Abstract
Context: Aceclofenac (ACE) is a systematically designed drug, developed to circumvent the concerns associated with diclofenac. But ACE is also associated with non-steroidal anti-inflammatory drug (NSAIDs)-tagged side effects, although of decreased amplitude.
Objective: The present study aims to develop phospholipid-based vesicles (elastic membrane vesicles; EMV and ethosomes) loaded with ACE and explore their potential in topical delivery.
Methods: Elastic membrane vesicles (EMVs) were prepared by thin-film hydration method and ethosomes by cold method. The composition of both the vesicular systems was selected on the basis of vesicle density and drug entrapment. The developed systems were characterized for micromeritics, surface charge, drug entrapment, and morphology. Ex vivo permeation and retention studies on Laca mice skin were performed. In vivo pharmacodynamic evaluation was performed by tail-flick method and carrageenan-induced rat paw-edema model. During stability studies, percent drug leakage was studied.
Results: The selected ratios of phospholpid:drug:stearylamine for EMVs and ethosomes were 8:1:1 and 3:1:1, respectively. The ethosomes were found to offer more vesicle density, drug loading, and deformability index as compared with that of EMVs. The drug permeation and the retention offered by both the vesicular systems were superior to that of the conventional cream; however, performance of ethosomes superseded that of EMVs. The phospholipid-based vesicles were found to be well tolerated on mice skin. Although, the in vivo performance of ethosomes was found to be better than that of EMVs in both the studied models.
Conclusion: The phospholipid-based vesicular systems, especially, ethosomes can be a promising tool to enhance the delivery and safety of ACE by topical route.
Introduction
Osteoarthritis (OA) is the most chronic disease of the joints characterized with degeneration and inflammation of the cartilage, compromised mobility and functional disability (Reginster, Citation2002; Raza et al., Citation2014a). The clinical manifestations of the disease include morning stiffness, inflammation of the joint capsule, tenderness, and crepitus (Bjordal et al., Citation2004). The estimated worldwide prevalence of OA is about 9.6% in men and 18.0% in women and the disease is reported to severely affect the quality of life of the patients (Woolf & Pfleger, Citation2003). Orally administered non-steroidal anti-inflammatory drugs (NSAIDs) play a significant role in the symptomatic management of OA and are regarded as first-line treatment for the same (Raza et al., Citation2014a).
Aceclofenac (ACE) is an effective NSAID, frequently prescribed by the oral route for the management of OA owing to its analgesic and anti-inflammatory properties (Dooley et al., Citation2001; Pareek & Chandurkar, Citation2013). ACE inhibits the synthesis of inflammatory cytokines including interleukin (IL)-1β, tumor necrosis factor (TNF) and prostaglandin E2 (PGE2). It is also suggested that it blocks PGE2 production via COX-1 and COX-2 inhibition, after intracellular metabolism to 4′-hydroxyaceclofenac and diclofenac (Yamazaki et al., Citation1999). Although a relatively safer option than the market leader diclofenac, the administration of ACE by oral route has been associated with a number of adverse effects like dyspepsia, peptic ulcers, and gastrointestinal hemorrhage due to its acidic character (Raza et al., Citation2014a).
Apart from the pharmacological concerns, ACE also poses a number of challenges for the formulation scientists including its compromised aqueous solubility, poor chemical stability, and appreciably higher lipophilicity (Bhinge et al., Citation2008). These challenges associated with ACE pave a path for further research to explore the alternative routes of administration. Out of numerous routes of administration, topical route is the most preferred one (Altman & Barkin, Citation2009). Topical delivery of ACE seems to be a lucrative option on account of its advantages like ease of application, reduction in dosing frequency, and also reduced chances of occurrence of side effects (Raza et al., Citation2014a; Dasgupta et al., Citation2013). A number of approaches have been proposed in the past to improve the drug uptake in skin from topical formulations (Raza et al., Citation2011). One such approach is the exquisitely structured elastic membrane vesicles (EMVs) made up of phospholipids and surfactants which have shown great potential in improving the performance of drug molecules by way of modifying the release and permeation of drugs (Cevc et al., Citation1998; Raza et al., Citation2011; Bragagni et al., Citation2012). Another approach is the ethanolic phospholipid-based vesicles, commonly known as ethosomes. The high flexibility of ethosomes due to the presence of ethanol permits the vesicles to readily squeeze through the skin pores, thereby enhancing the therapeutic efficacy (Elsayed et al., Citation2006). These vesicular formulations are reported to enhance the drug permeation and its localization in deeper layers of skin, which otherwise cannot be achieved with conventional formulations, as the latter get restricted to the upper harder and tougher layers of stratum corneum (Verma & Pathak, Citation2012).
Henceforth, the current study focuses on developing ACE-loaded elastic membrane vesicles and ethosomes for its topical delivery and to assess the prospective of these lipidic carriers for the management of pain and inflammation.
