Abstract:
β-galactosidase of Aspergillus sp. AF crude homogenate was immobilized in Ca-alginate gel beads and used for the production of galactooligosaccharides (GOS) from lactose. Optimum pH and temperature, thermal and storage stability of the enzyme activity were investigated and compared with those of the free enzyme. The study on the improvement of mechanical strength of the alginate beads was carried out through various methods, which demonstrates that the hardening process, where the alginate beads were treated with 0.225 M CaCl2 solution after three batches to compensate the lost of calcium in the beads, provided a high mechanical stability for repeated use in large-scale production. The experiment results show that GOS yield increased with the increase of lactose concentration, and also increased with excessive addition of lactose (exceeding its solubility) at the beginning of the reaction. The immobilization of β-galactosidase of Aspergillus sp. AF crude homogenate is cheap in processing cost and easy to carry out, and the immobilized enzyme possesses high performance for industrial application.
INTRODUCTION
Galactooligosaccharides (GOS), non-digestible oligosaccharides, were applied to stimulate the growth of bifidobacteria and to change the ecological balance of the intestinal microflora [Citation15,Citation18,Citation32]. They are considered to be used as prebiotics in functional food [Citation5,Citation12,Citation29,Citation35]. Enzyme-catalyzed synthesis of oligosaccharides represents an interesting alternative to the classical chemical methods [Citation9,Citation14].
For the industrial application, the immobilized form of enzyme offers several advantages as compared with soluble enzyme, including repeated use of the enzyme, easy separation from product and enhancement of enzyme stability. Entrapment of enzyme inside a polymeric matrix is one of the most widely used techniques for enzyme immobilization [Citation1,Citation8,Citation20]. Calcium alginate has been used frequently, mainly due to its mild operation conditions and fast diffusion of the substrates and products through the pores of the matrix. Other advantages of its corresponding technique are the short operation time, simple procedure, and low operation cost [Citation8]. During the repeated use of the immobilized enzyme, the alginate beads prepared by calcium alginate may become softer; therefore harder beads are required for a larger-scale bioreactor. It was reported that addition of Ca2+ into the substrate [Citation17,Citation24] or pretreatment of Ca-alginate beads with polyethyleneimine [Citation3,Citation10] or glutaraldehyde [Citation22] can promote mechanical strength and prevent the beads from swelling and dissolving.
Some β-galactosidases, which are used to produce GOS, were purified from bacteria [Citation16,Citation28] and fungi [Citation6,Citation19,Citation21,Citation27,Citation28,Citation34]. A few reports are available about the immobilized β-galactosidase in the synthesis of oligosaccharides [Citation4]. There is no report on the immobilization of β-galactosidase of fungi crude homogenate that do not require extraction and purification steps as compared with the purified enzymes.
In this work, the differences of activity and stability between the immobilized β-galactosidases of Aspergillus sp. AF (in the form of crude homogenate in Ca-alginate gel) and free enzyme are to be investigated. Effective techniques will be applied for the enhancement of mechanical strength of the immobilized enzymes during their repeated use. Optimization of the reaction conditions for the maximum production yield of GOS will be performed.
MATERIALS AND METHODS
Materials
β-galactosidase (EC 3.2.1.23) homogenate, which is a cell debris suspension disrupted from Aspergillus sp. AF in 0.05 M, pH 5.5 acetate buffer (weight ratio of β-galactosidase and acetate buffer is 1), was supplied by the Department of Microbiology, Yantai University. The activity of the β-galactosidase was 180 U/g (one unit of enzyme activity was defined as theamount of enzyme that released 1µ mol o-nitrophenol from o-nitrophenyl-β-D-galactopyranoside (ONPG) solution per min at pH 5.5 and 40°C). ONPG, standard glucose and lactose were purchased from Sigma (St. Louis, Mo.). All the other chemicals used were of analytical grade.
Methods
Immobilization of β-galactosidase. 20 g of 4% (w/v) Na-alginate were mixed with 10 g of β-galactosidase homogenate, followed by the dropwise extruding with a syringe into 100 ml 0.225 M CaCl2 solution. After being kept at 4°C for overnight, the beads of calcium alginate were transferred into 50 ml 0.135 M CaCl2 solution for storage in refrigerator. The average bead diameter was about 3 mm.
Determination of the activity of free β-galactosidase. 0.15 ml β-galactosidase homogenate was added to 0.4 ml reaction solution that consisted of 2.0 mM ONPG in 0.05 M acetate buffer (pH = 5.5). The mixture solution was incubated on a rotary shaker with 150 rpm for 10 min at 40°C, except for the determination in part 2.6. The reaction was stopped by adding 3 ml 0.5 M sodium carbonate solution, followed by centrifuging at 4800 rpm for 10 min. Absorbance of the supernatant liquid was measured at 405 nm where it showed the maximum absorbance. The principle of this method [Citation9] is based on the knowledge that the increase of absorbance results from the liberation of o-nitrophenol that was produced by the hydrolysis of o-nitrophenyl-β-D-galactoside.
