Abstract:
In addition to heme-irons, reactive (β-globin thiols (βCys93s) of hemoglobin (Hb) also have been shown to interact with endogenous nitric oxide (NO) thereby contributing to vascular tone regulation. What relative roles do these NO binding sites contribute to the overall Hb-mediated vasoactivity? Several test Hbs with either or both the NO binding sites preliganded or blocked were prepared and tested in a rat thoracic aortic ring model. Hbs tested were: NEM-Hb (ferrous Hb with masked thiols), HbNO (ferrous Hb preliganded with NO), Hb+CN (ferric Hb liganded with CN−), NEM-HbNO and NEM-Hb+CN (Hbs with both heme-iron and cysteine sites preliganded or blocked). Typically, >0.2 μM control Hb significantly increased isometric tension in agonist stimulated vessel rings (58.1 ±7.0% over baseline). At comparable concentrations, NEM-Hb also caused a significant contraction (50.7±9.5%) while HbNO and Hb+CN did not (−5.5±6.0% and −3.7±4.6%, respectively). For these Hbs, masking thiols as well did not significantly alter respective vascular effects. Ferrous sperm whale myoglobin (Mb), which has no reactive thiol, elicited a significant contraction (55.1±13.2%) while metMb did not (−0.8±3.2%), suggesting the relative importance of heme-iron ligand and oxidation state in Hb vasoactivity. Additionally, ferrous or ferric equine heart cytochrome-C, a heme protein with no readily available heme-iron and cysteine binding sites, did not elicit notable contraction. Human Hb variants in which (βCys93s are deleted or substituted with non-cysteine residues did not reveal any documented significant hemodynamic abnormalities. These results indicate that reactive globin-thiols do not appear to play a prominent role relative to heme-irons in Hb-mediated vasoconstriction.
INTRODUCTION
Currently, hemoglobin-based oxygen carriers (HBOCs) are being evaluated in preclinical and clinical studies as potential red blood cell substitutes (‘blood substitutes’) for emergency treatment of acute anemia due to traumatic or surgical hemorrhage and other conditions [Citation1, Citation2]. HBOCs are generally products derived from natural, recombinant human or animal hemoglobins (Hb). Advantages of HBOCs include the ability to carry and deliver life-sustaining oxygen, availability, an extended shelf-life and universal compatibility. However, most current acellular HBOC products elicit varying degrees of vasoconstriction and blood pressure elevation in preclinical animal studies and human clinical trials [Citation3–6]. The “hypertensive” effect of HBOCs appears to stem from an inherent vasoconstrictive property of acelluar Hb, which some believe is responsible for many of the observed adverse events noted in clinical trials. The HBOC-mediated vasoactivity issue must be fully addressed to win regulatory approval of these products [Citation3, Citation7].
Human and bovine hemoglobin in solution free of erythrocyte membrane are well known to elicit vascular contraction [Citation8–10]. A currently prevailing theory for the mechanism of acellular Hb mediated vasoactivity is heme-iron scavenging (inactivation) of endogenous nitric oxide (NO), a potent smooth muscle relaxation factor constitutively synthesized and released by the vascular endothelium to regulate normal vascular tone [Citation8, Citation11].
Recently, reactive globin-thiols of Hb have been proposed to play a key role in regulating vascular tone through reversible nitrosation reactions with endogenous NO [Citation12]. In this theory, βCys93 thiols serve as either NO scavengers or donors depending on the local oxygen tension. In the pulmonary venous circulation where PO2 is high, Hb is oxygenated (HbO2) taking the R-conformational state. In this state, Hb βCys93 thiol's reactivity for NO is reported to be high allowing NO to be “loaded” onto the Hb-thiols by forming S-nitrosothiol (SNO-Hb). In the blood vessels of actively metabolizing tissues where O2 is consumed (thus lower PO2), the conformational structure of Hb is switched to T-state as it is being deoxygenated. In the T-conformation, Hb βCys93 thiols’ affinity for NO is claimed to be lower, allowing NO to be released to dilate blood vessels, thus increasing blood flow and oxygen supply to the affected tissues [Citation12–14]. This is an intriguing theory since it assigns Hb a new function as an allosterically controlled blood flow regulator. Interestingly, thiol containing agents such as L-cysteine and dithiothreitol were shown to contract isolated rabbit aorta [Citation15].
In this study, we investigated whether βCys93 thiols play a significant role in acellular Hb mediated vascular contraction using an isolated rat thoracic aorta model as a functional assay. To better define the roles of globin-thiols and heme-iron, Hbs modified at the heme-iron and/or βCys93 sites were prepared and their vasoactivities tested. In addition, vasoactivities of natural heme proteins with heme and/or cysteine sites not readily available for NO reaction were also examined. Finally, a database for naturally occurring Hb variants was searched for Hb variants with βCys93 deleted or substituted with other residues or Hb variants with non-cysteine residues were substituted with cysteine. They were reviewed for any documented blood pressure abnormalities and other cardiovascular pathophysiology.