Materials and methods
Materials
ACE and phospholipids (Phospholipon® 90G, PLG; Phospholipon® 90H, PLH; and Lipoid S-100, PLS) were obtained ex-gratis from M/s Ipca Laboratories Ltd., Mumbai, India, and M/s Phospholipid GmBH, Nattermannallee, Germany, respectively. Carbopol® 940 was obtained as a gift sample from M/s Panacea Biotec Ltd., Lalru, Punjab, India. Sephadex G-50 (medium) was procured from M/s Sigma-Aldrich, St. Louis, MO. Polycarbonate membranes were procured from M/s Whatman, Kent, UK. All other chemicals and reagents used were of analytical grade. Double distilled water was employed throughout the study.
Methods
Preparation of ACE-loaded EMVs
ACE-loaded EMVs were prepared by thin film hydration method (Cevc, Citation1997; Raza et al., Citation2011). Briefly, accurately weighed quantities of drug, PLH and surfactant were dissolved in minimum amount of chloroform in a round bottom flask. The solvent was evaporated at 60 ± 2 °C under reduced pressure (at 100 rpm) using a rotary film evaporator (Laborata 4011 Digital, M/s Heidolph, Schwabach, Germany). After complete evaporation of the solvent, the flask was kept overnight under vacuum to remove the residual solvent. The obtained thin lipid film was hydrated at 50 ± 2 °C with aqueous phase (normal saline) using a rotary film evaporator (at 60 rpm) to obtain a homogenous suspension of EMVs. The suspension was kept for 2 h at room temperature for complete hydration, before conducting any further characterization studies (Cevc, Citation1996; Jain et al., Citation2007).
Preparation of ACE-loaded ethosomes
ACE-loaded ethosomes were prepared by the cold method (Jain et al., Citation2004). Briefly, PLG (5%, w/w) and drug (1.5%, w/w) were dissolved in ethanol (20–50%, v/v). To the alcoholic solution, double-distilled water was added with constant mixing at 700 rpm employing a magnetic stirrer. Mixing was continued for an additional 5 min. The system was maintained at 30 °C throughout the procedure (Touitou et al., Citation2000; Pandey et al., Citation2015).
Selection of composition
Elastic membrane vesicles
PLH was employed for the formulation of EMVs and its mass ratio with drug was varied from 6:1 to 10:1 to decide upon the optimum PLH: drug ratio. In these studies, 10 mg of stearylamine (SA) was employed as charge inducer per 10 mL of the suspension. After selection of appropriate PLH:drug, selection of edge activator was done, out of Span 80 (SP), Tween 80 (TW), and (1:1, w/w) ratio of Span 80:Tween 80 (SP:TW). A mass ratio of PLH to edge activators was varied from 95:05 to 75:25 with a constant amount of SA, i.e. 1 mg/mL. Subsequent to that, an optimum ratio of PLH:ACE:SA was determined out of the studied mass ratios from 8:1:0.5 to 8:1:2. All the ratios were selected on the basis of vesicle density and drug entrapment (Hussain et al., Citation2015b).
Ethosomes
PLG was employed for the formulation of ethosomes. Various PLG:ACE ratios were studied, ranging from 1:1 to 6:1 to select the optimum PLG amount. For the determination of amount of charge inducer, various ethosomal suspensions were prepared with PLG:ACE:SA ratios ranging from 3:1:0.5 to 3:1:2. For selection of PLG:ACE ratio and PLG:ACE:SA ratio, 20% v/v ethanol was employed. Ethanol amount was optimized by developing formulations with fixed PLG:ACE:SA ratio as 10:1:1, varying ethanol amount, i.e. 20–50%. The selections were made on the basis of vesicle density and drug entrapment (Sarwa et al., Citation2015b).
Characterization of the developed systems
Particle size and size distribution
Dynamic light scattering (DLS) was employed to determine the vesicle size and the size distribution of the developed vesicles. Vesicular suspension, 1 mL, was diluted to 10 mL with normal saline and size of the vesicles was determined using Delsa™ Nano (M/s Beckman Coulter, India Pvt. Ltd., Mumbai, India) installed at Institute of Microbial Technology; IMTECH, Chandigarh, India. The mean value of three repeated measurements for each sample was reported as the final observation (Zakir et al., Citation2010; Negi et al., Citation2015b; Hussain et al., Citation2016).
Zeta potential
Analysis of zeta-potential of the undiluted vesicular suspension was carried out on Delsa™ Nano (M/s Beckman Coulter, India Pvt. Ltd., Mumbai, India) installed at IMTECH, Chandigarh, India. The measurements were done at 25 °C at electric field strength of 23.2 V/cm (Negi et al., Citation2015b).