Determination of the activity of immobilized β-galactosidase. Beads were washed with distilled water twice, and touch-dried with filter paper before the activity determination of immobilized β-galactosidase. Then 0.45 g of the beads (about 26 beads), equivalent to the amount of 0.15 ml β-galactosidase homogenate, was used to measure immobilized β-galactosidase activity. The following determination procedure was the same as described in the above paragraph. The relative activity was expressed in percentage of the maximum activity under the corresponding conditions.
Determination of swelling degree. Swelling degree was defined as the ratio of the weight of 26 beads before and after reaction.
HPLC analysis. The oligosaccharides were analyzed by HPLC. 1.5 ml sample solution was centrifuged at 10,000 rpm for 10 min. The supernatant (1.0 ml) was diluted 10-fold and filtered through a 0.22 µm cellulose-acetate membrane (F-0139, Sigma) before injection into the HPLC system. The HPLC system was an Agilent 1200 (USA) instrument with a G1362A refractive index detector. The Aichrom-NH2 column from Abel industries Co. (USA), with a size of 4.6×250 mm, was maintained at 30°C, where a mixture of acetonitrile and water (75:25) was used as mobile phase at a flow rate of 1mL/min.
Effect of temperature on activities of free enzyme and immobilized enzyme. The activities of free enzyme and immobilized enzyme were measured at pH 6.5 (0.05 M phosphate buffer) between 20 and 70°C.
Thermal stability of β-galactosidase. 0.15 ml β-galacto-sidase homogenate or 0.45 g of beads samples suspended in 2 ml 0.05 M phosphate buffer (pH = 6.5) was treated at different temperatures for 24 h. Then, the remaining enzyme activity was measured at 40°C.
Effect of pH on activities of free enzyme and immobilized enzyme. The activities of free enzyme and immobilized enzyme were measured in buffer solutions from pH 2.2 to 8.0. The buffers (0.05 M) were citrate buffer (pH = 2.2–3.4), acetate buffer (pH = 4.0–5.4), and phosphate buffer (pH = 6.0–8.0).
pH stability of β-galactosidase. 0.15 ml β-galactosidase homogenate or 0.45 g of beads samples were suspended in 2 ml buffer solutions from pH 2.2 to 8.0. The remaining enzyme activity was measured after they were placed at 4°C for 24 h. The buffers (0.05 M) were citrate buffer (pH = 2.2–3.4), acetate buffer (pH = 4.0-5.4), and phosphate buffer (pH = 6.0–8.0).
Storage stability of β-galactosidase. The free enzyme was stored at 4°C in phosphate buffer (0.05 M, pH 6.5). On the other hand, the immobilized enzymes were stored at 4°C, respectively, in various solutions: (A) phosphate buffer (0.05 M, pH 6.5); (B) 0.045 M CaCl2 aqueous solution; (C) 0.135 M CaCl2 aqueous solution; (D) 0.225 M CaCl2 aqueous solution; (E) 0.09 M NaCl aqueous solution. The storage stability of the enzyme preparations was determined at intervals of 5 days.
Improvement of mechanical strength of the alginate beads. In order to enhance the mechanical strength of the alginate beads for their repeated use in industrial production, several immobilizing techniques (or modifications of operational conditions) were used:
(A) The immobilizing method is described in part 2.2, and the alginate beads were used as control.
(B) 1 g kaolin and 20 g 4% (w/v) Na-alginate were mixed with 10 g β-galactosidase homogenate for the preparation of alginate beads.
(C) 1g diatomite and 20 g 4% (w/v) Na-alginate were mixed with 10 g β-galactosidase homogenate for the preparation of alginate beads.
(D) The initial preparation of the alginate beads was the same as (A), and then the beads were crosslinked with 0.5% (w/v) glutaraldehyde for 1 h. The glutaraldehyde solution was withdrawn and the alginate beads were washed with distilled water.
(E) The initial preparation of the alginate beads was the same as (A), and then the beads were crosslinked with 2% (w/v) polyethylenimine (PEI) for 20 h. The PEI solution was withdrawn and the alginate beads were washed with distilled water.
(F) The immobilizing process was the same as (A); however, the reaction solution contains additional CaCl2 with concentration of 0.135 M.
(G) The immobilizing method was the same as (A). The difference is that the alginate beads were immersed in 0.225 M CaCl2 solution for 0.5 h after each cycle.
The 10 g above-mentioned immobilized enzymes and 30 g lactose were added into 250 ml capped conical flasks containing 50 ml phosphate buffer (0.05 M, pH 6.5). Reaction was carried out at 50°C and 150 rpm on a rotary shaker.
