Abstract
After the nerve selective regeneration theory was put forth [Citation], many researchers had focused on it and its possible application. According to our previous study, the results of small gap sleeve bridging peripheral nerve mutilation had been confirmed [Citation]. But the imminent causes of those results haven't been unveiled completely. This study tries to explore these reasons in some aspects. The results indicate the advantages of this new surgical method not only because of nerve selective regeneration but also because the small gap can create a suitable microenvironment for peripheral nerve regeneration.
INTRODUCTION
Over the past century, a dogma has dominated that adult mammalian peripheral nervous system (PNS) neurons can regenerate easily and completely. However, this regeneration does not always translate into successful functional outcomes following repair of human nerve injuries. The outcomes of mutilation of peripheral nerve may be the worst in peripheral nerve injury. There are three difficulties impair the repairing results: one is that the perfect in situ saturation cannot be achieved as in an animal model; even a little rotation could result in serve function descent [Citation3]; another is misconnection and paraplasia in nerve fibers, and the third is that regeneration nerve fiber cannot re-innervate the distal target organ.
The selective nerve regeneration theory of peripheral nerve was confirmed and repeated. Some researchers raised a new way, known as small gap (2-3mm) sleeve bridging in repairing peripheral nerve mutilation (). When there was a small gap between the ruptured mixed nerve, the proximal sensory and motor nerve fibers would grow through the gap and selectively find their counterparts in the distal nerve stump. This new approach showed more advantages than the suturation of epineurium with translocation in rodents and primates [Citation3,Citation4]. In our previous studies, the hollow cylindrical conduit (an absorbable de-acetyl chitin conduit invented by Beijing University and the Chinese Textile Academy, the State Patent No: 01136314.2) can construct a nerve regeneration chamber with well biocompatibility (). The small gap can create a “window” for observing the process of regeneration and detecting many factors’ expression.
Figure 2. Absorbable de-acetyl chitin conduit with 2 mm inner diameter used in this experiment (with permission of Dr. Peixun Zhang).
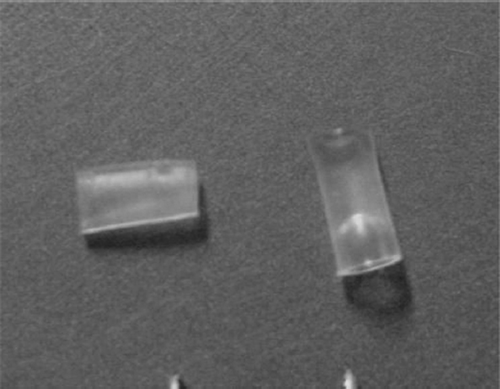
Reciprocal interactions between neurons and Schwann cells are crucial for the organization and function of the PNS, from neurogenesis in embryonic development to synaptic plasticity and nerve regeneration. Neuregulin-1 (NRG1) has emerged as the pivotal signal that controls Schwann cells at every stage of the lineage [Citation5,Citation6]. Growth associated protein 43(GAP-43) was the first neuronal growth-associated protein to be identified [Citation7]. It is concentrated in the cortical cytoskeleton of axonal growth cones, interacts with the cell membrane and actin filaments, and overexpression results in enhanced neurite outgrowth and enhanced axonal sprouting in vivo [Citation8]. Previous studies of these two factors emphasized the mechanism or relationship between NRG-1 GAP-43 and Schwann cells in vitro. Our previous results show that the small gap bridging peripheral nerve mutilation is better than epineurium neurorrhaphy with distal stumps turning [Citation2]. What are the imminent causes for these outcomes and which factors are working predominantly in this gap? Does the nerve selective regeneration theory work alone? These factors’ role and changing rules in the small gap sleeve bridging is still undefined. Resolutions of these intangible questions can provide powerful support for applying this new repairing method in clinical practice.