MATERIALS AND METHODS
Preparation of Test Hbs
Highly purified human HbA0 (obtained from Hemosol, Inc, Toronto, Canada) was used in preparing test Hbs. Utilizing heme-iron and globin-thiol site specific reaction chemistries, several test Hbs with either heme-iron sites or βCys93 sites or both of the sites modified were prepared (see below). In addition, sperm whale myoglobin (Mb) and equine heart cytochrome-C were purchased from Sigma Chemical Co. (St. Louis, MO). Because commercial heme products were supplied in mostly oxidized form, they were reduced to ferrous form as necessary by using sodium dithionite. Reduction was confirmed by monitoring absorption spectra with a spectrophotometer. Unless otherwise noted, all other chemicals/reagents were purchased from Sigma Chemical Co.
Thiol-masked Hb (NEM-Hb). A human Hb masked at βCys93 was prepared by modification of a previously described method [Citation16, Citation17]. Hb was reacted with excess N-ethyl maleimide (NEM; a cysteine specific masking reagent) ([NEM]/[Hb] = 10) in 100 mM phosphate buffer, pH 7.0, at room temperature for 2 hours. To confirm complete masking of the (βCys93 thiols, an aliquot of the reaction product was titrated with p-chloromercuribenzoate while monitoring changes in absorption at 235–255 nm using a UV/VIS spectrophotometer (HP-8452, Palo Alto, CA) [Citation18, Citation19].
Heme-iron preliganded with NO or CN− (HbNO, Hb+CN). To prepare nitrosyl Hb (HbNO), deoxygenated Hb (0.1 mM) was reacted with 1 mM sodium nitrite at room temperature for 2 hours. CyanometHb (Hb+CN) was produced using a commercial reagent kit (DSA, San Antonio, Texas) based on potassium ferricyanide and potassium cyanide to convert Hb to stable cyanometHb.
Both heme-iron liganded and globin-thiol masked Hbs (NEM-HbNO, NEM-Hb+CN). NEM-HbNO was similarly produced using deoxygenated NEM-Hb. NEM-Hb+CN was produced by reacting oxy NEM-Hb with cyanometHb reagent at room temperature.
Final test Hb preparations were dialyzed against at least 100x volumes of phosphate buffer overnight at 4°C and concentrations adjusted as necessary. Absorption spectra of test Hbs were examined using a UV/VIS spectrophotometer to confirm formation of desired Hb products.
Experimental Protocols
Isometric vascular tension measurements. The isolated rat thoracic aortic ring model has been described previously [Citation10]. The animal protocol was approved by the Institutional Animal Care and Use Committee. Briefly, male Sprague Dawley rats of 250–350 g body weight were anesthetized with methoxy flurane. Through a midline incision, the thoracic aorta was carefully excised avoiding endothelial damage. Following clearing of connective and other non-vascular tissues, the vessels were cut transversely into 2–3 mm ring segments and placed in a tissue bath for isometric tension measurements. The tissue bath pre- filled with a Krebs buffer (pH=7.4) was maintained at 37° C. The buffer was continuously aerated with a 95% O2-5% CO2 gas mixture. The vessel rings were initially set at imposed tension of 1.5–2 grams and allowed to relax for 1 hour. The responsiveness of vessel rings was first assessed by treating with 50 nM norepinephrine (NE); vessel rings that developed less than 0.5 g of additional tension (contraction) from the baseline were considered nonviable and excluded. Functional integrity of the endothelium was, then, assessed by treating the vessels with 10–50 μM acetylcholine (Ach). If vessel rings showed relaxation less than 10% from the NE-produced tension, functional endothelium was considered absent and excluded from further testing. After the equilibration and viability testing, vessel rings were treated with test Hbs and the maximal changes in developed tension were assessed.
Survey of human Hb variants at βCys93. Human Hb variants databases (Globin Gene Server, Pennsylvania State University; http://globin.cse.psu.edu/) were searched for Hbs with cysteine (Cys) residues substituted with other amino acids. If those Cys variants, reports of any documented abnormalities of Hb function (O2 affinity, Bohr effect, stability, etc.), BP and other cardiovascular physiology in the afflicted individuals were searched using Pub Med literature search.
Data representation and analysis. Data are presented as standard errors of means (SE). Statistical significance was determined by Student's paired (before and after treatment comparisons) or unpaired t-tests (between group comparisons) at p=0.05 level.