Preparation of galactooligosaccharides. 10–50 g immobilized enzymes and 15–45 g lactose were added into 250 ml capped conical flasks containing 50 ml buffer solutions (pH 3.0–6.0). Reaction was carried out at 45°C or 50°C and 150 rpm on a rotary shaker. Samples were withdrawn at time intervals of 4 hours and assayed by HPLC. The adopted lactose concentrations in the study are expressed as weight percent lactose of the buffer volume (% w/v). The yield of GOS (sum of tri-, tetra- and pentasaccharides) is the weight percent GOS of the total sugar weight (% w/w).
Statistical analysis. All experiments were done in triplicate and all assays were carried out three times. The results have been expressed as the mean values ± SD. One-way ANOVA was used to compare the values for each of experimental groups. Tukey's honest significant difference (HSD) test was used to distinguish the difference between two experimental groups. The P-value of less than 0.05 (P < 0.05) was considered to be with statistical significant difference.
RESULTS AND DISCUSSION
Effects of Temperature and pH on Activities of Free Enzyme and Immobilized Enzyme
Effect of temperature. As shown in , the maximum activity is observed at 55°C for immobilized enzyme and at 50°C for free enzyme. However, the immobilized enzyme has a little broader temperature range than that of the free one in the high temperature region with no significant difference of activity in the temperature range of 20–45°C. When the temperature was higher than 60°C for immobilized enzyme and above 50°C for free enzyme, an obvious loss of activity was observed. Below 40°C, the activity for both forms of enzymes decreased substantially. In the range of 40–60°C, the two enzymes are very stable and possess activity ≥80% of the original values; therefore, the experiments were performed at 40–60°C to examine their thermal stability.
Figure 1. Effect of temperature on the activity of immobilized (•) and free (▴) enzyme at pH 6.5. The highest activity is referred to as 100%.
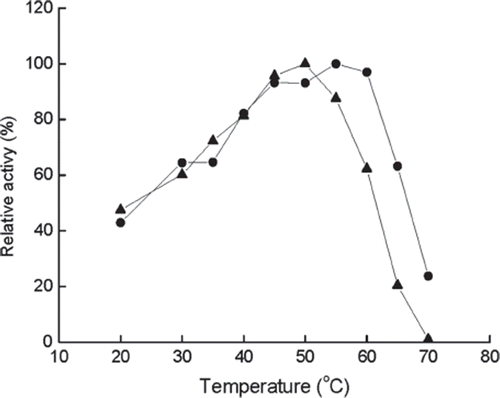
Effect of pH. As presented in , the immobilized enzyme exhibits broad peak of optimum pH in the acid region between pH 3.0 and pH 6.0, having little difference of activity. For free enzyme, the optimum pH is between 4.0 and 5.0. Beyond the optimum pH, the activity of the immobilized enzyme shows a slower developing trend with the change of pH and better stability as compared with the free enzyme. This demonstrates that the third class configuration is more stable after the enzyme is immobilized; therefore, the effect of PH on the enzyme active is weakened. The immobilized enzyme has better adaptability to the environmental acidity and basicity.
Effects of Temperature and pH on the Stability of Free Enzyme and Immobilized Enzyme
Thermal stability. clearly indicates that the activity of the immobilized enzyme is more thermostable than that of the free enzyme. For example, the activity of the immobilized enzyme remains about 70% at 60°C, while no activity is detected for the free enzyme. The thermal stability of the immobilized enzyme can be obtained in the range of 40–50°C, while a narrow temperature range of 40–45°C is observed for the free enzyme. At temperature of higher than 50°C, the remaining activity of both immobilized enzyme and free enzyme decreases with the increase of temperature. However, the free enzyme possesses a faster decreasing trend of activity than the immobilized one. The thermal stability of immobilized enzyme is usually very important for its industrial application. In several studies of a-galactosidases obtained from different sources, the thermal stability was also increased by immobilization [Citation13,Citation20].
Figure 3. Thermal stability of immobilized (•) and free (▴) enzymes at different temperature and pH 6.5 for 24 h. The highest activity is referred to as 100%.
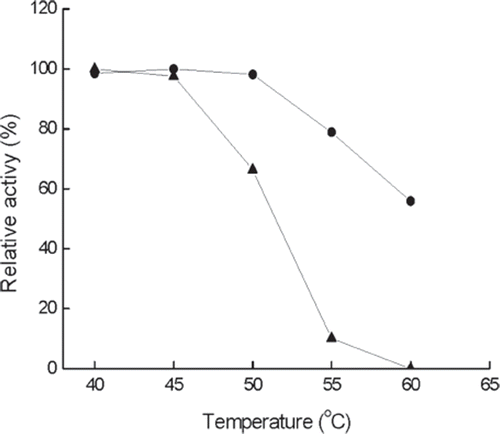
pH stability. As shown in , the immobilized enzyme is more stable than the free one at various pHs, especially in high acidity and basicity. For example, at pH 2.6 free enzyme loses more than 30% activity after 24 h, while the immobilized enzyme retains almost the same activity. Under more alkaline conditions such as pH 7.0, the free enzyme loses more than 90% activity after 24 h, while the immobilized enzyme retains around 40% activity, indicating that the resistance of β-galactosidase to acid and basic denaturation is increased remarkably after immobilization.
