Abstract
An effective anti-cancer drug, icariside II (IS), has been used to treat a variety of cancers in vitro. However, its poor aqueous solubility and permeability lead to low oral bioavailability. The aim of this work was to use Solutol®HS15 and Pluronic F127 as surfactants to develop novel mixed micelles to enhance the oral bioavailability of IS by improving permeability and inhibiting efflux. The IS-loaded mixed micelles were prepared using the method of ethanol thin-film hydration. The physicochemical properties, dissolution property, oral bioavailability of the male SD rats, permeability and efflux of Caco-2 transport models, and gastrointestinal safety of the mixed micelles were evaluated. The optimized IS-loaded mixed micelles showed that at 4:1 ratio of Solutol®HS15 and Pluronic F127, the particle size was 12.88 nm with an acceptable polydispersity index of 0.172. Entrapment efficiency (94.6%) and drug loading (9.7%) contributed to the high solubility (11.7 mg/mL in water) of IS, which increased about 900-fold. The SF-IS mixed micelle release profile showed a better sustained release property than that of IS. In Caco-2 cell monolayer models, the efflux ratio dramatically decreased by 83.5%, and the relative bioavailability of the mixed micelles (AUC0–∞) compared with that of IS (AUC0–∞) was 317%, indicating potential for clinical application. In addition, a gastrointestinal safety assay also provided reliable clinical evidence for the safe use of this micelle.
Introduction
Icariside II (IS, also known as baohuoside I, ), a kind of natural flavonoid, is the metabolite of icariin, which is the main effective component of heat-processed Epimedium koreanum (Berberidaceae) (Song et al., Citation2015). Recently, IS has been proven to have remarkable anti-cancer properties. Arief et al. (Citation2015) proved that IS has cytotoxic and cytostatic effects on six cancer cell lines. Geng et al. (Citation2015) investigated the underlying mechanism of IS against hepatoblastoma cells in vitro and in vivo, and discovered that it can decrease the viability of HepG2 cells in a time- and concentration-dependent manner and inhibit tumor growth in mice transplanted with H22 liver carcinomas. Thus, as a promising chemotherapeutic agent, IS plays multiple roles in inducing mitochondrial and lysosomal damage that leads to cell apoptosis. In addition, Wu et al. (Citation2015) found that IS possesses anti-proliferative effects on A375 human melanoma cells. Moreover, IS can induce cell cycle arrest through activation of the ROS-p38-p53 pathway. These observations reveal that IS has great potential in cancer treatment. Because of its high pharmacological efficacy, IS has been researched in vitro and in vivo. Unfortunately, poor aqueous solubility and obvious efflux from cancer cells are two of the main limiting factors in the clinical use of IS (Chen et al., Citation2009a,b). Therefore, developing a novel formulation of IS with improved solubility would enhance its therapeutic effect.
Figure 1. (A) Chemical structure of icariside II. (B). Chemical structure of Solutol®HS15. (C). Chemical structure of Pluronics F127.
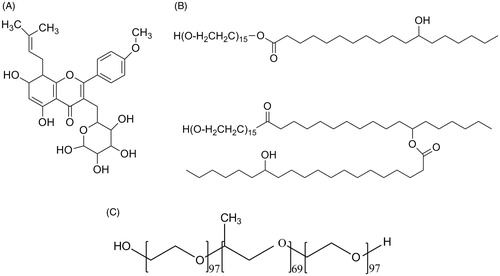
Self-assembled micelle systems are known as the main delivery method for poorly soluble drugs (Gradauer et al., Citation2015). This system has several advantages including enhancing drug solubility, ability to control drug release, avoiding reticuloendothelial system (RES) uptake, and tumor targeting ability (Wu & Guan, Citation2015). Based on the theory of synergistic effect, mixed micelles composed of two and more excipients exhibit preferable characteristics to micelles formed from a single material (Zhang et al., Citation2014). In our previous study (Li et al., Citation2012), a self-assembled mixture micelles (fatty acid-IS-bile salts) was prepared to improve oral absorption in order to strengthen the effect of warming kidney enhancing yang, but the effect was not significant (Jun et al., Citation2015).
Multidrug resistance (MDR) is one of the main challenges in developing chemotherapeutic agents. Efflux-mediated drug resistance is mainly caused by ATP-binding cassette families such as P-glycoprotein (P-gp) and breast cancer resistance protein (BCRP) (Fletcher et al., Citation2010). Efflux pumps decrease the intracellular concentration of anti-cancer drugs, reducing their therapeutic efficacy (Dehghan et al., Citation2014). IS has low absorptive permeability due to BCRP and P-gp mediating its apical efflux (Chen et al., Citation2008). Therefore, if IS is to be used clinically, suitable excipients must solve not only poor aqueous solubility, but also MDR.