RESULTS
Vascular Responses to Hb with Heme and/or Cysteine Modifications
In NE tone-enhanced endothelium-intact aortic vessel rings, treatment with control ferrous Hb (HbO2) elicited a significant contraction; 0.4μM Hb caused 58.1 ±7.0% increase in vessel ring tension over the pretreatment tension value (P<0.01, N=8). Ferrous oxyHb with (βCys93 masked with NEM (NEM-Hb, 0.4μM) also caused a significant contraction (50.7±9.5%, P<0.01, N=8) comparable to that elicited by HbO2 (). In contrast, cyanometHb (Hb+CN), a Hb whose ferric hemes are liganded with CN-, did not produce a notable contraction (−3.7±4.6%, N=7, P>0.05) (, ). For an unknown reason, vessel rings in this series produced smaller than usual but still significant contractions following HbO2 treatments relative to pretreatment values or those elicited by HbCN. Masking both the heme-iron and cysteine residues (NEM-Hb+CN) had no further effect (12.0±4.5, N=7, P>0.05) (, ). When deoxyHb was preliganded with NO (HbNO), it did not elicit any significant contraction (−5.5±6.0%, N=7, P>0.05) (). Similarly, masking cysteines of HbNO (NEM-HbNO) did not alter its non-vasoactivity (3.2± 1.7%, N=3, P>0.05).
Vascular Responses to Heme Proteins with no Reactive Cysteines or Hemes
Human Mb contains one cysteine (E110) while sperm whale Mb has none [Citation20–21]. Ferrous sperm whale myoglobin (2 μM) elicited a significant contraction (55.1±13.2%, N=10, P<0.01) while ferric Mb (met-Mb) at comparable concentration did not (−0.8±3.1%, N=6, P>0.05) (). Finally, equine cytochrome C (Cyt-C) has two cysteine residues per molecules but they are crosslinked to the propionic groups of the heme, making them unreactive. In addition, the 6th coordinate of the heme-iron is covalently linked to methionine of the polypeptide chain, leaving no ready binding site for NO. Neither the 2 μM ferrous cytochrome-C nor 2 μM ferric cytochrome-C elicited a notable contraction at all (−6.8±4.3% and −3±3.2%, respectively, N=10 each, P>0.05) ().
Figure 1. Vascular responses to native and cysteines masked Hbs. a) In rat thoracic aortic rings precontracted with 100 nM NE, treatment with 33 μM Ach produced a signifcant relaxation, indicating presence of intact functional endothelium. In these vessel rings, subsequent treatment with 0.4 μM oxyhemoglobin (Fe+2) elicited a signifcant contraction. A representative tracing is shown. b) In similarly treated rat aortic rings, treatment with 0.4 μM NEM-HbO2 (oxyhemoglobin with (βCys93 masked by NEM treatment) also caused a signifcant contraction. c) In these vessel rings, both HbO2 and NEM-HbO2 produced comparable contractions (58.1 ±7.0% and 50.7±9.5% of baseline values, respectively). N=7-8 vessels each.
**significantly different from respective pretreatment value (P<0.01)
NS: not signifcantly different between the two groups (P>0.05)
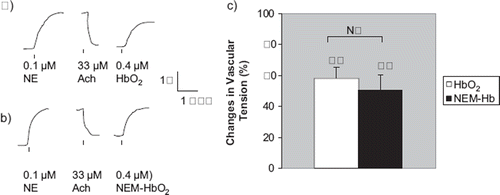
Figure 2. Vascular responses to Hb+CN, an Hb with ferric heme-irons, and NEM- Hb+CN, an Hb with both ferric heme-irons and masked (βCys93 cysteines. a) In rat thoracic aortic rings precontracted with 100 nM NE, treatment with 33 μM Ach produced signifcant relaxation indicating presence of intact functional endothelium. In these vessel rings, treatment with 0.4 μM Hb+CN did not cause any signifcant contraction. But subsequent treatment with 0.4 μM HbO2 (Fe+2) elicited a signifcant contraction. A representative tracing is shown. b) In similarly treated rat aortic rings, treatment with 0.4 μM NEM- Hb+CN did not cause a signifcant contraction, either. But subsequent treatment with 0.4 μM HbO2 again elicited a signifcant contraction. c) and d) In these vessel rings, neither Hb+CN nor NEM- Hb+CN produced signifcant contractions (− 3.7±4.6% and 12.0±4.5% of baseline values, respectively). N=7 vessels each.
**signifcantly different from respective pretreatment value (P<0.01)
NS: not signifcantly different from respective pretreatment value (P>0.05)
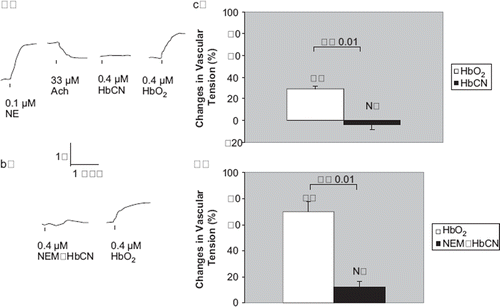
Survey of Naturally Occurring Hb Variants of Cysteine Residues
Search of a database on known human Hb variants revealed 4 naturally occurring Hb variants with cysteine residues substituted with other amino acid(s) (Globin Gene Server, PSU). It appears that Hb variants exist for all three cysteine locations (α104, β93, and β112): Hb Sallanches (α104Cys->Tyr), Hb Okazaki (β93Cys->Arg), Hb Yahata (β112Cys->Tyr) and Hb Cleveland (β93Cys->Arg and βGlu121-> Gln) [Citation46–49]. Most of these Hb variants were reported to exhibit increased oxygen affinity, decreased Bohr effect, and reduced stability, especially in homozygous individuals. However, reports of serious abnormal blood pressure, vasoactivity and other hemodynamic abnormalities in afflicted individuals have not been found.