Storage Stability of Free Enzyme and Immobilized Enzyme
shows the difference of storage stability between the free and immobilized enzymes at 4°C. After the immobilized enzyme is stored in a phosphate buffer (0.05 M, pH 6.5) in the refrigerator at 4°C only for 5 days, disintegrated alginate beads appeared, probably due to the complex reaction between Ca2+ in alginate beads and PO43– in phosphate buffer [Citation30]. Hence, the data of the immobilized enzyme stored in phosphate buffer (0.05 M, pH 6.5) is not presented in .
Figure 5. Storage stability of immobilized and free enzymes at 4°C. The highest activity is referred to as 100%. ▴, free enzyme in phosphate buffer (0.05 M, pH 6.5); •, immobilized enzyme in 0.045 M CaCl2 aqueous solution; ♦, immobilized enzyme in 0.135 M CaCl2 aqueous solution; ▾, immobilized enzyme in 0.225 M CaCl2 aqueous solution; ◂, immobilized enzyme in 0.09 M NaCl aqueous solution.
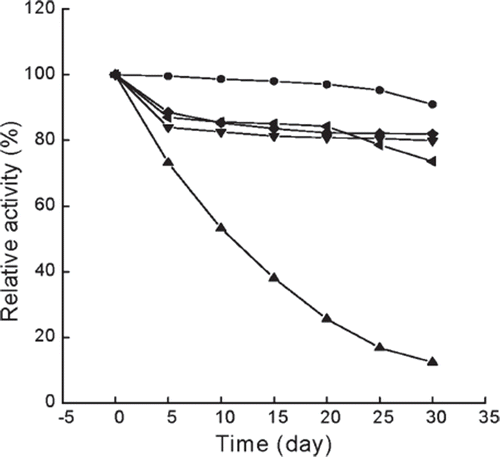
The immobilized enzymes retained about 80% (in 0.135 M, 0.225 M CaCl2 or 0.09 M NaCl solution) or 90% (in 0.045 M CaCl2 solution) of their original activities after 30 days, whereas the free enzyme lost about 90% of its original activity during the same period of time. The immobilized enzyme stored in 0.135 M or 0.225 M CaCl2 solution showed lower storage stability than in 0.045 M CaCl2, which may be caused by the inhibitive effect of high CaCl2 concentration on β-galactosidase. However, the 0.045 M CaCl2 solution became somewhat turbid and gave off an unbearable odor over 20 days of storage, mainly due to the low osmosis pressure of the solution, which caused the dissolution of alginate gel, resulting in the contamination by microorganisms. The remarkable turbidity and terrible smell in 0.135 M CaCl2 and 0.09 M NaCl solutions were also observed after the same time. In addition, the alginate beads in 0.09 M NaCl solution exhibited a significant swelling effect, due to the replacement of Ca2+ of alginate gel by Na+ in storage solution, resulting in less calcium ion bounding to the alginate beads, and then a mild gel solution was observed. But the 0.225 M CaCl2 aqueous solution still remained clear within 25 days, and only slightly turbid after 30 days. This fact indicates that longer storage requires higher concentration of CaCl2 aqueous solution. Various reports also confirm that the storage stability of immobilized enzyme depends on the storage conditions [Citation11,Citation20,Citation36].
Operation Stability of Immobilized Enzyme and the Corresponding GOS Yield
The activity and swelling degree of the immobilized enzyme were measured before the first cycle and after each cycle ( and , except for crosslinker PEI). In contrast to immobilized cells [Citation3,Citation11], the immobilized β-galactosidase retained almost zero activity after being crosslinked with PEI, probably due to denaturation of β-galactosidase by this agent. So this method was not further performed in this test. The yield of GOS after each cycle is presented in . As shown in and , the immobilized enzyme loses about 80% of activity after the initial cycle in all cases, except for the one pretreated by 0.225 M CaCl2 solution. The activity of the immobilized enzyme decreases gradually in the ensuing cycles, and the residual activity is only around 15% after 3 cycles.
Figure 6. Variations in the relative activities versus operational cycles for the immobilized enzymes prepared or treated with different methods. ▪, normal immobilized method, as control; •, co-immobilized with kaolin; ▴, co-immobilized with diatomite; ▾, crosslinked with glutaradeyde after immobilization; ♦, presence of 0.135 M CaCl2 in reaction solution; ◂, immersion in 0.225 M CaCl2 aqueous solution after each cycle, the activity is determined after the immersion for 0.5h. The intial activity of the control is referred to as 100%.