Morphology and structure of vesicles
The morphological characterization, namely shape, uniformity, and aggregation in the prepared vesicular carrier systems were monitored by optical microscopy and transmission electron microscopy (TEM). The prepared vesicles were examined under an optical microscope (Eclipse 80i, M/s Nikon Corporation, Tokyo, Japan) at a suitable magnification of 100×. The structure of the vesicles was also determined by transmission electron microscope (JEOL Ltd., JEM-1230, Tokyo, Japan) installed at Central Instrumentation Lab, Panjab University, Chandigarh, India. A drop of diluted sample was placed on the surface of carbon-coated copper grid and stained with a drop of (1%, w/v) aqueous solution of phosphotungstic acid. The thin film formed on the surface of copper grid was viewed under the microscope at a suitable magnification (Jain et al., Citation2016).
Number of vesicles
The vesicular suspensions were diluted five times with normal saline and the number of vesicles per cubic mm was counted microscopically employing a hemocytometer. The vesicles in 80 small squares were counted and the vesicle density was calculated using the formula mentioned in EquationEquation (1)(1) (Raza et al., Citation2011):
(1)
Degree of deformability
The deformability index was determined using a vesicle extruder (M/s Eastern Machine Inc., Westminster, MD). Briefly, the vesicular suspension was passed through polycarbonate filter of 50 nm pore size employing vesicle extruder. The vesicle size was measured before and after the extrusion by differential light scattering measurements using Beckman Coulter Delsa™ Nano. Percent deformability was calculated using the following formula (Raza et al., Citation2011):
(2)
where average PE is the particle size before extrusion (n = 3) and PA is the average particle size after extrusion (n = 3).
Drug entrapment efficiency
Mini-column centrifugation technique was employed for the removal of unentrapped drug (Fry et al., Citation1978). The prepared columns were pre-saturated with plain (i.e., without drug) vesicular systems and further, drug-loaded vesicular suspension (0.2 mL) was placed in the column. The centrifugation was carried out at 2000 rpm for 3 min. The eluted vesicles were observed under the microscope for the absence of any unentrapped drug. The eluted vesicular suspension was treated with chloroform–methanol (2:1, v/v) mixture and the solution was analyzed at 275 nm by a validated reverse phase high-performance liquid chromatographic (RP-HPLC) method. In brief, an automated HPLC system (LC-2010CHT, Schimadzu, Tokyo, Japan) was employed equipped with a UV detector and a software LC solution through a reverse phase C-18 column of length 250 × 4.6 mm, 5 μ ODS1 Spherisorb (M/s Waters Corporation, Milford, MA). The mobile phase composed of methanol:water (70:30, v/v) delivered at a flow rate of 1 mL/min was employed.
Development of secondary topical vehicle
The vesicular systems were dispersed in Carbopol® 940 hydrogel (1%, w/v) as gelling agent to make them rheologically acceptable for topical applications. Incorporation of the vesicular suspension was carried out by gentle levigation with Carbopol® 940 gel base in an appropriate amount, resulting in complete gelling. After complete mixing and hydration, the pH was adjusted to 6.0 by drop-wise addition of (10%, w/v) triethanolamine solution.
Preparation of conventional cream of ACE
For various comparisons, ACE-loaded conventional cream was also formulated. The conventional cream was composed of 1.5% ACE, 6% sorbitan mono-oleate, 3% white bees wax, 36% white soft paraffin, 15% liquid paraffin, and quantity sufficient of distilled water. The conventional cream was formulated by simple melting and mixing the ingredients under agitation.
Ex vivo permeation studies
The permeation characterization of the vesicular formulations was carried out on Franz diffusion cells (M/s Perme Gear Inc., Hellertown, PA). The ex vivo permeation experiments were conducted on excised abdominal skin of Laca mice (Agarwal et al., Citation2001). All the animal protocols were duly approved by the Institutional Animal Ethics Committee, Panjab University, Chandigarh, India (Ref. letter no. 5622/PS).
The various prepared formulations, namely EMV suspension and gel, ethosomal suspension and gel, drug solution in PBS 7.4: ethanol (70:30, v/v) and conventional cream containing 1.5% equivalent to 5 mg of ACE were applied onto the shaven Laca mice skin mounted on the interface of the donor and the receptor compartments (a cross-sectional area of 3.142 cm2 and a receptor volume of 30.0 mL). The receptor compartment was composed of phosphate buffer saline, (PBS 7.4): ethanol (70:30, v/v) as a diffusion medium maintained at 32 ± 1 °C, while the diffusion medium was stirred continuously using a magnetic stirrer (Sharma et al., Citation2015; Wadhwa et al., Citation2015). An aliquot of 1 mL each was withdrawn through the sampling port at suitable intervals and replaced with an equal volume of fresh diffusion medium to maintain constant receptor volume and proper sink conditions. The samples were analyzed for drug concentration by RP-HPLC. The average flux values were calculated by dividing the amount of drug permeated by the cross-sectional area as well as the time span of the study. For comparison, the theoretical flux with maximum possible retention of 50% was calculated and fixed as 26.53 μg. cm−2 h−1.