To overcome the challenge of IS’ poor oral bioavailability, we aimed to establish a novel nano-drug delivery system using Solutol®HS15 (HS15) and Pluronic F127 (F127). Solutol®HS15 (), a MDR modifier, consists of a lipophilic part and a hydrophilic part, which include polyglycol mono- and di-esters of 12-hydroxystearic acid and approximately 30% of free polyethylene glycol, respectively. Furthermore, HS15 has notably high stability and solubility. It has been demonstrated that HS15 can alter plasma protein binding, enhance adsorptive behavior, and induce marked effects on the pharmacokinetics of large number of drugs (Murgia et al., Citation2013). It was originally developed as a parenteral formulation, and has not very many been reported in orally formulation. F127 is a versatile tri-block composition of PEG97-PPO69-PEG97 (). It has been widely used as a nano carrier excipient because of its safety, long circulation period, and commercial availability. F127 has also has been reported to markedly inhibit the P-gp efflux system by depleting ATP in MDR cancer cells and reversing MDR. Moreover, F127 was selected to prepare the micelles due to its biocompatibility and its approval by the FDA (Delghan et al., 2014). However, poor solubility and low stability make it difficult to use alone. In addition, F127 has poor drug-loading capacity (Li et al., Citation2015; Liu & Zhang, Citation2015; Stef et al., Citation2015).
Hence, we combined the advantages of the two pharmaceutical excipients and developed a novel binary micelles system to encapsulate IS, enhancing its aqueous solubility and permeability for improving its oral bioavailability. The influence of the mixed micelles on IS transport across Caco-2 cell monolayers and the bioavailability in SD rats were evaluated. A gastrointestinal safety assay was also carried out to ensure clinical safety.
Materials and methods
Materials and reagents
IS (purity > 98%) was supplied by the Laboratory of Pharmaceutical Preparation (Jiangsu Provincial Academy of Chinese Medicine, China). Pluronic F127 was purchased from Sigma-Aldrich (St. Louis, MO). Solutol®HS15 was purchased from BASF Ltd. (Shanghai, China). Milli-Q water (Millipore, Bedford, MA) was used throughout the study. Cloned Caco-2 TC7 cells were provided by Dr. Moniqué Rousset of INSERM U178 (Villejuit, France). Chromatographic grade methanol and acetonitrile were used (Tedia Company Inc., Fairfield, OH). All other reagents were of analytical grade.
Animals
Male Sprague-Dawley rats (200 ± 20 g) were purchased from the SLAC Lab Animal Center of Shanghai (Shanghai, China). All rats were given distilled water ad libitum and kept at a temperature of 25 °C and relative humidity of 45% for two weeks. All animal experiments were reviewed and approved by the Institutional Animal Care and Use Committee of the Jiangsu Provincial Academy of Chinese Medicine.
Preparation of IS-loaded mixed micelles
IS-loaded mixed micelles were prepared by the solvent evaporation method (Jin et al., Citation2013). Solutol®HS15 and Pluronic F127 of different proportions and concentrations were co-dissolved in alcohol by gentle agitation at 45 °C until a transparent solution formed. Then, IS dissolved in 10 mL of alcohol was slowly added dropwise to the above solution. The solution was evaporated under a vacuum of −0.1 MPa and at a temperature of 45 °C until the ethanol evaporated to dryness. 10 mL of deionized water was then added to the flask and shaken until a transparent solution formed. The mixed micelles were obtained by ultracentrifugation at 14 000 rpm for 15 min. The supernatant was retained and the stability of the mixed micelles was observed for 48 h.
Particle size and potential analysis
Particle size and zeta potential of SF-IS mixed micelles were determined by dynamic light scattering using a Malvern system (Malvern ZE S.A., Worcestershire, UK). Samples were equilibrated at 25 °C for at least 5 min prior to the analysis. The results were expressed as the average of three measurements. Then, polydispersity index (PDI) was measured for assessing the particle size distribution of SF-IS mixed micelles.
Transmission electron microscopy
The morphology of IS-loaded mixed micelles was evaluated by transmission electron microscopy (TEM, JEM-200CX, Japan). a drop of SF-IS micelles was instilled onto a carbon-coated copper grid, and then stained with a 2% aqueous solution of phosphotungstic acid. The grid was dried with infrared light for 5 min, and the sample was then observed by TEM and photographed with a digital camera.