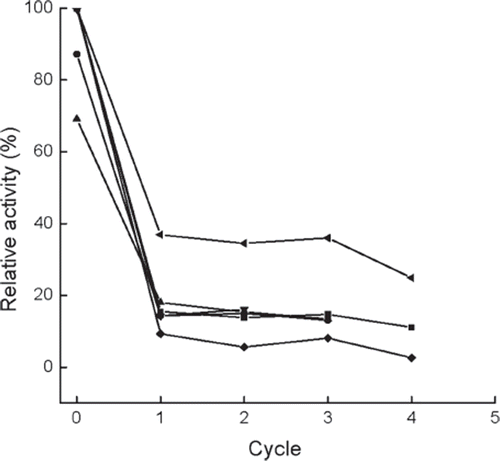
Figure 7. Variations in swelling degrees versus operational cycles for the immobilized enzymes prepared or treated with different methods. ▪, normal immobilized method, as control; •, co-immobilized with kaolin; ▴, co-immobilized with diatomite; ▾, crosslinked with glutaradeyde after immobilization; ♦, presence of 0.135 M CaCl2 in reaction solution; ◂, immersion in 0.225 M CaCl2 aqueous solution after each cycle, swelling degree is detemined afterthe immersion for 0.5h. Swelling degree is defined as the ratio of theweight of 26 beads of the immobilized enzyme with relatively even particle size before the first cycle to that after reaction of the corresponding cycle.
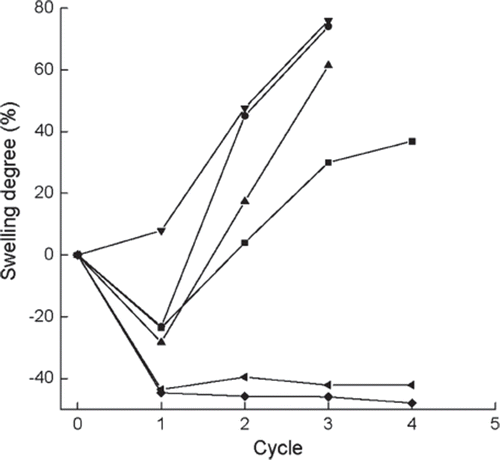
Figure 8. Variations in GOS yields versus operational cycles for the immobilized enzymes prepared or treated with different methods. ▪, normal immobilized method, as control; •, co-immobilized with kaolin; ▴, co-immobilized with diatomite; ▾, crosslinked with glutaradeyde after immobilization; ♦, presence of 0.135 M CaCl2 in reaction solution; ◂, immersion in 0.225 M CaCl2 aqueous solution after each cycle.
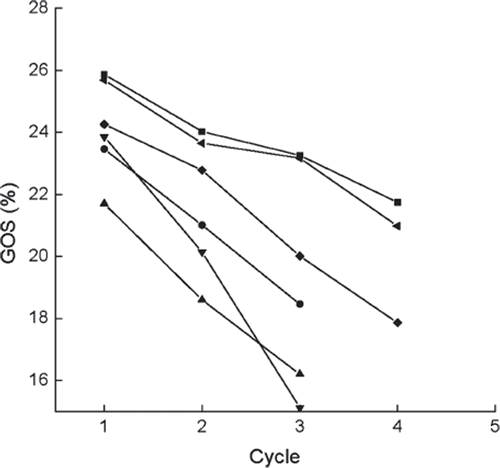
As concluded from , , and , the immobilized enzyme modified with glutaraldehyde gives no significant loss of activity, but presents lower GOS yield and does not increase the mechanical strength, which means that the treatment of the enzyme with glutaraldehyde is no longer effective. Neither mechanical strength nor GOS yield is improved for the co-immobilization of kaolin or diatomite with Ca-alginate. Although adding Ca2+ into the substrate can enhance the mechanical strength of the immobilized bead, a significant decrease of GOS yield occurs, probably due to the inhibiting effect of Ca2+ in the enzymatic reaction, which is consistent with other immobilized enzymes [Citation17,Citation24].
When the immobilized enzyme is pretreated with 0.225 M CaCl2 aqueous solution before reuse, higher remaining activity and harder beads can be achieved, although no increase of GOS yield is observed as compared with the control. But after each cycle and before pretreatment with 0.225 M CaCl2 aqueous solution, the remaining activity is similar to that of the control (data not shown), which means that the treatment of CaCl2 solution can greatly recover the hydrolase activity. From an industrial point of view, the suitability of the immobilized enzyme for reuse is considered a major advantage. In this work, if the swelling degree is more than 50%, the alginate beads are difficult to reuse further and need to be immersed in CaCl2 aqueous solution to increase the mechanical strength. Therefore, the alginate beads are further treated by 0.025 M CaCl2 aqueous solution after every three batches in the following experiments.
Optimal pH and Temperature for the Production of Galactooligosaccharides
PH plays an important role in the mechanical stability of alginate beads [Citation2,Citation26]. However the operational pH might be different from the optimal pH of the enzyme [Citation7,Citation33]. In the present study, effects of pH and temperature on GOS synthesis and mechanical stability of alginate beads are investigated in the pH range 3.0–6.0 at 45°C and 50°C.