DISCUSSION
The primary objective of this study was to assess the relative roles of heme-irons and reactive globin-thiols (two β93Cys) in the acellular Hb mediated vasoconstriction.
Currently, the most plausible mechanism proposed for the Hb-mediated vascular contraction is Hb inactivation of endothelium derived NO, a potent endogenous relaxation factor, based on the fact that NO has a high affinity for ferrous Hbs [Citation8, Citation11]. Recently, the intriguing proposition that reversible binding of endothelial NO to reactive thiols of β-globin chains of Hb (βCys93) is a fundamental regulatory mechanism for vascular tone and control of blood flow [Citation12]. This theory postulates preferential NO binding to βCys93 thiols for Hb in the R-conformational state as Hb is oxygenated in the high PO2 pulmonary vessels, forming S-nitrosated oxyHb (SNO-HbO2). The NO from SNO-Hb is then released as Hb is desaturated, a reversion to the T-conformational state, in the blood vessels of the low PO2 or hypoxic tissues. Thereby, Hb is proposed to play a new role as a NO scavenger-carrier to regulate blood flow. According to this theory, endothelial NO binds to the heme-irons of deoxyHb (T-conformational state) to form iron-nitrosyl Hb (HbNO). As the iron-nitrosyl Hb is oxygenated in the lungs, T to R transition occurs allowing some of the heme-bound NO to be transferred intramolecularly to the β globin-thiols (βCys93) to form S-nitroso Hb (SNO-Hb). Upon redeoxygenation in the tissues, some of these thiol-bound NO (e.g., SNO-) is released to the vascular smooth muscle to effect vasodilation [Citation14, Citation22–24]. The intramolecular NO transfer (intramolecular transnitrosation) from heme to globin-thiol is postulated to be allosterically controlled by PO2 under the normal physiologic condition [Citation12, Citation25, Citation26].
Figure 3. Vascular responses to HbNO, an Hb with nitrosylated heme-irons, and NEM-HbNO, an Hb with both nitrosylated heme-irons and masked (βCys93 cysteines. a) In rat thoracic aortic rings precontracted with 100 nM NE, treatment with 33 μM Ach produced signifcant relaxation, indicating presence of intact functional endothelium (tracings not shown). In these vessel rings, treatment with 2 μM HbNO did not cause any signifcant contraction. But subsequent treatment with 0.4 μM HbO2 elicited a signifcant contraction. A representative tracing is shown. b) In similarly treated rat aortic rings, treatment with 0.4 μM NEM-HbNO, Hb with both nitrosylated hem-iron and masked cysteines, did not cause a signifcant contraction, either. But, again, subsequent treatment with 0.4 μM HbO2 elicited a signifcant contraction. A representative tracing is shown. c) and d) In these vessel rings, both HbNO and NEM-HbNO produced no signifcant contractions (—5.5 ±6.0% and 3.2± 1.7% of baseline values, respectively). N=7 and 3 vessels each, respectively.
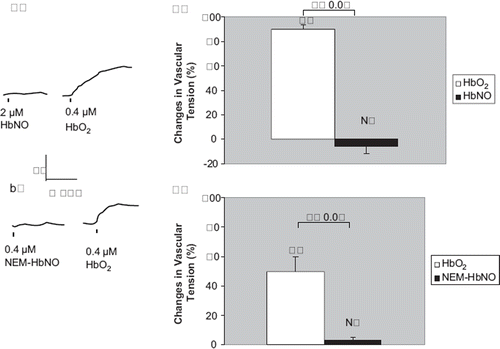
Figure 4. Vascular responses to ferrous (Fe+2) and ferric (Fe+3) sperm whale myoglobins. a) In rat thoracic aortic rings precontracted with 50 nM NE, treatment with 2 μM ferric sperm whale myoglobin (metMb) did not cause any signifcant contraction. But subsequent treatment with 2 μM oxymyoglobin (MbO2) elicited a signifcant contraction. A representative tracing is shown. b) In these vessel rings, 2 μM MbO2 and metMb produced vessel tensions of 55 ±13.2% and —0.8±3.1% of baseline values, respectively). N=10 and 6 vessels each, respectively.