SURGERY
All animal protocols were approved by the Institutional Animal Care and Use Committee of The Peking University.72 Sprague Dawley adult female rats weighing 200–250g were used in this study. Animals were anesthetized in an ice-water bath. Using aseptic technique, the right sciatic nerve was exposed at the sciatic notch and transected with fine scissors. The left sciatic nerve was not lesioned and served as a control. The skin was closed with sutures, and the pups were resuscitated by warming and then returned to their cages. Two groups of experiments were carried out. Group A: epineurium neurorrhaphy with distal stumps turning 180°: epineurium neurorrhaphy was applied using 10-0 nylon suture at 4 × microscopes with 2 stitches one stump with the distal stump turning 180°. Groups B: chitin conduit small gap sleeve bridging: conduit was put between the two stumps at 4 × microscopes, 10-0 nylon suture penetrated the conduit wall from external side to inner side at the site 1mm the conduit stump fringe. After suturing the epineurium, suture needle penetrated the conduit wall from inner side to external side at the corresponding paralleling site with the needle point. There was 2 mm space between the two nerve stumps and nerve stumps were inserted into conduit about 1mm. The suture model is shown in . At various times after surgery (1d, 3d, 5d, 7d, 14d, 28d,), the animals were killed by CO2 inhalation, from the suture point, and both 5mm proximal and distal stumps were removed for immunofluorescence and real-time PCR detection.
Immunofluorescence
Forimmunofluorescence, samples were fixed in 4% paraformaldehyde for 12h, embedded in paraffin and cut perpendicular to the main nerve axis from the suture site in group A (A1: proximal stump; A2 distal stump). In group B, the sections were performed perpendicular to the main nerve axis from the suture site of both proximal (B1) and distal stumps (B2) with the tube. Sections were then incubated overnight in a solution containing the axonal marker anti-neurofilament-200kD (monoclonal, mouse, dilution 1:400, Sigma,) and the Schwann cell's marker anti-S100 (polyclonal, rabbit, dilution 1:200, Sigma). After washing in PBS, sections were incubated for 1 h in a solution containing two different secondary antibodies: FITC-conjugated swine anti-rabbit IgG (dilution 1:200, Santa Cruz) and TRITC-conjugated goat anti-mouse IgG (dilution 1:200, Santa Cruz). The sections were finally mounted with a Dako fluorescent mounting medium and analyzed by a fluorescence microscope (Olympus, BX50, Japan).
RNA isolation and cDNA Synthesis
The samples were carefully excised from each animal and frozen in liquid nitrogen immediately. For quantitative assessment of mRNA expression, three animals for each time-point were used. Total RNA was isolated using RNeasy mini kit (Qiagen, Valencia, CA). Real-time quantitative RT-PCR was performed on a 7900HT Real-Time Detection System (ABI) using QuantiTect SYBR Green RT-PCR kit (Qiagen, Valencia, CA). Expression levels of NRG-1, GAP43 in rat sciatic nerve were determined and normalized as for P-actin. Standard curves for every set of primers were performed to assess amplification efficiency and dynamic range. Each sample was measured in quadruplicate. Data were expressed as average of normalized gene expression of at least three independent experiments as described previously [Citation9]. The following forward (F) and reverse (R) primers were used for real-time quantitative RT-PCR ().
Table 1. Description of oligonucleotide primers used for real time RT-PCR analysis
Each PCR reaction consisted of 2.0 μl cDNA, appropriate primers. All reactions were performed in triplicate under identical conditions, which consisted of incubation at 95L for 10 min to denature cDNA and activate the enzyme followed by 40 cycles of amplification with denaturation at 95L for 15 s —60L 1min and finally, annealing/ elongation at 95L for 15 s —slope 60L 1min. The relative expression level of the gene of interest was computed with respect to the mRNA expression level of the internal standard, β-actin, using the formula: Relative mRNA
where Ct is the threshold cycle value [Citation10].