Drug content analysis
In this study, the loading amount of IS in mixed micelles was determined by HPLC assay (Yan et al., Citation2015). The SF-IS mixed micelles were filtered with a 0.45 μm micro porous membrane, and then with 0.1 mL of precision drawing filtrate, adding an appropriate amount of acetonitrile and diluted destroyed micelles. Drug content using HPLC method drug loading (DL%) and entrapment efficiency (EE%) were calculated by the following equations. Because of its poor solubility in phosphate-buffered saline (PBS) (experimentally measured < 15 μg/mL), IS concentration in PBS is negligible compared to its concentration in micelles (>1 mg/mL). Therefore, when calculating the encapsulation efficiency and drug loading, IS free of micelles solution was ignored. DL% and EE% were calculated with the following equations:
In vitro release study
The in vitro release property of SF-IS mixed micelles was studied using the dialysis method (Zhang et al., Citation2015). To summarize, 1 mL of SF-IS mixed micelles as an experimental group and an equivalent amount of IS suspension as a control group were added to dialysis membrane bags (MWCO = 3500; Greenbird Inc., China). A receptor media comprising 200 mL PBS (pH 7.4, 37 °C) and 1% (w/v) Tween 80 (Sinopharm Chemical Reagent Co., Ltd) was freshly prepared. The completely released IS was under the leakage condition. Triplicate suspensions (500 μL) of release medium were taken out and equal volumes of fresh release medium were quickly replenished to maintain a constant volume (200 mL) at predetermined time intervals up to 72 h. Samples (n = 3) were filtered with 0.45 μm filters, and the amount of IS released in the supernatant was evaluated by HPLC analytical methods.
Caco-2 cells culture and transport experiment
The Caco-2 cell culture model, a viable model of human intestinal absorption recognized by the FDA, was selected to investigate IS absorption in this work. The culture conditions were described previously (Zhang et al., Citation2012). In summary, Caco-2 CT-7 cells were stored in an incubator (37 °C, 95% relative humidity, 5% CO2) and used for transport experiments 28-d post-seeding, which is when they are physiologically and morphologically well developed with transepithelial electrical resistance (TEER) values greater than 400Ω × cm2. When comparing the permeability, the cell monolayers were washed three times with blank HBSS (pH = 7.4). After the last addition of HBSS solution, the cell monolayers were incubated in the shock shaker with shaking for 30 min. Then, a solution-dissolved mixed micelles loaded with 20 μM IS was added to the apical (AP) or basolateral (BL) side. The donor samples (400 μL) and the receiver samples (400 μL) were taken 0, 1, 2, 3, and 4 h after incubation, then followed by the addition of 400 μL of drug solution to the donor side or 400 μL of fresh HBSS to the receiver side. Then, 100 μL of methanol containing 5 μM of testosterone was added to the sample as an internal standard. The samples were then centrifuged at 16 000 rpm for 5 min at 4 °C. The supernatant containing the transported drug was measured by the HPLC method. At the end of transport, TEER values were monitored to ensure the survival of Caco-2 cell monolayers. The rate of transport is obtained from the amount transported versus time curve using linear regression. The permeability of IS was calculated using the following equation:
Where V is the volume of the receiver (2.5 mL), S is the surface area of the cell monolayer (4.2 cm2), C is the initial concentration, dC/dt is the rate of concentration change in the receiver side, and dM/dt is the rate of drug transport. The rate of IS transport was obtained by linear regression analysis.
Pharmacokinetic studies
Male SD rats (200 ± 20 g) were divided into two groups (n = 6) at random and fasted overnight before the experiment. Group I was orally administered a dose of 100 mg/kg IS (in 0.5% aqueous methylcellulose), and Group II was treated with SF-IS mixed micelles at a dose equivalent to 100 mg/kg of IS. At predetermined times (0, 5, 15, 30, 60, 90, 120, 180, 240, 300, 360, 480, 720, and 1440 min after administration), blood samples were obtained by retro-bulbar puncture and collected into heparinized tubes for determining the IS concentrations and calculating its pharmacokinetic parameters. The blood samples were immediately centrifuged and the supernatant plasma was collected. Plasma (150 μL) was collected into tubes containing 50 μL of internal standard carbamazepine (100 ng/mL). Samples were then vortexed for 5 min. Acetonitrile (800 μL) was added to the samples and vortexed for 5 min to precipitate the plasma proteins. These samples were then centrifuged at 16 000 rpm for 15 min at 4 °C, and 800 μL of supernatant was transferred to another tube and evaporated to dryness at 20 °C. The residue was dissolved in 100 μL of methanol and vortexed for 5 min. After centrifugation at 16 000 rpm, 50 μL of the samples was injected into the UPLC-Q-TOF-MS system for determination.