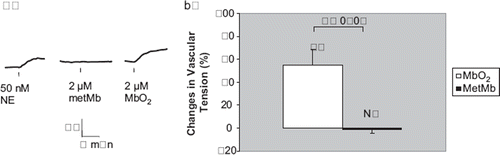
This SNO-Hb-mediated blood flow regulation hypothesis is highly controversial as some aspects of this theory have not been fully validated [Citation27–29]. High affinity of NO toward oxy and deoxy Hbs has long been established (k=2–3×107/M/sec) [Citation30]. If the higher affinity heme-iron sites are available for binding, how could NO preferentially interact with the much lower affinity βCys93 thiols? Affinity of NO toward the thiol is believed to be much lower than that toward the ferrous heme (kheme>>kthiol) as minimal nitrosation of human serum albumin was shown to occur in-vivo; NO reaction with thiol is complex as it appears to involve NO oxidation to N2O3 [Citation31]. Recently increasing data arguing against the “SNO-Hb blood flow regulation theory” have been reported [Citation32–34]. For example, in an in-vitro study of hemoglobin subjected to repeated oxygenation and deoxygenation cycle, O2 dependent allosterically controlled intramolecular transnitrosation was not observed [Citation32]. For the theory to hold, a measurable arterio-venous gradient of SNO-Hb is required; there were no significant differences in the SNO-Hb levels in the arterial and venous blood [Citation34]. In addition, the theory also claims that NO preferentially binds to β-chain hemes and thiols for the transnitration reaction. In contrast, approximately equal amounts of α- and β-nitrosylated Hbs were detected [Citation32] confirming an earlier report that NO has equivalent association rates for both α and β subunits [Citation35].
Figure 5. Vascular responses to ferrous (Fe+2) and ferric (Fe+3) equine heart cytochrome-C. a) In rat thoracic aortic rings precontracted with 50 nM NE, treatment with 2 μM equine heart ferrous or ferric cytochrome C (Cyt-C) did not cause any signifcant contraction. But subsequent treatment with 2 μM oxyhemoglobin (Hb O2) or oxymyoglobin (MbO2) elicited a signifcant contraction (tracings not shown). b) In these vessel rings, neither 2 μM ferrous Cyt-C nor 2 μM ferric Cyt-C produced signifcant contractions (− 6.8 ±4.3% and — 3.0±3.2% of baseline values, respectively). N = 10 and 6 vessels each, respectively.
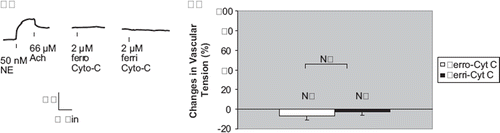
The ‘SNO-Hb mediated hypoxic vasodilation’ theory also appears to be in conflict with a well known hypoxic pulmonary vasoconstriction phenomena. In addition, in animal studies [Citation36–39] and clinical trials [Citation5, Citation40], intravenous administration of acellular HBOC elicited increases in pulmonary arterial pressure and vascular resistance. Under such conditions, the “SNO-Hb” theory predicts NO release from the βCys93 thiols will promote vasodilation since, in the pulmonary artery, Hb is predominantly in the deoxygenated state. In this state, the βCys93 thiols are claimed to have lower NO affinity [Citation12–14].
In support of the “SNO-Hb theory,” a mechanism has been proposed that SNO-Hb formation occurs via glutathione as an intermediary. Glutathione is present in high concentration in the red blood cells (mM range); it may play an intermediary role by forming S-nitroso glutathione (GSNO), which transnitrosates the Hb-βCys93 to form SNO-Hb [Citation12]. However, when Hb or Mb was incubated with GSNO, predominantly iron nitrosylated products (HbNO and MbNO) were detected [Citation41] suggesting NO is primarily transferred from GSNO to the heme-iron moieties rather than globin-thiols. These results are inconsistent with the notion that GSNO transfers NO only to other reactive thiols (e.g., βCys93) through transnitrosation to produce S-nitroso compounds such as SNO-Hb. In addition, detection of predominantly iron nitrosylated Hb in human subjects breathing NO indicates that absorbed NO predominantly interacted with heme moieties of Hb [Citation42].
In the present study, because the tissue bath was oxygenated with a gas mixture enriched with O2, the predominant conformational state of the test Hbs would be the R-state (oxyHb). Therefore, if the “SNO-Hb theory” is valid, the vessel rings would have been exposed to a substantial amount of the SNO-Hb that formed. In preliminary experiments, the vessel rings failed to relax when subjected to lower PO2 conditions by either turning off the O2 supply for an extended period or switching to 95%N2-5%CO2 gas. For this hypothesis to be accepted, the vessel rings should have relaxed upon removal of oxygen supply since NO should have been released from SNO-Hb formed under the high oxygen condition.