Drug retention studies in skin
The skin sections mounted on the diffusion cells during skin permeation studies were removed and washed thrice with saline and dried using lint free cotton swab. Subsequently, the clean skin was chopped in pieces and added to 10 mL ethanol and shaken for 24 h at 32 ± 1 °C for complete extraction of drug (Negi et al., Citation2015a). The supernatant was filtered through 0.45 μm membrane filter and analyzed for the drug content by RP-HPLC.
Skin safety studies
Male Laca mice (4–6 weeks old; 15–20 g) were divided into four groups, each group consisting of six animals, hair on the dorsal side of mice were removed with the help of a 0.1 mm animal hair clipper. The skin was wiped three to four times with a saline wetted cotton swab and after drying; the formulations (equivalent to 1.5% of ACE) were topically applied once-a-day for 7 d. On one hand, Groups 1 and 2 received topical application of EMV and ethosomal hydrogel, while Group 3 received conventional cream. Group 4, on the other hand, was treated only with saline, which served as the control. After exposure, the formulation remaining on skin was removed carefully and swabbed with saline soaked cotton. The animals were then sacrificed by cervical dislocation; skin samples were removed and fixed in 10% formalin solution. The skin samples obtained were stained with hematoxylin and eosin and observed microscopically for any histopathological changes (Raza et al., Citation2013; Hussain et al., Citation2015a).
In vivo pharmacodynamic evaluation
Radiant heat tail-flick method
Radiant heat tail-flick latency test using analgesiometer (IMCORP, Ambala, India) was employed for evaluating analgesic effect of the prepared formulations. The test was carried out on male Laca mice. The animals were divided into four groups of six mice each (control, conventional cream, EMV, and ethosomal gel treated). After application of formulation (0.5 g) on the tail of mice, the study was initiated. As a standard protocol, the cut-off for the basal reaction time was fixed as 10 s. The reaction time was measured at various time-intervals (15, 30, 45, 60, 90, and 120 min) for each group (Kulkarni, Citation1999; Raza et al., Citation2014b; Negi et al., Citation2015b). The percent analgesic effect was determined using the following equation:
(3)
Carrageenan-induced rat paw edema
The anti-inflammatory activity of prepared formulations was determined by carrageenan-induced rat paw edema model. The test was carried out on male Wistar rats (170–190 g). The animals were divided into four groups (control, conventional cream, EMV, and ethosomal gel treated) of six animals each. Animals were injected sub-plantarly 0.1 ml of 1.0% carrageenan solution, in saline into the left hind paw of the animals (Patil et al., Citation2010). Thereafter, the animals were left for 1 h for the induction of inflammation on the paw. After 1 h, each formulation (0.5 g) was applied topically on paw and the paw volume was measured by volume-displaced method using a Plethysmometer (UGO-Basile, Varese, Italy) before after the injection of carrageenan at 0, 1, 2, 4, 6, 8, 10, and 12 h after application of formulations. The percentage increase in paw volume from time zero was calculated (Kulkarni, Citation1999). The percent swelling of the paw was determined using the following equation:
(4)
where V is the paw volume at different time intervals, after the carrageenan injection and V0 is the initial paw volume at time zero.
The average percent paw swelling in the formulation treated group was compared with the control group and the percent inhibition of the edema was determined using the following equation:
(5)
where PSt is the percent swelling of formulation treated group and PSe is the percent swelling of control group.
Stability studies
The prepared vesicular systems were subjected to stability studies at different storage temperatures namely 6 ± 2 °C, 30 ± 2 °C and 45 ± 2 °C for a period of 6 weeks in sealed ampoules. The samples were charged for stability evaluation and after every 7th day, percent entrapment of the drug was determined, as per the method described in entrapment studies (ICH, Citation2003).
Statistical analysis
The results were statistically analyzed using one-way ANOVA followed by post hoc analysis using Student’s t-test. Statistical significance was considered at p < 0.05.
Results and discussion
Selection of composition
ACE-loaded EMVs
Out of various studied ratios of PLH:ACE, the formulation with ratio 8:1 offered maximum vesicles i.e. 72.30 × 103 ± 1.21 × 103 vesicles/mm3 and a maximum drug entrapment of 61.14 ± 1.09%. After this ratio, both the vesicle density and the drug entrapment were observed to decrease. The decrease in vesicle density as well as entrapment after PLH:ACE ratio of 8:1 can be ascribed to incomplete hydration of phospholipids (Mishra et al., Citation2007; Raza et al., Citation2011). Out of the various studied edge activators, SP was observed to offer maximum desirable attributes to the EMV dispersions. The formulation containing the PLH:SP ratio of 95:05 offered maximum vesicles of the order of 74.70 × 103 ± 2.13 × 103 vesicles/mm3 and a drug entrapment of 66.21 ± 2.18%. The studies with charge inducer (SA) revealed that the mean ratio of 8:1:1 (PLH:ACE:SA) offered maximum vesicle density, i.e. 75.3 × 103 ± 2.21 × 103 vesicles/mm3 and a drug entrapment of 67.61 ± 1.04%. Henceforth, the final formulation of EMVs was composed of phospholipid, drug and charge inducer in the mass ratio of 8:1:1.