Analytical method
The content of IS was determined by high performance liquid chromatography (HPLC, Agilent 1260, Agilent Co. Ltd.) with an Agilent Eclipse XDB-C18 column (4.6 × 250 mm, 5 μm). The mobile phase consisted of a 30:70 ratio of methyl alcohol and water, and the flow rate was 1 mL/min with a column temperature of 30 °C. The DAD detector was set at 273 nm to analyze the column effluent. The injection volume was 20 μL. The samples were filtered through a 0.45 μm membrane filter (Millipore Corp.). The recovery rates for IS were in the range of 99–102%, and the RSDs were less than 2%. Intra-day and inter-day precisions for IS were below 2%.
UPLC-Q-TOF/MS equipment a ZORBAX SB-C18 column (4.6 × 250 mm × 5 μm) was operated with a Waters in positive ionization mode using SCAN to measure IS at m/z 515.76-369.87, 28.78 min, and carbamazepine at m/z 237-194, 21.77 min. The stationary phase, mobile phase A, 0.05% aqueous formic acid solution; B, acetonitrile contained 0.05% formic acid, gradient, 0–3 min, 95% A, 3-5 min, 95–75% A, 5–18 min, 75% A, 18–40 min, 75%-10% A. The flow rate was 1.0 mL/min. Separation was monitored at 270 nm.
Hematoxylin-eosin (HE) staining
HE staining was performed to evaluate the gastrointestinal safety of the micelles. Two groups were orally administered 20 mg/kg of IS and SF-IS mixed micelles every 3 d for 10 weeks. The rats were sacrificed by cervical dislocation, and the stomach and intestinal (duodenum, jejunum, ileum, colon) tissue were taken and fixed in fresh 10% formaldehyde-mixing fixative for 24 h, rehydrated, and embedded into paraffin. Serial 5 μm sections were cryosectioned and then placed on superfrost/Plus microscope slides. The sections were stained with HE according to the manufacturer’s protocol, then observed and photographed with a digital camera.
Statistical analysis
All experiments were conducted in triplicate and all values are presented as mean ± standard deviation. Statistical tests of significance were performed with SPSS Statistic 18.0 (SPSS Inc., IBM, Armonk, NY). The data were analyzed by the Student’s t-test and a value of p < 0.05 was considered statistically significant between results with the control experiment.
Results
Characterization of SF-IS mixed micelles
The physicochemical characterization and drug-loading parameters of SF-IS mixed micelles are presented in . A range of single factor experiments was performed to select a better prescription. The results indicate that an increase in the relative amount of HS15 to the SF-IS mixed micelles resulted in an increase in drug solubility. The EE value and average size of the SF-IS micelles showed little change. The optimized mixed micelle showed that at a 4:1 ratio for HS15: F127, the mean diameter of the mixed micelles was 12.88 nm with an acceptable PDI of 0.172 (seen in ). The morphology of the SF-IS mixed micelles is shown in . HS15 and F127 can form self-assembled micelles with a spherical shape and good dispersion in this novel nano-drug delivery system. Zeta potential (−27.4 mv) contributes to high stability. The amount of IS in the SF-IS mixed micelles was determined. The results showed that these mixed micelles contributed to the high solubility (11.7 mg/mL in water) of IS, which increased about 900-fold.
Figure 2. (A) Size distribution of SF mixed micelles as determined by DLS. (B) TEM images of SF-IS mixed micelles.
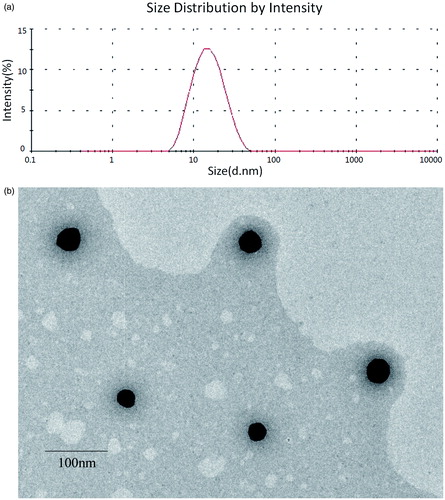
Table 1. HS15/F127 ratio and characteristics of SF mixed micelles.