After the first cycle, a pH range of 3.0–4.2 gives low yields of GOS for immobilized enzyme (data not shown), therefore tests are continued at pH 4.6-6.0. The average GOS yields over 10 cycles are summarized in . It can be seen that the optimum pH for GOS production is between 4.6 and 5.4; temperature at 45°C and 50°C seems to give no significant effect on the yield of GOS, which is opposite to the reports of some researchers [Citation9,Citation37]. Although there is no significant effect of temperature, the amount of precipitate formed in sample obtained at 50°C is 2 times higher than that of sample obtained at 45°C after storage for three days in the refrigerator, which can be attributed to the higher dissolution of alginate gel into reaction solution at higher temperature. In order not to contaminate the GOS product, the optimum temperature is determined to be 45°C.
Table 1. Average yields of GOS over 10 cycles at different temperatures and pH values
The swelling degree of alginate beads is measured during the cycle at 45°C (data not shown). At pH 6.0, the swelling degree increases dramatically after 2 cycles, showing visible disintegration of alginate beads. This may be explained by the fact that the buffer used is phosphate buffer at pH 6.0, whereas Ca2+ in alginate beads is easily lost due to the complex with phosphate buffer [Citation30]. During the operating cycle, the lowest increase in swelling degree is observed at pH 4.6, indicating the maximum stability of alginate beads is at pH 4.6. Taking into consideration the GOS yield and mechanical stability of alginate beads, the optimum pH is determined to be 4.6.
Effects of Reaction Time and Alginate Beads/Reaction Solution Ratio (w/v) on the GOS Yield
shows the curve of reaction time versus the yield of GOS within a ratio range of alginate beads to reaction solution between 1:1 and 1:10. It is observed that GOS yield increases with the increasing of the alginate beads amount. With sufficient alginate beads (ratio >1:2), the yield of GOS is almost independent of the amount of alginate beads. Increasing the ratio from 1:10 to 1:1, the optimal reaction time, at which the maximum GOS yield is obtained, is reduced from 44 h to 32 h. After the maximum point of GOS yields, GOS yield decreases slowly due to the sequential hydrolysis of GOS, which is similar to the result of Hansson and Adlercreuts [Citation9] where the reaction time at maximum yield is very short in all cases. The 1:10 ratio of the immobilized enzyme to reaction solution is too low to give a good yield of tetrasaccharides. To obtain a high reaction rate and guarantee the maximum yield, a ratio of 1:2 is optimally used.
Effect of Substrate Concentration on the GOS Yield
Effect of substrate (lactose) concentration on the yield of GOS synthesis was studied in using various concentrations. When the initial lactose concentration increases from 15% to 30% (w/v), the yield of GOS, increased by 30%, indicates that GOS synthesis from lactose is favored by high lactose concentrations, which is in agreement with the results of other researchers [Citation9,Citation23,Citation25,Citation31,Citation37].
Due to the low solubility of lactose in water, lactose concentration of 30% (w/v) is the upper limit at 45oC. We consider whether the yield of GOS can be increased with excessive additions of lactose at the beginning of the reaction, which is equivalent to successive additions of lactose to keep the lactose solution in saturated state for a longer time. illustrates variations in the GOS yield between the original lactose concentration of 15% (w/v) and 90% (w/v).
Figure 10. Effect of lactose concentration on the maximum yield of GOS and optimal time. Reaction conditions: 10 g alginate beads in 50 ml buffer, 45°C and pH 4.6, lactose concentration %(w/v) referred to be weight per unit volume solvent. □, Optimum yield; •, Optimal time.
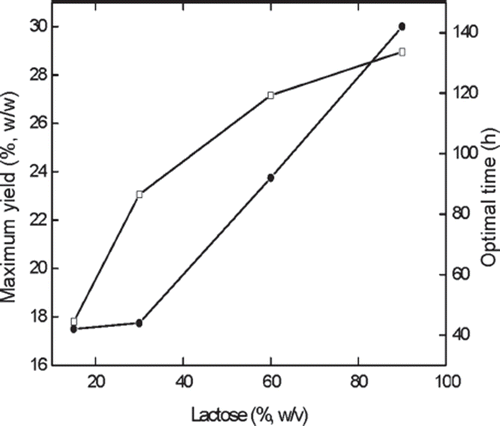
As presented in , the maximum GOS yield increases with the substrate concentration up to 90% (w/v) lactose. However, the increasing extent differs at various concentration regions by about 30% and 18% increase in the region 15–30% (w/v) and 30–60% (w/v), respectively, showing a greater extent at lower concentration region, and only a slight increase (about 7%) in the higher concentration region 60–90% (w/v). This may be due to the increased amount of formed galactose inhibiting the enzymatic reaction, or may be due to the excess formation of some oligosaccharides at higher concentrations of substrate, which retard the rate of hydrolysis [Citation25,Citation27]. The optimal reaction times at which GOS yields reach the maximum at corresponding lactose concentrations are also depicted in . It shows that the optimal time increases linearly with the increase of lactose concentration; however, in the concentration range of 15–30% (w/v) the optimal time is almost unchanged. Although the initial concentration of excessive saturated solution is 60% (w/v) or 90% (w/v) at 45°C, the effective concentration is only 30%, and complete solubilization of the solid lactose occurs after 21 h and 53 h at initial concentration of 60% (w/v) and 90% (w/v), respectively. The reduction of cost in the subsequent downstream process resulting from higher yield of GOS is very important for practical application. Thus the suitable concentration of lactose and optimal reaction time is determined to be 60% (w/v) and 92 h, respectively.
