle ear and inner ear cavity, and Kp as the drug permeability coefficient through the RWM.
Therefore, the process of the drug delivery through RWM can be described as follows:
(1)
Calculating absorption rate constant using Wagner–Nelson method
The Wagner–Nelson method (also known as absorbed fraction method) was used in this model to calculate the absorption rate constant (ka) from a drug concentration (C) versus time plot. In the RWM application model, at each point in time, the amount of drug absorbed (XA) is the sum of the drug amount in the perilymph (X) and the amount of drug eliminated (XE), that is:
(2)
Differentiating each term with respect to time gives us:
(3)
We will assume that the drug elimination process in the perilymph follows the first order elimination kinetics, and then the can then be defined as:
(4)
The method of residuals (also known as feathering or stripping of the curve) is used in this model to calculate the elimination rate constant (ke) from a C versus time plot. In brief, this method uses the drug elimination phase of the drug administration process to calculate the ke from the slope of the tail section of the curve of the semi-ln C versus time plot.
According to the C formula of the extravascular administration, C versus time is:
(5)
where F is the percentage of the drug absorbed, and X0 is the drug dose administered.
This equation can be rearranged as:
(6)
(7)
We assume that ka is much larger than ke. When the time t following RWM administration is large enough, ka approaches zero and therefore the equation can be simplified as:
(8)
Taking the ln of both side of the equation:
(9)
If we plot ln C versus time on a semi-log graph paper, the end of the curve will approach a straight line. Then, the slope K of the curve is:
(10)
The ke is:
(11)
We can then substitute EquationEquation (4)(4) into EquationEquation (3)
(3) .
(12)
where
(13)
In addition, V is the volume of the perilymph and C is the drug concentration in the perilymph.
Then, EquationEquation (12)(12) can be written as:
(14)
Integrating EquationEquation (14)(14) from the time point 0 to t gives us:
(15)
Integrating EquationEquation (14)(14) from the time point 0 to ∞ gives us:
(16)
The fraction of drug absorbed at time t is:
(17)
We can define the process of local drug administration via the RWM as a single-compartment model following extravascular administration. Therefore, the fraction of drug absorbed is:
(18)
EquationEquation (18)(18) can be simplified as follows:
(19)
(20)
Taking the ln of both side of EquationEquation (20)(20) :
(21)
Plotting versus time would produce a straight line and the slope of the curve is the absorption rate constant ka.
Establishing the Kp numerical equation
Transbiofilm delivery is limited by numerous factors including molecular geometry, surface charge and partition coefficient of the substance, diffusion coefficient in the membrane, and path length of the substance being transported. Among these factors, the partition coefficient (Km), the diffusion coefficient (Dm) and the path length of the drug transport (h) comprehensively affect the drug permeability (Diamond & Katz, Citation1974; Walter & Gutknecht, Citation1986). Thus, the Kp through the RWM can be obtained from the following equation:
(22)
where, h is the thickness of the RWM.
The Km is particularly difficult to measure directly experimentally. There is a functional relationship between the partition coefficient and octanol-water partition coefficient of the drug (Leo et al., Citation1971; Walter et al., Citation1986).
Therefore, the Km can be replaced by the octanol-water partition coefficient Koct:
(23)
There is an exponential correlation between the diffusion coefficient in the RWM and the drug molecular volume (MV) (La-Scalea et al., Citation2005):
(24)
where β is a constant. D0 is the diffusion coefficient while the molecular volume of the drug is zero.
By substituting EquationEquation (24)(24) into EquationEquation (22)
(22) , the following equation is obtained:
(25)
Then, EquationEquation (25)(25) can be changed as follows:
(26)
(27)
It is generally considered that the molecular volume has a linear relationship with the molecular weight (MW), therefore, the EquationEquation (26)(26) can be written as:
(28)
By substituting EquationEquation (23)(23) into EquationEquation (28)
(28) , we obtain the following:
(29)
(30)
(31)
(32)
As mentioned previously, the RWM application is considered a single-compartment model with extravascular administration. Therefore, in this model the drug transits through an ideal surface (without thickness) into the compartment (inner ear). Actually, the RWM cannot be simply regarded as a non-thickness surface and, therefore, we assumed there is a relationship between the Kp and ka as follows:
(33)
where, h from EquationEquation (33)
(33) is the thickness of RWM, which is approximately 10 μm in the guinea pig (Goycoolea & Lundman, Citation1997).