In vitro release
In the methods, the instantaneous release rate is measured as the release media removed and replaced infinitely quickly. The IS concentration was calculated from the integral of the release rate. The in vitro release profile of IS loaded micelles and IS are presented in . In the first 3 h of the study, in simulated intestinal medium under sink conditions at pH 6.8, IS showed a burst release of 29.9% while the micelles released at a slow rate. Moreover, micelles released smoothly without a burst effect, and the cumulative amount of release was 61.6% at 72 h. However, the IS group showed a cumulative amount of release of 79.1% at 72 h. The IS release profile of the SF-IS mixed micelles showed a better sustained-release property than IS.
Transport of IS and mixed micelles across Caco-2 cell monolayers
In this experiment, the transport of IS (20 μM) and SF-IS mixed micelles in both apical (AP) to basolateral (BL) and BL to AL directions were investigated, as shown in and . For AP-BL transport, the PappAB value of IS was 1.97 × 10−6 cm/S. IS-loaded micelles significantly (p < 0.05) increased the absorptive direction (AP-BL) transport of IS (3.94 × 10−6 cm/S). BL-AP permeability (Papp) of mixed micelles (4.82 × 10−6 cm/S) compared to the control (15.24 × 10−6 cm/S) showed a significant decrease, indicating that the SF-IS mixed micelles can markedly inhibit the P-gp efflux system. Compared with the absorption and efflux of IS, forming micelles could promote absorption by 200% and reduce efflux by 316%. This could mainly be due to the characteristics of pharmaceutical excipients HS15 and F127. Therefore, using these two excipients could increase the absorption of IS and inhibit efflux.
Figure 4. (A) IS. (B) SF (4:1) mixed micelles. Effects of micelles in permeability of IS (20 μM). Absorptive permeability is expressed as Papp (AP-BL) and secretory permeability is expressed as Papp (BL-AP). Date are presented as mean ± SD (n = 3). The asterisk symbol indicates a statistically significant difference. The number of asterisk symbol indicates the level of significance with **p < 0.01. One-way ANOVA with Tamhane’s post hoc was used to analyze the data statistically.
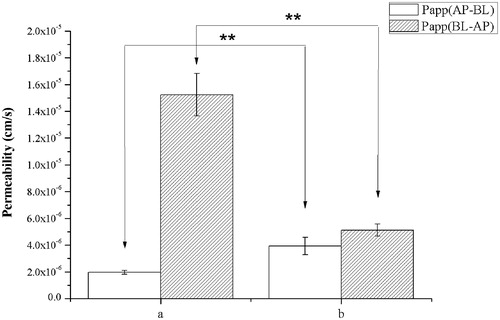
Table 2. Permeability and efflux ratio.
Pharmacokinetic studies
and show the plasma drug concentration-time curve of IS and SF-IS mixed micelles after a single oral administration of 100 mg/kg of IS and micelles in rats (n = 6). The average values of Cmax are 2553.79 and 538.55 ng/mL with Tmax values of about 1.07 and 0.56 h in the micelles group and the control groups, respectively. This indicates that forming micelles could markedly increase IS bioavailability. In addition, there was no significant difference in the time taken to reach peak concentration (Tmax) of IS between two groups (Tmax = about 1 h in both groups), but t1/2 showed a significant difference (p < 0.01), indicating a slow-release property. The average values of the AUC0–∞ of IS and micelles were 4059.81 and 12 886.76 μg/Lċh, respectively. The mixed micelle group was markedly higher than the control group, and the relative bioavailability of the micelles (AUC0–∞) compared with IS was 317%, demonstrating great potential for their clinical use.
Figure 5. The plasma concentration-time curve of IS in rats after oral administration of IS, SF mixed micelles (100 mg/kg, IS). Data are presented as mean ± SD (n = 6).
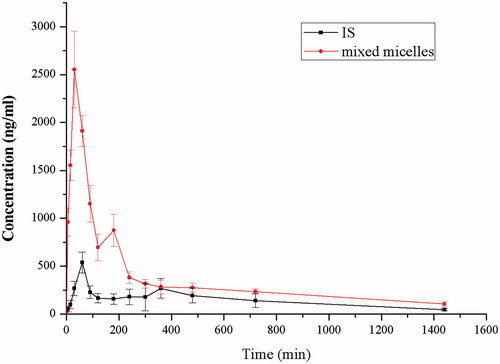
Table 3. Pharmacokinetic parameters of IS, SF mixed micelles (100 mg/kg, IS).