Ethosomes
Out of various studied mass ratios of PLH:ACE, the formulation with ratio 3:1 was selected. This composition offered maximum vesicle density, i.e. 175.30 × 103 ± 9.43 × 103 vesicles/mm3 and a maximum drug entrapment of 75.12 ± 3.21%. The decrease in drug entrapment after 3:1 mass ratio can be ascribed to the saturation of the phospholipid layers and hydro-alcoholic chamber with the drug.
The formulation consisting of PLG:ACE:SA ratio as 3:1:1 was found to offer maximum vesicle density of 181.50 × 103 ± 11.21 × 103 vesicles/mm3 and a maximum drug entrapment of 75.12 ± 3.21%. However, further increase in the amount of SA did not offer any significant effect on the entrapment efficiency, hence, this composition was selected for further characterization. Out of the various studied ethanolic concentration, 20% v/v was selected, as it offered visible vesicles at 100× by optical microscopy.
Formulation characterization
Particle size and size distribution
depicts the pattern of particle size distribution of the selected formulations. A mean particle size of EMVs was 475.21 ± 10.23 nm (PDI = 0.216) and that of ethosomes was 135.42 ± 8.27 nm (PDI = 0.134), respectively. The lower values of PDI ensured that the vesicle population was relatively homogenous in size.
Zeta potential
The observed zeta potential of the final formulation was +15.13 ± 2.34 mV for EMVs and +22.81 ± 4.73 mV for ethosomes, respectively. The zeta-potential value for EMVs does not advocate the suspension stability; however, this moderate value may impart substantial stability in gel form. The ethosomal suspension offered substantial zeta potential value for suspension as well as gel stability (Barupal et al., Citation2010).
Morphology and structure of vesicles
As shown in , EMVs were found to be multilamellar in nature and relatively uniform in size. They were observed to be spherical in shape. The population of vesicles was dense with no visible aggregates. Optical microscopy of ethosomes as shown in revealed their multilamellar nature with uniform shape and size. TEM photomicrographs as shown in , although do not allow visualization of the lamellae, however, assure the size uniformity and symmetry of the vesicles.
Number of vesicles and drug entrapment
portrays the data for vesicle density and drug entrapment for both the selected formulations. Ethosomes offered more entrapment efficiency and vesicle density than that of EMVs. It can be ascribed to the alcoholic solubility of drug as well as phospholipid. The presence of ethanol results in dissolution of phospholipid and reduced surface tension, resulting in small vesicles, which may be the underlying reason for higher vesicular density and drug loading.
Table 1. Number of vesicles, percent transmittance and drug entrapment of ACE-loaded EMVs and ethosomes.
Degree of deformability
The percent deformation in EMVs was observed to be 47.31 ± 7.49%, whereas for ethosomes, it was 5.10 ± 0.22%. This indicates that the ethosomes offered maximum resistance to the mechanical deformation and were able to maintain the vesicle integrity vis-à-vis the EMVs. This property is regarded as one of the crucial attributes for elastic vesicles for penetration by topical route (Honeywell-Nguyen & Bouwstra, Citation2005). Hence, it can be regarded that ethosomes can penetrate the stratum corneum intact than that of EMVs.
Ex vivo permeation studies
shows the drug permeation profile of the studied formulations across Laca mice skin. It is vivid from the profile that the drug solution was able to cross the skin in substantial amount; however, the vesicular systems controlled the release of drug across the skin. The permeation of drug followed the following order:
Figure 4. Percutaneous permeation profile of the selected formulations and other reference products. Each cross bar indicates average value ± SD (n = 3).
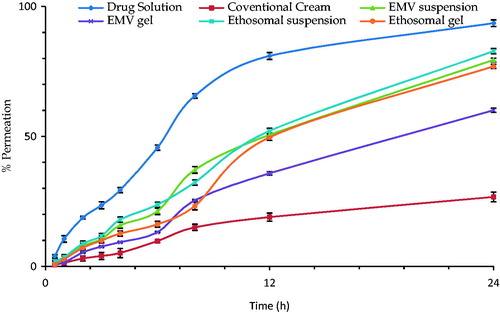
ACE solution (54.04 ± 2.23 μg cm − 2 h − 1) > Ethosomal suspension (46.34 ± 3.13 μg cm − 2 h − 1) > Ethosomal gel (45.68 ± 2.23 μg cm − 2 h − 1) > EMV suspension (42.16 ± 1.77 μg cm − 2 h − 1) > EMV gel (32.84 ± 2.37 μg cm − 2 h − 1) > Conventional cream (20.62 ± 0.98 μg cm − 2 h − 1).