In addition, ferrous Hb with β-globin thiols masked by thiol specific reagent NEM (NEM-Hb) did not significantly attenuate the vascular contractile potency of unmodified control Hb and Hb preparations with either ferrous heme-iron preliganded with NO (HbNO, NEM-HbNO) or ferric heme (Hb+CN, NEM-Hb+CN) did not elicit a significant contraction. These observations in the isolated rat aorta model clearly indicate that globin thiols are not key players in Hb mediated vasoactriction. Further, ferrous sperm whale myoglobin (Mb), which has no reactive thiols, elicited contraction (and did not relax upon deoxygenation) but ferrous or ferric cytochrome-C did not. In equine heart cytochrome-C, the fifth and the sixth coordinates of heme-iron are covalently bonded to imidazole nitrogen of histidine and sulfur of methionine, respectively, making it relatively unreactive to NO in either ferrous or ferric state (binding rate constants for ferrous and ferric cytochrome-Cs: 8.3 M−1s−1 and 7.2x102 M−1s−1, respectively) [Citation43]. Of note, reported data indicate that ferric cytochrome-C appears to have a higher reactivity to NO than the ferrous counterpart, presumably because the heme-methionine bond may be more easily broken in the ferric state than ferrous state during conformational change [Citation44]. Our results seem to make sense when considering relatively low reactivities of ferric heme-irons and protein-thiols toward free NO when compared with ferrous heme-irons of oxyHb or oxyMb [Citation42, Citation45]. except when the ferrous heme sites are already liganded by other NO molecules.
Finally, we investigated whether there are documented incidences of cardiovascular pathophysiology in subjects with Hb variants in which βCys93 are deleted or substituted with other residues (e.g., Hb Okazaki, Hb Cleveland). These human Hb variants of βCys93 generally show an increased O2 affinity but reports of serious hemodynamic abnormalities have not been found in the literature search. Of note, our findings are consistent with those of a recent study conducted using a transgenic mice model expressing either human wild-type Hb or human Hb in which the βCys93 residues are replaced with alanine (βCys93A) to assess the role of SNO-Hb in red blood cell–mediated hypoxic vasodilation [Citation28]. Substitution of βCys93 residues, thereby precluding hemoglobin S-nitrosation, did not change total red blood cell S-nitrosothiol abundance but did shift S-nitrosothiol distribution to lower molecular weight species, consistent with the loss of SNO-Hb. Vaso-activities of red cells from normal and βCys93A animals were essentially identical when tested on isolated rabbit pulmonary arterial rings [Citation28]. Substitution of βCys93 with alanine did modestly increase oxygen affinity as in human Hb variants but did not elicit significant changes in systemic or pulmonary hemodynamics under basal and exercise stress conditions. Increased O2 affinity, lower co-operativity and reduced Bohr effect relative to wild type human Hb have also been observed in a study using recombinant Hbs in which βCys93 was substituted with ala-nine, glutamine, lysine or methionine [Citation19]. These results also support the notion of SNO-Hb not being essential for the physiologic coupling of erythrocyte deoxygenation with increased NO bioactivity in vivo.
We conclude in the isolated rat thoracic aorta ring model that reactive thiols of beta globins do not appear to play a prominent role relative to heme-irons in acellular Hb-mediated vasoactivity.
Declaration of interest: The authors report no conflicts of interest. The authors alone are responsible for the content and writing of the paper.
This paper was first published online as an Early Online article on 5 January 2010.
REFERENCES
- Kocian, R. and Spahn, D.R. (2008). Haemoglobin, oxygen carriers and perioperative organ perfusion. Best Pract Res Clin Anaesthesiol 22:63–80.
- Kim, H.W. and Greenburg, A.G. (2004). Artifcial oxygen carriers as red blood cell substitutes: a selected review and current status. Artifcial Organs 28:819–828.
- Silverman, T., Landow, L., Ko, H.S., and Lindsey, K. (2008). Review of publicly available reports of adverse events associated with HBOCs. Public Workshop: Safety of Hemoglobin-Based Oxygen Carriers (HBOCs), April. (http://www.fda.gov/downloads/BiologicsBloodVaccines/NewsEvents/WorkshopsMeetingsConferences/ucm092845.pdf)
- Reah, G., Bodenham, A.R., Mallick, A., Daily, D.K., Przybelski, R.J. (1997). Initial evaluation of diaspirin cross-linked hemoglobin (DCL-Hb(R)) as a vasopressor in critically ill patients. Crit Care Med 25:1480–1488.
- Kasper, S.-M., Walter, M., Griine, F., Bischoff, A., Erasmi, H., Buzello, W. (1996). Effects of a hemoglobin-based oxygen carrier (HBOC-201) on hemodynamics and oxygen transport in patients undergoing preoperative hemodilution for elective abdominal aortic surgery. Anesth Analg 83:921–7.
- Cheng, D.C., Mazer, C.D., Martineau, R., Ralph-Edwards, A., Karski, J., Robblee, J., Finegan, B., Hall, R.I., Latimer, R., Vuylsteke, A. (2004). A phase II dose-response study of hemoglobin raffimer (Hemolink) in elective coronary artery bypass surgery. J Thorac Cardiovasc Surg 127:79–86.