Experimental
The following five drugs: notoginsenoside R1 (R1), ginsenoside Rg1 (Rg1), ginsenoside Rb1 (Rb1), salvianolic acid B (SalB), and tanshinone IIA (TsIIA) with different physicochemical characteristics (the solubility and molecular weight of the five drugs were distributed within a certain range) were used as model drugs. They are the major bioactive components in the Chinese herbal compound – Salvia miltiorrhiza (Danshen in Chinese) and Panax notoginseng (Sanqi in Chinese). These drugs have been proven to be the responsible for the neuroprotective effects against apoptosis and protective effects against free radical-induced cell toxicity (Zhang et al., Citation2011). Thus, they may have the potential ability to treat the inner ear diseases (Cai et al., Citation2014).
For the current study, our previous in vivo experiment data were adapted to set up the trans-RWM delivery mathematical model. Hartley albino guinea pigs were euthanized for use as the experimental animal model. The dosage of drugs and administration method are described in our previous studies (Long et al., Citation2014). Micro-injectors were used to collect the perilymph samples for analysis. The levels of R1, Rg1, Rb1, TsIIA and Sal B were measured using high-performance liquid chromatography (HPLC, the measurement procedure of HPLC can be found in Supporting information). The concentration data were applied to our mathematical model and the Matlab software (version: R2012b) was used as a mathematical model-fitting tool in this study.
Results and discussions
Kp and physicochemical properties of each drug
The Kp of diffusion through the RWM was calculated using the Wagner–Nelson method (details in the Supporting information). The physicochemical properties of the drugs were obtained from the literature (Xu, Citation2003; Zhang, Citation2007; Li, Citation2008; Shi, Citation2008) and are presented in along with their Kp values.
Table 1. Permeability coefficient (Kp) and physicochemical properties of each drug.
Mathematical model fitting and parameter determination
The MATLAB function command “regress ( )” was used to fit the model and determine the parameters of the mathematical model.
First, we input the data for each drug into MATLAB, and then used the following code to fit the model:
The parameters for fitting the equation in were obtained as an automatic output from the MATLAB software after the codes had been entered.
Table 2. Equation parameter.
Then, we inserted the parameters into the fitting equation as follows:
(34)
Parametric analysis
According to the fitting equation of the numerical model, the positive value (b) of the Koct parameter suggests that the liposoluble drugs (Koct > 1; log Koct > 0) are easily transported through the RWM, which agrees with the results obtained in the experimental study showing that the drug Kp increases as the liposolubility increases (Bilej & Vetvicka, Citation1989).
The negative value (c) of the MW indicates that low Mw drugs are more easily delivered through the RWM than high Mw drugs are. Experimental studies have shown that low Mw substances such as electrolytes, dye solution, and various kinds of antibiotics are easily transported through the RWM to the inner ear (Juhn et al., Citation1989). Studies also indicate that low Mw substances are rapidly detected in the perilymph after intratympanic administration. The low c value indicates that the drug trans-RWM ability is not dependent on Mw and this is consistent with experimental results showing that macromolecular drugs can pass through the RWM. In addition, the c value was much smaller than b value, which suggests that the Mw has low influence on the drug permeability.
Based on the fitting equation, drugs that possess appropriate physicochemical properties such as low molecular weight and high lipid solubility diffuse through the RWM easily.
However, to simplify this numerical model, we assumed that the RWM acts like a semipermeable membrane and the process of the trans-RWM follows Fick’s Laws of Diffusion and therefore, active transport processes, facilitate diffusion as well as phagocytosis (all these routes have been assumed particularly for larger molecules and particles, but have not been totally confirmed so far (Goycoolea, Citation2001)) were not considered. Hence, the result of the model may not adequately describe the ability of micro/nano-particles and some large molecular weight substances such as horseradish peroxidase and monoclonal antibodies to penetrate the RWM.
Model evaluation
The function commands “[b,bint,r,rint,stats] = regress(logk,X,alpha)” was used to evaluate the mathematical model and alpha was set at 0.1. shows the statistical parameters of the mathematical fitting model.
Table 3. Statistical parameters of the mathematical fitting model.
According to the value of the coefficient of determination (R2) and coefficient of product-moment correlation (r), the fitting equation has a goodness of fit, which indicates that independent and dependent variables have a correlation with each other. Although the F value is not very large and the corresponding p > 0.05, considering the limited number of the experimental data, the results can be accepted (Zhuo et al., Citation2011).
is the residual plot that was drawn using the functional command “rcoplot(r,rint)”.
Figure 1. Residual plot of confidence interval of all data residuals 1, R1; 2, Rg1; 3, Rb1; 4, SalB; 5, TsIIA. R1, notoginsenoside R1; Rg1, ginsenoside Rg1; Rb1, ginsenoside Rb1; SalB, salvianolic acid B; TsIIA, tanshinone IIA.