Hydroalcoholic solution offered least resistance by the skin components and the drug was able to reach the receptor compartment in substantial amounts (Verma & Pathak, Citation2012). Phospholipid-based vesicular systems offered greater flux than the conventional cream, but less than the drug solution, due to the presence of drug in the interiors of vesicles. However, the developed systems, i.e. the ethosomes and EMVs, as well as the respective gels were able to offer the target permeation flux of 26.53 μg cm−2 h−1.
Drug retention studies in skin
shows the drug retention in mice skin after the permeation studies. The following order was obtained: Ethosomal gel (15.45 ± 1.23%) > ethosomal suspension (14.49 ± 0.98%) > EMV gel (13.28 ± 0.79%) > EMV suspension (11.89 ± 0.69%) > conventional cream (2.10 ± 0.13%) > ACE solution (0.94 ± 0.02%).
Figure 5. Bar diagram showing the mean percent drug retention in skin from various formulations. Each cross bar indicates average value ± SD (n = 3).
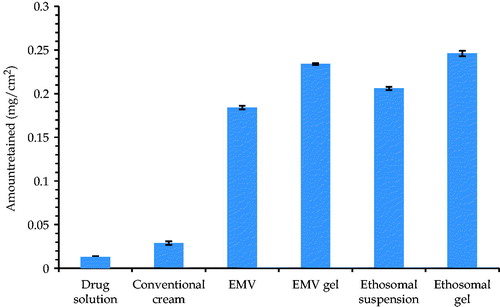
For topical products, drug retention in the skin is the most important and desired parameter. The developed carriers offered substantial retention in skin vis-à-vis conventional cream as well as ACE solution (p < 0.01). However, the drug deposition values offered by ethosomes were slightly higher than that of the EMVs, plausibly due to the smaller size and higher drug loading Henceforth, the developed systems offer the promise of depot drug reservoirs for sustained drug delivery and minimal undesired transdermal drug delivery (Sarwa et al., Citation2015a).
Skin safety studies
The photographs of mice receiving various treatments are shown in . It is clear from the pictures that the animal group receiving conventional gel showed marked signs of skin inflammation. However, the skin samples of the groups administered with the EMV and ethosomal gel did not represent such signs and were observed to be analogous to the control group. Similar results were obtained from the histopathological studies, as shown in . Irritation in mice skin receiving conventional gel might be due to the free carboxyl group of ACE (Raza et al., Citation2014a), whereas the EMVs and ethosomes were able to mask this group and avoided the direct contact with the skin (Verma & Pathak, Citation2012). Also the employment of biocompatible phospholipids might have enhanced the biocompatibility of the drug (Fry et al., Citation1978; Bodade et al., Citation2013).
In vivo pharmacodynamic evaluation
Radiant heat tail-flick method
A significant increase (p < 0.05) in the basal reaction time in the group receiving EMV gel and ethosomal gel formulation group vis-à-vis the conventional formulation group was observed, as shown in . The study showed enhanced analgesic effect of vesicular formulations. The enhanced analgesia offered by ethosomal and EMV system can be ascribed to better skin permeation and enhanced skin deposition potential (Cevc, Citation1996). The ethosomes were found to execute better analgesic potential to that of EMVs. This can be assigned to the better deformability index of the ethosomes vis-à-vis EMVs.
Carrageenan-induced rat paw edema
The mean percent edema and % inhibition of inflammation of all the studied groups has been depicted in . The inhibition of edema was found to be highest in case of ethosomal gel formulation followed by EMV gel and least for conventional cream (p < 0.05). The results obtained were analogous to that obtained in radiant heat tail-flick model vouching the superiority of the developed systems over conventional gel. These unique findings support the hypothesis that vesicle size and deformability of the vesicles have a marked effect on the performance of topical products, despite similar composition (Trotta et al., Citation2004).
Stability studies
and show the percent drug loss from the EMV and ethosomal systems stored at various temperatures. The results show very less leakage of drug (∼3%), when the formulations were stored at lower temperatures and significantly higher leakage, when stored at higher temperatures (∼9% at 30 ± 2 °C and ∼15% at 45 ± 2 °C). An increase in temperature might have resulted in increase in the fluidity of lipoid bilayers leading to defects in the membrane packing. The increase in the leakage of drug from the ethosomes can be ascribed to the increased mobility of the drug and apparent enhanced flexibility of the phospholipid bilayers (Papahadjopoulos, Citation1978). Thus, the marked leakage at higher temperatures demands the storage conditions to be somewhat lower than the normal room temperature. Therefore, refrigerated conditions seem to be the best storage conditions for the prepared systems.