Gastrointestinal safety assay
As depicted in , the gastric wall is composed of four layers. From innermost to outermost, they are the mucosa, the submucosa, the muscularis propria, and the serosa. The monolayer columnar epithelial cells constitute the mucosa layer, while the gastric gland is located in the lamina propria. The stomach wall is typically covered by stratified squamous epithelium. The administration of API could cause a great degree of pathological change, and can cause the surface of the gastric wall to appear slightly keratinized. It is the connective tissue beneath the mucosa layer. Moreover, in the API group, mild congestion and moderate proliferation but not cellular heteromorphosis can be observed in the lamina propria.
Figure 6. Gastrointestinal safety assay observed by HE staining. (A) APIs used in this study; (B) SF-IS mixed micelles used in this study (1–5) stomach and intestinal (duodenum, jejunum, ileum, colon).
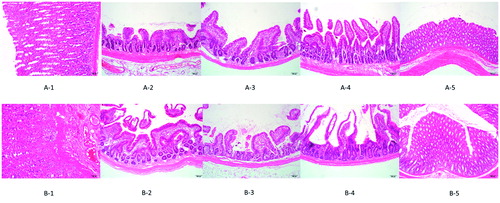
The intestine is made up of the mucosa, the submucosa, the muscularis propria, and the serosa in sequence, and each layer possesses an explicit structure. The neatly arranged mucosa is covered by monolayer columnar epithelial cells and forms the thin intestinal villi by bulging toward the enteric cavity. The intestinal gland is situated in the lamina propria. Under the mucosa is loose connective tissue. The muscularis propria is comparatively thick, and the serosal layer consists of mesothelial cells. There was no prominent lesion in all parts of the intestinal tract. Nevertheless, as depicted in , the intestinal villi were shortened and emerged relatively loose. Furthermore, there was no inflammatory cell infiltration. All results demonstrated that although the preparation used in the experiment mildly stimulates the intestine, it has no apparent toxicity.
Discussion
Many drug candidates are highly therapeutic but have low solubility in water, which poses a challenge for its in vivo delivery. IS has various pharmacological activities and a good safety profile, but poor oral bioavailability is the biggest limiting factor in its clinical use. The main reason for this problem lies in its extremely low aqueous solubility. In our previous study (Jin et al., Citation2013), efflux via the BCRP, multidrug resistant-associated protein 1 and 2 (MRP1 and MRP2) and P-gp lead to IS’ low absorptive permeability and restricted its application. To overcome this difficulty, excipients and preparations can be incorporated into the drug to alter its ADME (absorption, distribution, metabolism, excretion) properties. Despite there being literature discussing strategies to increase the solubility of IS, there are few studies that focus on oral formulations. In the present study, we developed a novel SF-IS mixed micelle composed of HS15 and F127, aiming to improve the poor absorption of IS. F127 was selected to prepare the micelles due to its biocompatibility and its approval by the FDA (Kwon et al., Citation2014). HS15 has several advantages, such as a good solubilizing effect, high stability, and low toxicity. The results indicate that at a 4:1 ratio of HS15:F127, the mixed micelles significantly increased the oral bioavailability and markedly inhibited the efflux ratio of IS. These excipients’ presence greatly increases the solubility of IS to 11.7 mg/mL, increasing about 900-fold compared with IS. In addition, when the amount of HS15 is increased, the SF-IS mixed micelles’ drug-loading capability and stability increase as well. In this SF-IS mixed micelle (), HS15 and F127 dissolve in water, and amphiphilic molecules gather by driving forces and form molecular aggregates with a crimped hydrophobic core. The same hydrophilic chain promotes the formation of micelles. Furthermore, the critical micelle concentration (CMC) of HS15/F127 solution is interposed between HS15 and F127 (Wu et al., Citation2013), which indicates that the stability of SF-IS mixed micelles is greater than unified micelles. Moreover, nanoparticles with sizes ranging from 10 to 100 nm could effectively avoid being metabolized by the liver or filtered out by the kidneys (De et al., Citation2008; Yu et al., Citation2010). Therefore, these SF-IS mixed micelles with particle sizes of 12.88 nm could effectively avoid rapid metabolism and elimination, and guarantee the stability and long circulation time (Zhang et al., Citation2014). In addition, HS15 and F127 can markedly reduce IS efflux and improve the concentration of the drug in cells which could enhance permeability and retention (ERP) effect and passive targeting ability (). Under TEM, SF-IS mixed micelles presented a well-formed spheroidal shape with no conglutination. This core-shell structure was due to electrostatic repulsion, steric hindrance, and amphiphilic block interactions.
Figure 7. (A) Schematic illustration of forming progress of SF-IS mixed micelles. (B) Schematic illustration of SF-IS mixed micelles inhibit the efflux system.