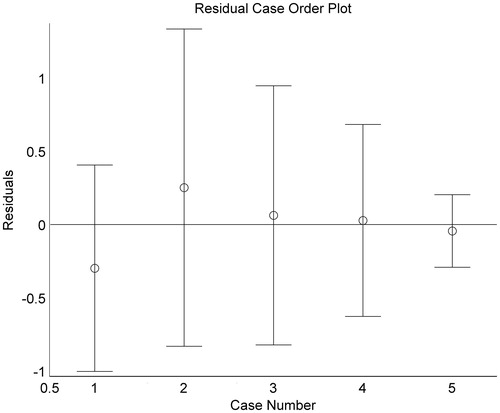
Based on the residual plot, the confidence interval (CI) of all data residuals contained the zero point in the residual distribution, which implies that the mathematical model fits the observed data well.
All these statistical parameters indicate that the mathematical model and the fitting equation fit the data obtained in the in vivo experiments. However, the model still has its limitations, which are worth mentioning. First, we were unable to ascertain the parametric assumptions of the model, normal distribution, homoscedasticity, and non-multicollinearity between the descriptors due to the limited number of samples in the experimental data (Dillon & Goldstein, Citation1984; Randic, Citation1991; Helguera et al., Citation2007). In addition, we only measured drug concentration in the perilymph and did not consider drug transport to other tissues. Therefore, this model equation will only be applicable for the prediction of the trans-RWM ability of drugs, and may not adequately describe their pharmacokinetic profiles. Furthermore, bias may have been introduced because we simplified the mathematical model by replacing the diffusion coefficient in the RWM and the drug partition coefficient with the Mw and the Koct. Finally, the numerical model may have been more precise if we had considered other factors that affect drug permeability across the RWM such as molecular geometries, pH of the drug solution and drug surface charge.
Conclusions
This study provides the preliminary results of mathematical modeling of the transport of different drugs throughout the RWM by using historical data for the assessment to show that permeability throughout the membrane was influenced by the physicochemical properties of drugs. The statistical parameters of the fitting equation indicated that the numerical model fitted the experimental data well. This mathematical model is the first to quantify the relationship between the physicochemical properties of a drug and its permeability through the RWM, and may be able to reduce the number of animals required and euthanized in related. Furthermore, an understanding of the relationship between the drug physicochemical properties and its trans-RWM ability is not only valuable for interpreting clinical treatment results, but also provides useful information for designing future drug delivery systems for local inner ear application.
Supplementary material available online
Supporting_Information.docx
Download MS Word (403.5 KB)Declaration of interest
This work was supported by the National Nature Science Foundation of China (Grant Nos. 81274097, 81573618), the Pearl River S&T Nova Program of Guangzhou (Grant No. 2013J2200059), and the Cultivation Foundation for Distinguished Young Teachers in Higher Education of Guangdong (Grant No. Yq2013099).
References
- Anonymous. (2013). EU: final ban on animal experiments for cosmetic ingredients implemented. ALTEX 30:268–9
- Bilej M, Vetvicka V. (1989). The transmembrane and intracellular transport of drugs: interactions with the cytoskeletal network. Crit Rev Ther Drug Carrier Syst 6:613–91
- Cai H, Wen X, Wen L, et al. (2014). Enhanced local bioavailability of single or compound drugs delivery to the inner ear through application of PLGA nanoparticles via round window administration. Int J Nanomedicine 9:5591–601
- Couto A, Fernandes R, Cordeiro MN, et al. (2014). Dermic diffusion and stratum corneum: a state of the art review of mathematical models. J Control Release 177:74–83
- Diamond JM, Katz Y. (1974). Interpretation of nonelectrolyte partition coefficients between dimyristoyl lecithin and water. J Membr Biol 17:121–54
- Dillon W, Goldstein M. (1984). Multivariate analysis: methods and applications. 1st ed. New York: Wiley
- Donnellan L. (2007). Animal testing in cosmetics: recent developments in the European Union and the United States; Donnellan, Laura. Animal Law 251:251–80
- Duan ML, Chen ZQ. (2009). Permeability of round window membrane and its role for drug delivery: our own findings and literature review. J Otol 4:34–43
- El Kechai N, Agnely F, Mamelle E, et al. (2015). Recent advances in local drug delivery to the inner ear. Int J Pharm 494:83–101