Conclusions
The present piece of work provides a scientific support for the superior performance of novel drug delivery systems over conventional drug products. The phospholipid-based vesicular formulations were able to deliver aceclofenac in a safe and effective manner by topical route. The studies provide an insight that mere achievement of nano-size and use of novel materials cannot ensure better outcomes. The phenomenon like deformability cannot be neglected for phospholipid-based vesicles to harness the maximum benefits. However, the masking of free carboxyl group of aceclofenac by strategically designed phospholipid-based vesicular systems can emerge as a promising approach towards the development of commercial topical products.
Acknowledgements
Authors are thankful to M/s Ipca Laboratories Ltd., Mumbai, for generously providing the gift samples of aceclofenac where as to M/s Phospholipid GmbH, Nattermannallee, Germany, for the ex-gratis supply of Phospholipids.
Declaration of interest
The authors confirm that this article content has no conflicts of interest. Financial grants obtained from Department of Science & Technology (DST-INSPIRE), New Delhi, India, are duly acknowledged.
References
- Agarwal R, Katare OP, Vyas SP. (2001). Preparation and in vitro evaluation of liposomal/niosomal delivery systems for antipsoriatic drug dithranol. Int J Pharm 228:43–52
- Altman R, Barkin RL. (2009). Topical therapy for osteoarthritis: clinical and pharmacologic perspectives. Postgrad Med 121:139–47
- Barupal AK, Gupta V, Ramteke S. (2010). Preparation and characterization of ethosomes for topical delivery of aceclofenac. Indian J Pharm Sci 72:582–6
- Bhinge JR, Kumar RV, Sinha VR. (2008). A simple and sensitive stability-indicating RP-HPLC assay method for the determination of aceclofenac. J Chromatogr Sci 46:440–4
- Bjordal JM, Ljunggren AE, Klovning A, et al. (2004). Non-steroidal anti-inflammatory drugs, including cyclo-oxygenase-2 inhibitors, in osteoarthritic knee pain: meta-analysis of randomised placebo controlled trials. BMJ 329:1317. doi: 10.1136/bmj.38273.626655.63
- Bodade SS, Shaikh KS, Kamble MS, et al. (2013). A study on ethosomes as mode for transdermal delivery of an antidiabetic drug. Drug Deliv 20:40–6
- Bragagni M, Mennini N, Maestrelli F, et al. (2012). Comparative study of liposomes, transfersomes and ethosomes as carriers for improving topical delivery of celecoxib. Drug Deliv 19:354–61
- Cevc G. (1996). Transfersomes, liposomes and other lipid suspensions on the skin: permeation enhancement, vesicle penetration, and transdermal drug delivery. Crit Rev Ther Drug Carrier Syst 13:257–88
- Cevc G. (1997). Drug delivery across the skin. Expert Opin Investig Drugs 6:1887–937
- Cevc G, Gebauer D, Stieber J, et al. (1998). Ultraflexible vesicles, transfersomes, have an extremely low pore penetration resistance and transport therapeutic amounts of insulin across the intact mammalian skin. Biochim Biophys Acta 1368:201–15
- Dasgupta S, Dey S, Choudhury S, et al. (2013). Topical delivery of aceclofenac as nanoemulsion comprising excipients having optimum emulsification capabilities: preparation, characterization and in vivo evaluation. Expert Opin Drug Deliv 10:411–20
- Dooley M, Spencer CM, Dunn CJ. (2001). Aceclofenac: a reappraisal of its use in the management of pain and rheumatic disease. Drugs 61:1351–78
- Elsayed MM, Abdallah OY, Naggar VF, et al. (2006). Deformable liposomes and ethosomes: mechanism of enhanced skin delivery. Int J Pharm 322:60–6
- Fry DW, White JC, Goldman ID. (1978). Rapid separation of low molecular weight solutes from liposomes without dilution. Anal Biochem 90:809–15
- Honeywell-Nguyen PL, Bouwstra JA. (2005). Vesicles as a tool for transdermal and dermal delivery. Drug Discov Today Technol 2:67–74
- Hussain A, Haque MW, Singh SK, et al. (2015a). Optimized permeation enhancer for topical delivery of 5-fluorouracil-loaded elastic liposome using Design Expert: part II. Drug Deliv 24:1–12
- Hussain A, Samad A, Ramzan M, et al. (2015b). Elastic liposome-based gel for topical delivery of 5-fluorouracil: in vitro and in vivo investigation. Drug Deliv 14:1–15
- Hussain A, Samad A, Singh SK, et al. (2016). Nanoemulsion gel-based topical delivery of an antifungal drug: in vitro activity and in vivo evaluation. Drug Deliv 23:652–67
- ICH. (2003). Stability Testing of New Drug Substances and Products Q1A(R2)