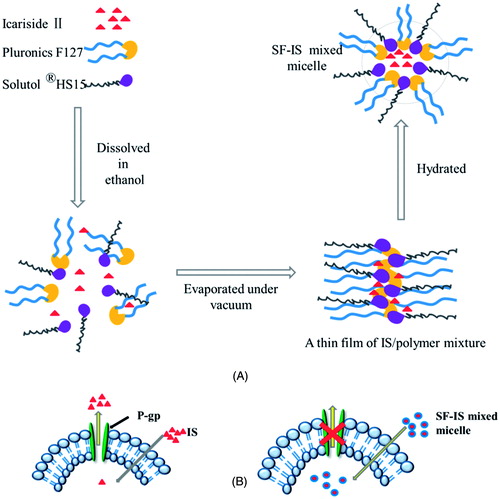
In this study, Caco-2 transport models were applied. Before performing transport studies, TEER values (>400Ω/cm2) were measured to ensure the cell-seeded inserts were tight and junctions and ready for the transport study. At the end of experiment, the TEER values (RSD < 5%) were calculated to ensure that the drug and preparations are not toxic to cells. The absorption properties of IS and mixed micelles showed notable differences, indicating that the mixed micelles can significantly inhibit efflux. The results show that (1) both PappAB and accumulation of IS in mixed micelles remarkably increased compared with the active pharmaceutical ingredient (API), and consequently, the efflux ratios was 3.2-fold higher than API; (2) both the PappBA and efflux of API were significantly higher than mixed micelles; (3) the SF-IS micelles can increase intestinal absorption of IS. The mechanism of this phenomenon may lie in the inclusion of the two excipients.
Furthermore, SF-IS mixed micelles’ higher relative bioavailability after oral administration may be due to the following reasons: (1) HS15 and F127 form a formulation with a small diameter and large dispersion, which contributes to improved solubility and bioavailability; (2) the efflux ratio of micelles was significantly increased in Caco-2 cell monolayers; (3) the carrier HS15, a surfactant, may increase permeability of intestinal epithelium by opening tight junctions to allow paracellular transport, or may inhibit the P-gp and/or CYP450 to increase intracellular concentration and residence time of IS (Kommuru et al., Citation2001).
In HE staining, there is no obvious difference between the two groups in the gastrointestinal safety assay after long-term administration in SD rats, which indicates that the novel DDS is safe. Therefore, this mixed micelle resolves issues of poor oral absorption of IS and has great potential for clinical use.
Conclusion
An oral drug delivery system based on Solutol®HS15 and Pluronic F127 nanoparticles was developed to markedly enhance the solubility and oral absorption of IS. IS was encapsulated in spherical micelles and demonstrated a sustained release behavior. The mixed micelles significantly enhanced the permeability of IS across the Caco-2 cell monolayer, due to the inhibition of P-gp and other pumps by HS15 and F127. In addition, the HE staining results show gastrointestinal safety. Thus, mixed micelles have great potential to improve the absorption of IS and have significant clinical value.
Declaration of interest
The authors declare no competing financial interest.
The study was financially support by National Nature Science Foundation of China (Grant Nos. 81274088, 81303275, 81503253).
References
- Arief ZM, Munshi AH, Shawl AS. (2015). Evaluation of medicinal value of Epimedium elatum on the basis of pharmacologically active constituents, Icariin and Icariside II. Pak J Pharm Sci 28:1665–9
- Chen Y, Jia XB, Hu M. (2009). Absorption and transportation of flavonoids in Herb Epimedii across Caco-2 monolayer model. Chinese Trad Herb Drug 2:220–4
- Chen Y, Jia XB, Tan XB, et al. (2009). Absorption and metabolism of flavonoids in Herb Epimedii via rat intestinal perfusion model. China J Chinese Material Med 22:2928–31
- Chen Y, Zhao YH, Jia XB, Hu M. (2008). Intestinal absorption mechanisms of prenylated flavonoids present in the heat-processed Epimedium koreanumNakai (Yin Yanghuo). Pharm Res 25:2190–9
- De JWH, Hagens WI, Krystek P, et al. (2008). Particle size-dependent organ distribution of gold nanoparticles after intravenous administration. Biomaterials 29:1912–9
- Dehghan KP, Saadat E, Ravar F, et al. (2014). Pluronic F127 polymeric micelles for co-delivery of paclitaxel and lapatinib against metastatic breast cancer: preparation, optimization and in vitro evaluation. Pharm Dev Technol 29:1–9