Statistical Analysis
Data are expressed as mean ±SD and were evaluated by Student's t test or by one-way ANOVA followed by post hoc tests depending on the experimental design. Differences among groups were considered significant if p <0.05.
RESULTS
3.1 General Observations
None of the rats died due to surgically related or other causes before sacrifice. With respect to locomotor function, slight limping caused by unilateral foot drop was observed for up to 4 weeks after surgery. At each point after implantation, the chitin conduit in group B remained in its initial shape, while the suture sites in group A were swollen. The capillaries and granulation tissue could be found on the surface of the conduit. shows the well biocompatibility of this conduit.
3.2 Schwann Cells and Nerve Fibers Counting
The Schwann cells and nerve fibers counting at each time point are shown in and . In Group A the proximal stumps and distal stumps were defined A1 and A2; in Group B the two stumps were B1 and B2.
Table 2. Schwann cells counting per unit vision field
Table 3. Nerve fibers counting per unit vision field
The proximal stumps’ Schwann cell changes in two groups were similar (). The Schwann cells decreased heavily in 1 to 3 days after injury and the recovery tendency occurred at the time point of 5d and 14d after injury and last to 28d. There were no significant statistical differences between group A and group B at the time point of 1d, 3d and 5d (P≥0.05). There were significant statistical differences between group A and group B at the time point of 7d, 14d and 28d (P<0.05).
The distal stumps’ Schwann cell changes in two groups were dissimilar (). In group A the Schwann cells decreased heavily in 1 to 7 days after injury and the recovery tendency occurred at the time point of 5d and 14d after injury and last to 28d. In group B the Schwann cells also decreased heavily in 1 to 3 days but recovery tendency occurred at the time point of 5d after injury and last to 28d. There were no significant statistical differences between group A and group B at the time point of 1d, 3d and 5d (P≥0.05). There were significant statistical differences between group A and group B at the time point of 7d, 14d and 28d (P<0.05).
The proximal stumps’ nerve fiber changes in two groups were similar (). The fibers that decreased heavily in 1 to 7 days after injury and the recovery tendency occurred at the time point of 14d after injury and last to 28d. There were no significant statistical differences between group A and group B at the time point of 1d and 28d (P≥0.05). There were significant statistical differences between group A and group B at the time point of 3d, 5d, 7d and 14d (P<0.05).
The distal stumps’ nerve fiber changes in two groups were similar with proximal (). The fibers number decreased heavily in 1 to 7 days after injury and the recovery tendency occurred at the time point of 14d after injury and last to 28d. There were no significant statistical differences between group A and group B at the time point of 1d, 3d and 7d (P≥0.05). There were significant statistical differences between group A and group B at the time point of 5d, 14d and 28d (P<0.05).
3.4 NRG-1 GAP-43 mRNA Expression Analysis
Real-time quantitative RT-PCR analyses for NRG-1 and Gap43 were performed from cDNA using the TaqMan technology as described earlier. mRNA expression was quantified relative to the expression of the b-actin, whose expression remained constant for all conditions tested.
shows variations in the mRNA expression level of NRG-1 from 1d to 28d after mutilation of sciatic nerves. NRG-1 represents the increase in two groups at the time point of 1d after injury and last to 28d. There was a peak on 14d in two groups. There were no significant statistical differences between group A and group B at the time point of 1d, 3d, 5d and 7d (P≥0.05). There were significant statistical differences between group A and group B at the time point of 14d and 28d (P<0.05). There were significant statistical differences between normal sciatic nerves and two groups in each time point (P<0.05).
shows variations in the mRNA expression level of GAP-43 from 1d to 28d after mutilation of sciatic nerves. GAP-43 represents the increase in two groups at the time point of 1d after injury and last to 28d. There was a peak on 7d and 14d in two groups. There were no significant statistical differences between group A and group B at the time point of 1d, 3d, and 28d (P≥0.05). There were significant statistical differences between group A and group B at the time point of 5d, 7d and 14d (P<0.05). There were significant statistical differences between normal sciatic nerves and two groups in each time point (P<0.05).