- Jain A, Thakur K, Sharma G, et al. (2016). Fabrication, characterization and cytotoxicity studies of ionically cross-linked docetaxel loaded chitosan nanoparticles. Carbohydr Polym 137:65–4
- Jain S, Umamaheshwari RB, Bhadra D, et al. (2004). Ethosomes: a novel vesicular carrier for enhanced transdermal delivery of an antiHIV agent. Ind J Pharm Sci 66:72–81
- Jain SK, Gupta Y, Jain A, et al. (2007). Multivesicular liposomes bearing celecoxib-beta-cyclodextrin complex for transdermal delivery. Drug Deliv 14:327–35
- Kulkarni SK. (1999). Experiments on intact preparations (in vivo studies). Handbook of experimental pharmacology. Pitam Pura, New Delhi, India: Vallabh Parkashan
- Mishra D, Garg M, Dubey V, et al. (2007). Elastic liposomes mediated transdermal delivery of an anti-hypertensive agent: propranolol hydrochloride. J Pharm Sci 96:145–55
- Negi P, Singh B, Sharma G, et al. (2015a). Biocompatible lidocaine and prilocaine loaded-nanoemulsion system for enhanced percutaneous absorption: QbD-based optimisation, dermatokinetics and in vivo evaluation. J Microencapsul 32:419–31
- Negi P, Singh B, Sharma G, et al. (2015b). Phospholipid microemulsion-based hydrogel for enhanced topical delivery of lidocaine and prilocaine: QbD-based development and evaluation. Drug Deliv 21:1–17
- Pandey V, Golhani D, Shukla R. (2015). Ethosomes: versatile vesicular carriers for efficient transdermal delivery of therapeutic agents. Drug Deliv 22:988–1002
- Papahadjopoulos D. (1978). Liposomes and their use in biology and medicine. Ann N Y Acad Sci 308:367–8
- Pareek A, Chandurkar N. (2013). Comparison of gastrointestinal safety and tolerability of aceclofenac with diclofenac: a multicenter, randomized, double-blind study in patients with knee osteoarthritis. Curr Med Res Opin 29:849–59
- Patil PR, Bera T, Patil VM. (2010). Improvised plethysmometer for the detection of anti-inflammatory activity of drugs. J Pharm Biomed Sci 4:1–5
- Raza K, Kumar M, Kumar P, et al. (2014a). Topical delivery of aceclofenac: challenges and promises of novel drug delivery systems. Biomed Res Int 2014;2014:1. doi:10.1155/2014/406731
- Raza K, Shareef MA, Singal P, et al. (2014b). Lipid-based capsaicin-loaded nano-colloidal biocompatible topical carriers with enhanced analgesic potential and decreased dermal irritation. J Liposome Res 24:290–6
- Raza K, Singh B, Lohan S, et al. (2013). Nano-lipoidal carriers of tretinoin with enhanced percutaneous absorption, photostability, biocompatibility and anti-psoriatic activity. Int J Pharm 456:65–72
- Raza K, Singh B, Mahajan A, et al. (2011). Design and evaluation of flexible membrane vesicles (FMVs) for enhanced topical delivery of capsaicin. J Drug Target 19:293–302
- Reginster JY. (2002). The prevalence and burden of arthritis. Rheumatology (Oxford) 41:3–6
- Sarwa KK, Mazumder B, Rudrapal M, et al. (2015a). Potential of capsaicin-loaded transfersomes in arthritic rats. Drug Deliv 22:638–46
- Sarwa KK, Rudrapal M, Mazumder B. (2015b). Topical ethosomal capsaicin attenuates edema and nociception in arthritic rats. Drug Deliv 22:1043–52
- Sharma G, Dhankar G, Thakur K, et al. (2015). Benzyl benzoate-loaded microemulsion for topical applications: enhanced dermatokinetic profileand better delivery promises. AAPS PharmSciTech. [Epub ahead of print] doi: 10.1208/s12249-015-0464-0
- Touitou E, Dayan N, Bergelson L, et al. (2000). Ethosomes – novel vesicular carriers for enhanced delivery: characterization and skin penetration properties. J Control Release 65:403–18
- Trotta M, Peira E, Carlotti ME, et al. (2004). Deformable liposomes for dermal administration of methotrexate. Int J Pharm 270:119–25
- Verma P, Pathak K. (2012). Nanosized ethanolic vesicles loaded with econazole nitrate for the treatment of deep fungal infections through topical gel formulation. Nanomedicine 8:489–96
- Wadhwa S, Singh B, Sharma G, et al. (2015). Liposomal fusidic acid as a potential delivery system: a new paradigm in the treatment of chronic plaque psoriasis. Drug Deliv 23:1–10
- Woolf AD, Pfleger B. (2003). Burden of major musculoskeletal conditions. Bull World Health Organ 81:646–56
- Yamazaki R, Kawai S, Matsumoto T, et al. (1999). Hydrolytic activity is essential for aceclofenac to inhibit cyclooxygenase in rheumatoid synovial cells. J Pharmacol Exp Ther 289:676–81
- Zakir F, Vaidya B, Goyal AK, et al. (2010). Development and characterization of oleic acid vesicles for the topical delivery of fluconazole. Drug Deliv 17:238–48