- Fletcher JI, Haber M, Henderson MJ, Norris MD. (2010). ABC transporters in cancer: more than just drug efflux pumps. Nat Rev Cancer 10:147–56
- Geng YD, Zhang C, Shi YM, et al. (2015). Icariside II-induced mitochondrion and lysosome mediated apoptosis is counterbalanced by an autophagic salvage response in hepatoblastoma. Cancer Lett 366:19–31
- Gradauer K, Nishiumi A, Unrinin K, et al. (2015). Interaction with mixed micelles in the intestine attenuates the permeation enhancing potential of alkyl-maltosides. Mol Pharm 12:2245–53
- Jin X, Zhang ZH, Sun E, et al. (2013). A novel drug-phospholipid complex loaded micelle for baohuoside I enhanced oral absorption: in vivo and in vivo evaluations. Drug Dev Ind Pharm 39:1421–30
- Jun J, Li J, Zhang Z, et al. (2015). Mechanism of enhanced antiosteoporosis effect of circinal-icaritin by self-assembled nanomicelles in vivo with suet oil and sodium deoxycholate. Int J Nanomedicine 10:2377–89
- Kommuru TR, Gurley B, Khan MA, Reddy IK. (2001). Self-emulsifying drug delivery systems (SEDDS) of Coenzyme Q10: formulation development and bioavailability assessment. Int J Pharm 212:233–46
- Kwon SK, Kim HB, Song JJ, et al. (2014). Vocal fold augmentation with injectable polycaprolactone microspheres/pluronic F127 hydrogel: long-term in vivo study for the treatment of glottal insufficiency. PLoS One 9:e85512
- Li C, Sun E, Zhang ZH, et al. (2012). Enhancement of Epimedium fried with suet oil based on in vivo formation of self-assembled flavonoid compound nanomicelles. Molecules 17:12984–96
- Li X, Yu Y, Ji Q, Qiu L. (2015). Targeted delivery of anticancer drugs by aptamer AS1411 mediated Pluronic F127/cyclodextrin-linked polymer composite micelles. Nanomedicine 11:175–84
- Liu Y, Zhang J. (2015). Influence of pore symmetries on the supercapacitive performance of mesoporous carbons co-templated by F127 and PDMS–PEO. Micropor Mesopor Mat 206:81–5
- Murgia S, Fadda P, Colafemmina G, et al. (2013). Characterization of the Solutol® HS15/water phase diagram and the impact of the Δ9-tetrahydrocannabinol solubilization. J Colloid Interface Sci 390:129–36
- Song J, Huang H, Xia Z, et al. (2015). TPGS/phospholipids mixed micelles for delivery of icariside II to multidrug-resistant breast cancer. Integr Cancer Ther 20:1–9
- Stef K, Tom W, Heleen VDN, et al. (2015). Self-assembly of Pluronic F127-silica spherical core − shell nanoparticles in cubic close-packed structures. Chem Mater 27:5161–9
- Wu WT, Guan ZY. (2015). Docetaxel-loaded mixed micelles composed of Solutol HS15 and Pluronic F127 or folate-conjugated F127: preparation, optimization and in vitro comparative characterization. J Chin Pharm Sci 24:95–103
- Wu WT, Shi WJ, Wu QZ, Zhou JP. (2013). Preparation and in vitro evaluation of docetaxel-loaded Solutol HS 15/Pluronic F127 mixed micelles. Pro Pharm Sci 5:222–9
- Wu J, Song T, Liu S, et al. (2015). Icariside II inhibits cell proliferation and induces cell cycle arrest through the ROS-p38-p53 signaling pathway in A375 human melanoma cells. Mol Med Rep 11:410–16
- Yan H, Song J, Zhang Z, Jia X. (2015). Optimization and anticancer activity in vitro and in vivo of baohuoside I incorporated into mixed micelles based on lecithin and Solutol HS 15. Drug Deliv 8:1–9
- Yu JN, Zhu Y, Wang L, et al. (2010). Enhancement of oral bioavailability of the poorly water-soluble drug silybin by sodium cholate/phospholipid-mixed micelles. Acta Pharmacol Sin 31:759–64
- Zhang Z, Lv H, Jia X, et al. (2012). Influence of vitamin E tocopherol polyethylene glycol succinate 1000 on intestinal absorption of icariside II. Pharmazie 67:59–62
- Zhang H, Yang X, Zhao L, et al. (2015). In vitro and in vivo study of Baicalin-loaded mixed micelles for oral delivery. Drug Deliv 25:1–7
- Zhang H, Zhao L, Chu L, et al. (2014). Preparation, optimization, characterization and cytotoxicity in vitro of Baicalin-loaded mixed micelles. J Colloid Interface Sci 434:40–7