- Goycoolea MV. (2001). Clinical aspects of round window membrane permeability under normal and pathological conditions. Acta Otolaryngol 121:437–47
- Goycoolea MV, Lundman L. (1997). Round window membrane. Structure function and permeability: a review. Microsc Res Tech 36:201–11
- Helguera AM, Gonzalez MF, Cordeiro MN, Perez MAC. (2007). Quantitative structure carcinogenicity relationship for detecting structural alerts in nitroso-compounds. Toxicol Appl Pharmacol 221:189–202
- Hellstrom S, Johansson U, Anniko M. (1989). Structure of the round window membrane. Acta Otolaryngol Suppl 457:33–42
- Juhn SK, Hamaguchi Y, Goycoolea M. (1989). Review of round window membrane permeability. Acta Otolaryngol Suppl 457:43–8
- Kim D, Park S, Park K, et al. (2015). Development of a drug delivery system for the inner ear using poly (amino acid)-based nanoparticles. Drug Deliv 22:367–74
- La-Scalea MA, Carla MSM, Elizabeth IF. (2005). Molecular volume calculation using AM1 semi-empirical method toward diffusion coefficients and electrophoretic mobility estimates in aqueous solution. J Mol Struct Theochem 730:111–20
- Leo A, Hansch C, Elkins D. (1971). Partition coefficients and their uses. Chem Rev 71:525–616
- Li J. (2008). The preparation and the skin proliferation test in vivo of recombinant human interferon α-2b gels [master’s thesis]. Taiyuan: Shanxi Medical University
- Liu HZ, Hao JS, Li KS. (2013). Current strategies for drug delivery to the inner ear. Acta Pharm Sin B 3:86–96
- Long W, Zhang SC, Wen L, et al. (2014). In vivo distribution and pharmacokinetics of multiple active components from Danshen and Sanqi and their combination via inner ear administration. J Ethnopharmacol 156:199–208
- McCall AA, Swan EE, Borenstein JT, et al. (2010). Drug delivery for treatment of inner ear disease: current state of knowledge. Ear Hear 31:156–65
- McDodgall A. 2015. Korea proposes animal testing ban on finished cosmetics. Available at: http://www.cosmeticsdesign-asia.com/Regulation-Safety/Korea-proposes-animal-testing-ban-on-finished-cosmetics
- Pauwels M, Rogiers V. (2007). EU legislations affecting safety data availability of cosmetic ingredients. Regul Toxicol Pharmacol 49:308–15
- Plontke SK. 2015. Cochlear fluids simulator. Available at: http://oto2.wustl.edu/cochlea/model/modparams.htm
- Plontke SK, Salt AN. (2003). Quantitative interpretation of corticosteroid pharmacokinetics in inner fluids using computer simulations. Hear Res 182:34–42
- Plontke SK, Salt AN. (2006). Simulation of application strategies for local drug delivery to the inner ear. ORL J Otorhinolaryngol Relat Spec 68:386–92
- Randic M. (1991). Orthogonal molecular descriptors. New J Chem 15:517–25
- Salt AN, Plontke SK. (2005). Local inner-ear drug delivery and pharmacokinetics. Drug Discov Today 10:1299–306
- Salt AN, Plontke SK. (2009). Principles of local drug delivery to the inner ear. Audiol Neurotol 14:350–60
- Shi Y. (2008). Study of the tanshinone IIA clathrate for injection [master’s thesis]. Shenyang: Shenyang Pharmaceutical University
- Staecker H, Jolly C, Garnham C. (2010). Cochlear implantation: an opportunity for drug development. Drug Discov Today 15:314–21
- Walter A, Gutknecht J. (1986). Permeability of small nonelectrolytes through lipid bilayer membranes. J Membr Biol 90:207–17
- Walter J, Weber YPJr, Chin Rice CY. (1986). Determination of partition coefficients and aqueous solubilities by reverse phase chromatography—I: theory and background. Water Res 20:1433–42
- Xu QF. (2003). Studies on the high bioavailability of saponins of Panax notoginseng [PhD thesis]. Shanghai: Fudan University
- Yu M, Guo F, Ling Y, et al. (2015). Topical skin targeting effect of penetration modifiers on hairless mouse skin, pig abdominal skin and pig ear skin. Drug Deliv 22:1053–8
- Zhang LH. (2007). Study on long circulating liposomes loaded with total salvianolic acids [master’s thesis]. Sichuan: Sichuan University
- Zhang X, Chen G, Mu L. (2011). Research progress of novel dosage forms and pharmaceutical technologies for effective components of Danshen and Sanqi and their combination. Zhong Cheng Yao 33:1568–70
- Zhuo JW, Wei YS, Qing J, Li BW. (2011). Application of MATLAB in mathematical modeling. 1st ed. Beijing: Beihang University Press