DISCUSSION
In this study, we have shown that the Schwann cells and nerve fibers living condition and activated condition in small gap sleeve bridging group were better than that in the control group. The conduit and two stumps of mutilation nerve create a relatively closed chamber in the early period after injury (). This protective chamber can reduce the death of Schwann calls and nerve fiber by high concentration of NRG-1 and GAP-43. These results provide the powerful mechanical support for this new nerve repairing method.
Figure 11. 7 days after small gap sleeve bridging. The conduit and the two stumps create a relative airtight lacuna.
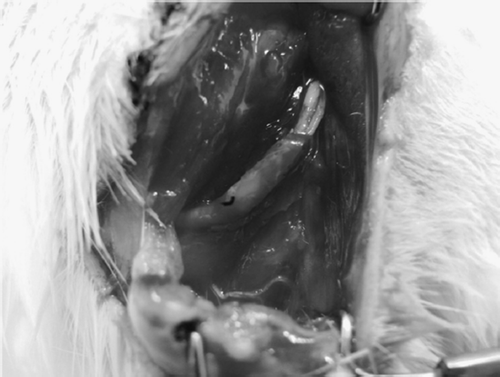
The outcomes of peripheral nerve mutilation are often unsatisfied. Even an experienced surgeon can hardly suture the two stumps in suit perfectly as in animal models. A main reason for phantom limb pain and limbs paresthesia is misconnection and paraplasia in regeneration nerve fibers. Only 10°rotation in two stumps can induce heavy dysfunction [Citation3]. In actuality, the rotation degree cannot be obtained in patients with peripheral nerve mutilation after surgery. So we create an animal model with which the distal stumps turn 180° as the control of small gap sleeve bridging. In this study we have sought to answer the question of whether the nerve regeneration procedures in these two different repairing methods are parallel. By comparing the Schwann cells and nerve fibers number and two factors mRNA expression level, we found that the nerve regeneration procedures in these two methods are dissimilar in adult rats. We also show that the nerve regeneration in our absorbable de-acetyl chitin conduit is better than that with distal stump turning suture.
With the nerve selective regeneration theory put forth by Cajal et al. [Citation1] and widely accepted, we performed serial experiments and provided powerful evidence for the effectiveness of the small gap sleeve bridging peripheral mutilation in rodent and monkey [Citation2,Citation4]. Indeed, in the last two decades many scholars have witnessed a renewed interest in tubulization research. Lots of materials were used, such as autogenous venous, arteries and other bio-materials. But there were some defects in these materials. The autogenous materials often collapsed in 7d-14d after repairs and other compound conduits possibly affected the injury site remodel in 2-3 mouths because these materials could not be absorbed. Our de-acetyl chitin conduit, which can retain the conduit structure for at least 6 weeks in vivo, can biodegrade completely. Furthermore, the deacetyl chitin conduit's microenvironment can be easily manipulated and the regenerative response monitored, thereby providing the investigator with a window into the biochemical and cellular events of peripheral nerve regeneration.
Some researchers deem that the injury response and regeneration start parallel within 24h. But the histological changes are Wallerian degenerations predominantly in this period. In this study, within 1-3 days, the Schwann cells in both proximal and distal stumps reduced dramatically in two groups. It is possible that the inflammatory response after injury evokes the Schwann cell apoptosis in both stumps [Citation11]. The possible reason for Schwann cells proliferation peak in 5 days at the proximal stump can be attributed to macrophage invasion; it might be triggered by some diffusible factors released from the nerve undergoing Wallerian degeneration. In two groups, the Schwann cells in proximal cease to proliferate while extending slender cytoplasmic processes, which form the cellular strands called the Schwann cell column in 7 days [Citation12]. In distal stumps in two groups, Schwann cells decrease not only because of cell apoptosis but they also lose the support of the neural innervations. There were significant statistical differences between group A and group B in both proximal and distal stumps Schwann cells counting at the time point of 7d, 14d and 28d (P<0.05). Because in group B the conduit can create a relatively airtight lacuna () that restrain the beneficial factors in the small gap and maintain an effective concentration without running off as in group A, these factors can sustain the Schwann cells’ survival and proliferation.