CONCLUSION
Ca-alginate entrapment of β-galactosidase is a very easy and inexpensive immobilization method. The immobilized β-galactosidase of Aspergillus sp. AF presents the optimal activity in a broader temperature and pH range, and exhibits remarkable thermal and storage stability at medium acidity and reaction temperature, as well as better reusability as compared with a free enzyme. As far as the mechanical strength of the immobilized enzyme in operation is concerned, treatment with 0.225 M CaCl2 after every three batches is sufficient to retain a relatively rigid gel for repeated use in the practical application. Optimally designed reaction conditions for GOS production using immobilized enzyme are 60% lactose solution at 45°C and pH 4.6 with immobilized enzyme/ reaction solution ratio of 1:2.
Declaration of interest: The authors report no conflicts of interest. The authors alone are responsible for the content and writing of the paper.
REFERENCES
- Aranaz, I., Ramos, V., De La Escalera, S., Heras, A. (2003). Coimmobilization of D-hydantoinase and D-carboamylase on Chitin: Application to the synthesis of p-hydroxyphenylglycine. Bifidobact. Microflora 21:349–356.
- Arruda, L. M. O., Vitolo, M. (1999). Characterization of invertase entrapped into calcium alginate beads. Appl. Biochem. Biotechnol 81:23–33.
- Bahulekar, R., Ayyangar, N. R., Ponrathna, M. S. (1991). Polyethyleneimine in immobilization of biocatalysts. Enzyme. Microb. Technol 13:858–868.
- Berger, J. L., Lee, B. H., Lacroix, C. (1995). Oligosaccharides synthesis by free and immobilized β-galacotosidades from Thermus aquaticus YT-1. Biotechnol Lett 17:1077–1080.
- Bhat, M. K. (1998). Oligosaccharides as functional food ingredients and their role in improving the nutritional quality of human food and health. Recent. Res. Dev. Agric. Food. Chem 2:787–802.
- Cho, Y. J., Shin, H. J., Bucke, C. (2003). Purification and biochemical properties of a galactooligosaccharide producing β-galactosidase from Bullera singularis. Biotechnol. Lett 25:2107–2111.
- Diao, Y. H., Wand, Q. L., Fu, S. Y. (2002). Laccase stabilization by covalent binding immobilization on activated polyvinyl alcohol carrier. Lett. Appl. Microbiol 35:451–456.
- Groboillot, A., Boadi, D. K., Poncelet, D., Neufeld, R. J. (1994). Immobilization of cells for application in the food industry. Crit. Rev. Biotechnol 14:75–107.
- Hansson, T. Adlercreutz P. (2001). Optimization of galactooligosaccharide production from lactose using β-glycosidases from hyperthermophiles. Food. Biotechnol 15:79–97.
- Joung, J. J., Aki, N. C., Royer, G. P. (1987). Immobilization of growing cells by polyethyleneimine-modified alginate. Appl. Biochem. Biotechnol 14:259–275.
- Krajewska, B., Leszko, M., Zaborska, W. (1990). Urease immobilized on chitosan membrane: preparation and properties. J. Chem. Technol. Biotechnol 48:337–350.
- Kunz, C., Radloff, S. (1993). Biological functions of oligosaccharides in human milk. Acta. Paediatr 82: 903–912.
- Mitsutomi, M., Uchida, Y., Ohtakara, A. (1985). Immobilization of thermostable α-galactosidase from Pyconoporus cinnabarinus on chitin and some properties of the immobilized enzyme. J. Ferment. Technol 163:325–329.
- Moracci, M., Trincone, A., Rossi, M. (2001). Glycosynthases: new enzymes for oligosaccharide synthesis. J. Mol. Catal. B: Enzym 11:155–163.
- Morishital, Y., Oowada, T., Ozaki, A., Mizutani, T. (2002). Galactooligosaccharide in combination with Bifidobacterium and Bacteroides affects the population of Clostridium perfringens in the intestine of gnotobiotic mice. Nutr. Res 22:1333–1341.