- Criteria for Safety and Efficacy. (2004). Evaluation of Oxygen Therapeutics as Red Blood Cell Substitutes. Draft Guidance, Center for Biologics Evaluation and Research, FDA. October 2004.
- Martin, W., Gina, M., Jothianandan, D. and Furchgott, R.F. (1985). Selelctive blockade of endothelium-dependent and glyceryl trinitrate-induced relaxation by hemoglobin and by methylene blue in the rabbit aorta. J Pharmacol Exp Ther 232:708–716.
- Martin, W., Smith, J.A., White, D.G. (1986). The mechanisms by which hemoglobin inhibits the relaxation of rabbit aorta induced by nitrovasodilators, nitric oxide, or bovine retractor penis inhibitory factor. Br. J. Pharmac 89:563–571.
- Kim, H.W. and Greenburg, A.G. (2000). Pharmacodynamic characterization of hemoglobin-induced vasoactivity in isolated rat thoracic aorta. J. Lab. Clin. Med 135:180–187.
- Kim, H.W. and Greenburg, A.G. (1997). Ferrous hemoglobin scavenging of endothelium derived nitric oxide is a principal mechanism for hemoglobin mediated vasoactivities in isolated rat thoracic aorta. Art. Cells Blood Subs., and Immob. Biotech 25:121–133.
- Jia, L., Bonaventura, C., Bonaventura, J., Stamler, J.S. (1996). S-nitrosohemoglobin: a dynamic activity of blood involved in vascular control. Nature 380:221–226.
- Stamler, J.S., Jia, L., Eu, J.P, . (1997). Blood flow regulation by S-nitrosohemoglobin in the physiological oxygen gradient. Science 276:2034–2037.
- Gow, A.J. and Stamler, J.S. (1998). Reactions between nitric oxide and haemoglobin under physiological conditions. Nature 391:169–173.
- Jia, L. and Furchgott, R.F. (1993). Inhibition by sulfhydryl compounds of vascular relaxation induced by nitric oxide and endothelium-derived relaxing factor. J Pharmacol Exp Ther 287:371–378.
- Rigg, A. (1961). The binding of N-maleimide of human hemoglobin and its effect on oxygen binding equilibrium. J. Biol. Chem 236:1948–1954.
- Taylor, J.F., Antonini, E., Brunori, M., and Wyman, J. (1966). Studies of human hemoglobin treated with various sulfhydryl reagents. J. Biol. Chem 241:241–248.
- Boyer, P.D. (1954). Spectrophotometric Study of the reaction of protein sulfhydryl groups with organic mercurials. J. Am. Chem. Soc., 76:4331–4337.
- Cheng, Y., Shen, T.-J., Simplaceanu, V., and Ho, C. (2002). Ligand binding properties and structural studies of recombinant and chemically modified hemoglobins altered at β93 cysteine. Biochemistry 41:11901–11913.
- Edmundson, A.B. (1965). Aminos-acid sequence of sperm whale myoglobin. Nature 205:884–887.
- Romero-Herrera, A.E. and Lehmann, H. (1974). The amino acid sequence of human myoglobin and its minor fractions. Proc. R. Soc. Lond. B 186:249–279.
- Singel, D.J. and Stamler, J.S. (2005). Chemical physiology of blood flow regulation by red blood cells: the role of nitric oxide and S-nitrosohemoglobin. Annu Rev Physiol 67:99–145.
- McMahon, T.J., Moon, R.E., Luschinger, B.P., Carraway, M.S., Stone, A.E., Stolp, B.W., . (2002). Nitric oxide in the human respiratory cycle. Nat Med 8:711–717.
- Powloski, J.R., Hess, D.T., Stamler, J.S. (2001). Export by red blood cells of nitric oxide bioactivity. Nature 409:622–626.
- Luchsinger, B.P., Rich, E.N., Gow, A.J., Williams, E.M., Stamler, J.S., Singel, D.J. (2003). Routes to S-nitroso-hemoglobin formation with heme redox and preferential reactivity in the β subunits. Proc Natl Acad Sci U S A 100:461–466.
- McMahon, T.J., Ahearn, G.S., Moya, M.P., . (2005). A nitric oxide processing defect of red blood cells created by hypoxia: deficiency of S-nitrosohemoglobin in pulmonary hypertension. Proc Natl Acad Sci U S A 102:14801–14806.
- Gladwin, M.T., Lancaster, J.R., Freeman, B.A. and Schecter, A.N. (2003). Nitric oxide's reactions with hemoglobin: a view through the SNO-storm. Nature Med 9:496–500.
- Isbell, T.S., Sun, C., Wu, L., . (2008). SNO-hemoglobin is not essential for red blood cell–dependent hypoxic vasodilation. Nature Med 14:773–777.
- Herold, S. (2003). Interaction of nitrogen monoxide with hemoglobin and the artefactual production of S-nitroso-hemoglobin. C.R. Biologies 326:533–541.