Seen from the results of this study, the changing tendency of the nerve fiber numbers was similar. There were significant statistical differences between group A and group B in proximal nerve fibers counting at the time point of 3d, 5d, 7d and 14d (P<0.05) and in distal nerve fibers counting at the time point of 5d, 14d and 28d (P<0.05). From the observation it was suggested that in group B there were more regeneration nerve fibers from proximal stumps across the conduit to the distal stumps, which spouting from the proximal stumps achieve effective regeneration. The conduit can guide the regenerative nerve fibers to reach the distal regularly (unpublished).
Our data clearly shows that the NRG-1 and GAP-43 were expressed in a higher level than the threshold levels in normal sciatic nerve. NRG1 has a crucial role at essentially every developmental stage of Schwann cells [Citation13,Citation14]. These functions include promoting the gliogenic fate of trunk neural crest cells, the migration of Schwann cell precursors (SCP) along axons, and their subsequent proliferation and survival induced by axons. NRG-1 has also been considered a candidate to mediate the Schwann cell response to injury as it is persistently expressed by adult axons and could therefore be released during Wallerian degeneration to stimulate Schwann cell proliferation [Citation15]. Additional NRG-1 can promote the Schwann cells myelinization in vitro. In our study, the expression peak of NRG-1 occurred at the time point of 14d and 28d, and there were significant statistical differences between group A and group B at the time point of 14d and 28d (P<0.05). This result can explain the Schwann cells’ number difference in two groups in some aspect. In conduit in group B, the high concentration of NRG-1 can maintain the survival and proliferation more intensely than that in group A [Citation16].
GAP-43 was the first neuronal growth-associated protein to be identified [Citation7]. The current consensus view is that GAP-43 is involved in axonal sprouting and in the response of growth cones to guidance cues. Some researchers have shown that overexpression of GAP-43 in dorsal root ganglion (DRG) neurons greatly enhances their ability to regenerate their central axons into peripheral nerve grafts [Citation17]. Our study showed that the expression level increased conspicuously after injury in 1 to 28 days and there were significant statistical differences between group A and group B at the time point of 5d, 7d and 14d (P<0.05). It showed that the nerve regenerated procedures start in 1d after injury even within 12h, but the marked regeneration occurred in 5d after repairing and last to 28 days vividly. At 28 days after injury, there were no significant statistical differences between group A and group B; it showed that the activated regeneration area had passed the injury site and the expression level inclined to the threshold level gradually.
In summary, we have demonstrated that repairing the peripheral nerve mutilation by small gap sleeve bridging technique can obtain a better microenvironment for Schwann cells and nerve fibers living and regenerated than by suturing two stumps directly with distal rotation. The overexpression of NRG-1 and GAP-43 in small gaps should be intrinsic factors for the protective effects of this small gap created by conduit. Our recent study showed that the axon regenerated method was different with epineurium neurorrhaphy (unpublished). There must be other factors and mechanisms working in this gap. Elucidating these questions promises to provide important insights into the application of this new method in clinical practice.
Declaration of interest: The authors report no conflicts of interest. The authors alone are responsible for the content and writing of the paper.
This paper was first published online as an Early Online article on 10 November 2009.
REFERENCES
- Cajal, R.S. (1928). Degeneration and Regeneration of Nervous System, Oxford University Press, New York.
- Baoguo, Jiang, Peixun, Zhang, Dianying, Zhang, Zhongguo, Fu, Xiaofeng, Yin, Hongbo, Zhang. (2006). Study on small gap sleeve bridging peripheral nerve injury. Artificial Cells, Blood Substitutes, and Biotechnology. 34:55–74.