- Mozaffer, Z., Nakanishi, K., Matsuno, R., Kamikubo, T. (1984). Purification and properties of β-galactosidase from Bacillus circulans. Agric. Biol. Chem 48:3053–3061.
- Nasri, M., Sayadi, S., Barbitin, J. N. (1987). Influence of immobilization on the stability of pT Escherichia coli. Appl. Environ. Microbiol 53:740–744.
- Ohkusa, T., Ozaki, Y., Sato, C., Mikuni, K., Ikeda, H. (1995). Long-term ingestion of lactosucrose increases Bifidobacterium sp. in human fecal flora. Digestion 56:415–420.
- Ohtsuka, K., Tanoh, A., Ozawa, O., Kanematus, T., Uchida, T., Shinke, R. (1990). Purification and properties of a β-galactosidase with high galactosyl transfer activity from Cryptococcus laurentii. J. Ferment. Bioeng 70:301–307.
- Önal, S. Telefoncu, A. (2003). Preparation and properties of a-galactosidase chemically attached to activated chitin. Artif. Cells. Blood. Substitues. Biotechnol 31:339–355.
- Onishi, N., Tanaka, T. (1995). Purification and properties of a novel thermostable galacto-oligosaccharide-producing β-galactosidase from Sterigmatomyces elviae CBS8119. Appl. Environ. Microbiol 61:4026–4030.
- Park, J. K., Chang, H. N. (2000). Microencapsulation of microbial cells. Biotechnol Adv 18:303–319.
- Petzelbauer, I., Zeleny, R., Reiter, A., Kulbe, K. D., Nidetzky, B. (2000). Development of an ultra-high-temperature process for the enzymatic hydrolysis of lactose: II. Oligosaccharide formation by two thermostable β-glycosidases. Biotechnol. Bioeng 69:140–149.
- Plainer, H., Spoerssler, B. G. (1982). Technical applications of lactase and amino acid acylase immobilized to form plexazym. Enzyme. Eng 6:293–294.
- Prenosil, J. E., Stuker, E., Bourne, J. R. (1987). Formation of oligosaccharides during enzymatic lactose hydrolysis and their importance in a whey hydrolysis process: Part II. Exp. Biotechnol. Bioeng 30:1026–1031.
- Quan, C. S., Fan, S. D., Ohta, Y. (2003). Immobilization of Candida krusei cells producing phytase in alginate gel beads: an application of the preparation of myo-inositol phosphates. Appl. Microbiol. Biotechnol 62:41–47.
- Rejikumar, S., Devi, S. (2001). Hydrolysis of lactose and milk whey using a fixed-bed reactor containing β-galactosidase covalently bound onto chitosan and crosslinked poly (vinyl alcohol). Int. J. Food. Sci. Technol 36:91–98.
- Reuter, S., Rusbory Nygaard, A., Zimmermann, W. (1999). β-Galactooligosaccharide synthesis with β-galactosidases from Sulfolobus solfataricus, Aspergillus oryzae, and Escherichia coli. Enzyme. Microb. Technol 25:509–516.
- Sako, T., Matsumoto, K., Tanaka, R. (1999). Recent progress on research and applications of non-digestible galacto-oligosaccharides. Int. Dairy J. 9:69–80.
- Segura, A. G. D., Alcalde, M., Plou, F. J., Remaudsimeon, M., Monsan, P., Ballesteros, A. (2003). Encapsulation in lentiKats of dextransucrase from leuconostoc mesenteroides NRRL B-1299, and its effect on product selectivity. Biocatal. Biotransform 21:325–331.
- Stevenson, D. E., Stanley, A. R., Furneaux, H. (1996). Oligosaccharide and alkyl-β-galactopyranoside synthesis with Caldocellum saccharolyticum β-glycosidase. Enzyme. Microb. Technol 18:544–549.
- Tanaka, R., Takayama, H., Morotomi, M., Kuroshima, T., Ueyama, S., Matsumoto, K., Kuroda, A., Mutai, M. (1983). Effects of administration of TOS and Bifidobacterium breve 4006 on the human fecal flora. Bifidobact. Microflora 2:17–24.
- Tischer, W., Kasche, V. (1999). Immobilized enzymes: crystals or carriers? Trends. Biotechnol 17:326–335.
- Toba, T., Yokota, A., Adachi, S. (1985). Oligosaccharide structures formed during the hydrolysis of lactose by Aspergillus oryzae β-galactosidase. Food. Chem 16: 147–162.
- Tomomatsu, H. (1994). Health effects of oligosaccharides. Food. Technol 48:61–65.
- Vaillant, F., Millan, A., Millan, P., Dornier, M., Decloux, M., Reynes, M. (2000). Co-immobilized pectinlyase and endocellulase on chitin and nylon supports. Process Biochem 35:989–996.
- Zarate, S., Lopez-Leiva, H. (1990). Oligosaccharide formation during enzymatic lactose hydrolysis: A Literature Review. J. Food. Prot 53:262–268.