- Cassoly, R, Gibson, Q. (1975). Conformation, co-operativity and ligand binding in human hemoglobin. J Mol Biol 91:301–13.
- Kharitonov, V., Sundquist, A.R., and Sharma, V.S. (1995). Kinetics of nitrosation of thiols by nitric oxide in the presence of oxygen. J Biol Chem 270:28158–28164.
- Huang, K.T., Azarov, I., Basue, J., Kim-Shapiro, D.B. (2006). Lack of allosterically controlled intramolecular transfer of nitric oxide from the heme to cysteine in the β-subunit of hemoglobin. Blood 107:2602–2604.
- Crawford, J.H., White, C.R., Patel, R.P. (2003). Vasoactivity of S-nitrosohemoglobin: role of oxygen, heme, and NO oxidation states. Blood 101:4408–15.
- Gladwin, M. T., Shelhamer, J. H., Schechter, A. N., Waclawiw, M. A., Panza, J. A., Ognibene, F.P. & Cannon, R. O., III. (2000). Role of circulating nitrites and S-nitrosohemoglobin in the regulation of regional blood flow in humans. Proc. Natl. Acad. Sci. USA 97:11482–11487.
- Hille, R., Palmer, G. and Olson, J.S. (1977). Chain equivalence in reaction of nitric-oxide with hemoglobin. JBC 252:403–405.
- Irwin, D.C., Foreman, B., Morris, K., . (2008). Polymerized bovine hemoglobin decreases oxygen delivery during normoxia and acute hypoxia in the rat. Am J Physiol 295:H1090–H1099.
- Malhotra, A.K., Kelly, M.E., Miller, P.R., Hartman, J.C., Fabian, T.C., Proctor, K.G. (2003). Resuscitation with a novel hemoglobin-based oxygen carrier in a Swine model of uncontrolled perioperative hemorrhage. J Trauma 54:915–24.
- Gurney, J., Philbin, N., Rice, J, . (2004). A hemoglobin based oxygen carrier, bovine polymerized hemoglobin (HBOC-201) versus hetastarch (HEX) in an uncontrolled liver injury hemorrhagic shock swine model with delayed evacuation. J Trauma 57:726–738.
- Vane, L.A., Funston, J.S., Kirschner, R., . (2002). Comparison of transfusion with DCLHb or pRBCs for treatment of intraoperative anemia in sheep. J Appl Physiol 92(1):343–53.
- Serruys, P.W., Vrancks, P., Slagboom, T., . (2008). Haemodynamic effects, safety, and tolerability of haemoglobin-based oxygen carrier-201 in patients undergoing PCI for CAD. EuroIntervention 3:600–609.
- Spencer, N.Y., Hong, Z., Patel, R.P., Hogg, N. (2000). Reaction of S-nitrosoglutathione with the heme group of deoxyhemoglobin. J. Biol. Chem 75:36562–36567.
- Gladwin, M.T., Ognibene, F.P., Pannell, L.K., . (2000). Relative role of heme nitrosylation and β-cysteine 93 nirosation in the transport and metabolism of nitric oxide by hemoglobin in the human circulation. Proc Natl Acad Sci U S A 97:9943–8.
- Hoshino, M., Ozawa, K., Seki, H., Ford, P.C. (1993). Photochemistry of nitric oxide adducts of water-soluble iron (III) porphyrin and ferrihemoproteins studied by nanosecond laser photolysis. J Am Chem Soc 115:9568–9575.
- Takano, T., Dickerson, R.E. (1981). Conformation change of cytochrome c. I. Ferrocytochrome c structure refined at 1.5 A resolution. J Mol Biol 153:79–94.
- Sharma, V.S., Traylor, T.G., Gardiner, R., Mizukami, H. (1987). Reaction of nitric oxide with heme proteins and model compounds of hemoglobin. Biochemistry 26(13):3837–43.
- Harano, K., Harano, T., Shibata, S., Ueda, S., Mori, H., Seki, M. (1984). Hb Okazaki [beta 93(F8) Cys----Arg], a new hemoglobin variant with increased oxygen affinity and instability. FEBS Lett 173(1):45–7.
- Wilson, J.B., Ramachandran, M., Webber, B.B., . (1991). Hb Cleveland or alpha 2 beta 2(93)(F9)Cys----Arg;121(GH4)Glu----Gln. Hemoglobin 15(4):269–78.
- Morlé, F., Francina, A., Ducrocq, R., . (1995). A new alpha chain variant Hb Sallanches [alpha 2 104(G11) Cys-->Tyr] associated with HbH disease in one homozygous patient. Br J Haematol 91(3):608–11.
- Harano, T., Harano, K., Kushida, Y., Ueda, S. (1991). A new abnormal variant, Hb Yahata or beta 112(G14) Cys----Tyr, found in a Japanese: structural confirmation by DNA sequencing of the beta-globin gene. Hemoglobin 15:109–13.