- Baoguo, Jiang, Shunhua, Wang, Chuanhan, Feng. (1994). The comparison of small gap autogenic artery and epineruium anastomosis bridging peripheral nerve. Journal of Beijing Medical University. 26:249–250.
- Peixun, Zhang, Xiaofeng, Yin, Yuhui, Kou, Yanhua, Wang, Hongbo, Zhang, Baoguo, Jiang. (2008). The electrophysiology analysis of biological conduit sleeve bridging rhesus monkey median nerve injury with small gap. Artificial Cells, Blood Substitutes, and Biotechnology. 36:1–7.
- Griffiths, I., Klugmann, M., Anderson, T., Yool, D., Thomson, C., Schwab, M.H., Schneider, A., Zimmermann, F., McCulloch, M., Nadon, N.. (1998). Axonal swellings and degeneration in mice lacking the major proteolipid of myelin. Science. 280:1610–1613.
- Lappe-Siefke, C., Goebbels, S., Gravel, M., Nicksch, E., Lee, J., Braun, P.E., Griffiths, I.R., Nave, K.A.. (2003). Disruption of Cnp1 uncouples oligodendroglial functions in axonal support and myelination. Nat Genet. 33:366–374.
- Pate Skene, J.H.. (1981). Annual Review of Neuroscience. 12:127–156.
- Zhang, Y., Bo, X., Schoepfer, R., Holtmaat, A., Verhaagen, J., Emson, P. C., Lieberman, A. R., Anderson, P. N.. (2005). Proc Natl Acad Sci. 102(41):14883–14888.
- Liu, Weihong, Saint, David A. (2002). A new quantitative method of real time reverse transcription polymerase chain reaction assay based on simulation of polymerase chain reaction kinetics. Analytical Biochemistry. 302:52–59.
- Heid, C.A., Stevens, J., Livak, K.J., Williams, P.M.. (1996). Real time quantitative PCR, Genome Res. 6:986–994.
- Boyle, K., Azari, M.F., Profyris, C. Petratos, S.. (2005). Molecular mechanisms in Schwann cell survival and death during peripheral nerve development, injury and disease. Neurotoxicity Research. 7(1–2):151–167.
- Yang, David P., Zhang, Dan P., Mak, Kimberley S., Bonder, Daniel E., Pomeroy, Scott L., Kima, Haesun A.. (2008). Schwann cell proliferation during Wallerian degeneration is not necessary for regeneration and remyelination of the peripheral nerves: Axon-dependent removal of newly generated Schwann cells by apoptosis. Mol. Cell. Neurosci. 38:80–88.
- Garratt, A.N., Britsch, S., Birchmeier, C.. (2000). Neuregulin, a factor with many functions in the life of a Schwann cell. Bioessays. 22:987–996.
- Jessen, K.R., Mirsky, R.. (2005). The origin and development of glial cells in peripheral nerves. Nat Rev Neurosci. 6:671–682.
- Hoke, A., Ho, T., Crawford, T.O., LeBel, C., Hilt, D., Griffin, J.W.. (2003). Glial cell line-derived neurotrophic factor alters axon Schwann cell units and promotes myelination in unmyelinated nerve fibers. J Neurosci. 23:561–567.
- Chen, S., Velardez, M.O., Warot, X., Yu, Z. X., Miller, S. J., Cros, D., Corfas, G. (2006). Neuregulin 1-erbB signaling is necessary for normal myelination and sensory function. J Neurosci. 26(12):3079–86.
- Joost Verhaagen, A., Catharina, E. E. M., Van der Zee, Henk B., Nielander, Jan P., Vos, Sofia Lopes da Silva. (1989). Expression of growth-associated protein B-50 (GAP43) in dorsal root ganglia and sciatic nerve during regenerative sprouting. The Journal of Neuroscience. 10:3505–3512.