Abstract:
Chitin conduits to bridge nerve injuries with a small gap (2mm) give us a biological window in which the peripheral nerve regeneration process can be observed. In this study, the regeneration process was observed on different intervals postoperatively. Histological analysis revealed that the early regeneration process occurred in three phase: degeneration and matrix phase, axonal and Schwann migration phase, myelination and maturation phase. Schwann cells grew into the lumen from both the proximal and distal nerve segments. Axonal regrowth progressed at an average rate of 1.4 mm/d. Regenerating axonal fibers were myelinated by Schwann cells from both sides of the conduit.
INTRODUCTION
Posttraumatic nerve repair continues to be a major challenge in the clinic, with no easily available formula for successful treatment. Functional recovery after surgical repair is dependent upon accurate regeneration of axons to their original target tissues. Thus, a better understanding of the histological and cellular biology of nerve regeneration is required to enhance regeneration and functional recovery.
In recent years, studies have confirmed that the peripheral nerve has the potential property of selective regeneration. Based on this property, a biocompatible chitin conduit (State Intellectual Property Office of P.R.C, Patent Number: 01136314.2) was developed and applied to repairing the peripheral nerve injury by bridging the nerve injury with a small gap (2-3mm). We have confirmed its validity and superiority compared to traditional epineurial neurorrhaphy according to good micro-surgical observation, favorable histological results, excellent electrophysiological and neurological functional results on SD rats [Citation1-3] and rhesus monkeys [Citation4]. This kind of surgical method has already been put into clinical trials in China.
This rapid development provides a deeper understanding of peripheral nerve regeneration; however, the spatiotemporal regeneration process, especially the myelinated nerve regeneration process that includes axon regeneration and myelin reconstruction, is still unclear. Our nerve bridge allows for peripheral nerve regeneration and recovery of neurological function, as well as a window in which the sequential organizational, cellular, and molecular events of nerve regeneration can be observed, analyzed, and ultimately modulated. Therefore, this article will mainly concentrate on the early regeneration process of myelinated nerve fibers through this biological window. We set out to characterize the regeneration progress with a specific focus on spatial and temporal progression of axon regeneration, myelin reconstruction, and Schwann-cell migration using the chitin conduit to bridge sciatic nerve injury with a small gap (2 mm) in SD rats.
MATERIALS AND METHODS
Materials
Hollow cylindrical conduit (a de-acetyl chitin conduit invented by Beijing University People's Hospital and the Chinese Textile Academy, the State Patent No.: 01136314.2). Size: tube length 4mm, thickness 1mm, inner diameter 2mm.
Experimental Animals and Grouping
Seventy-two SD male adult rats with a body mass of 225g to 250g were allocated into 9 groups, which were normal group and postoperative 1day, 5 day, 7 day, 10 day, 14 day, 21 day, 28 day, and 60 day groups; normal sciatic nerves of the same place were also harvested, which were only used as a measure of normal nerve parameters.
Surgical Procedure
SD rats were anaesthetized with 2% pentobarbitone (0.2-0.3 ml/100 g) by intraperitoneal injection. Sciatic nerve injury models were constructed by cutting the right sciatic nerve at 5 mm above sciatic nerve forks. A sewing needle threaded the conduit from outer to inner, 1mm distance to conduit, then threaded the perilemma and conduit from inner to outer, 1mm distance to conduit, tensing two suture lines and ligaturing. Both the distal and proximal ends were all inserted to the conduit about 1mm; the distance between the ends was 2mm (). All the operations were done by the same researcher.
Morphological Analysis
At 1st, 5th, 7th, 10th, 14th, 21st, 28 th, and 60th days post-operatively, a 2-cm segment of nerve was harvested, then fixed in 4% Paraformaldehyde for 12 h at 4.
Osmium tetroxide staining and light microscope observation. Five samples of each group were postfixed in 1% osmium tetroxide for 3 days. Then the specimen was sliced into 5µm cross-sections at the distal outlet of the conduit (). The images were obtained under a Leica microscope (Leica DM 4000B, Germany) at different magnifications. Five images from different parts of each section were analyzed and five nerve sections were quantified. Finally, the average thickness of the myelin, total number of myelinated axons, and average area of the axons were evaluated by Leica Q550CW analytical system; statistical data were expressed by .
Masson staining. Three sciatic nerves of each group were formalin-fixed and paraffin-embedded, then 5-µm-thick longitudinal sections were made and subjected to Masson trichrome staining (Tiangen, China) (). The recipes produced blue connective tissues and pink cytoplasm, helping us to determine what these elongate sciatic nerves look like from a longitudinal perspective.
Immunofluorescence staining. 5-µm-thick longitudinal sections were also carried out for immunofluorescence staining (MBP for myelin sheath, NF-200 for axons, and S-100 for Schwann cells) (). Consecutive sections were incubated, respectively, with anti-MBP antibody (1:100, Chemicon, USA), anti-NF-200 antibody (1:100, Sigma, USA), and anti-S-100 antibody (1:100, Sigma, USA), and with corresponding secondary antibody (1:100, Tiangen, China). After rinsing in PBS, sections were mounted and coverslipped.
Image processing. The immunofluorescence and Masson staining images were obtained undividedly from proximal to distal ends of the longitudinal section. The micrographs were imported into Adobe Photoshop CS software (version 7.0; Adobe Systems Inc., San Jose, CA, USA) and put together in sequence. This image processing methods have been used in previous research [Citation5,Citation6]. The red and green were the true color of fluorophore excitation light; the color of yellow was a pseudo- fluorescence color made by VideoTest-FISH software (version 2.0).
RESULTS
Spatiotemporal Progression of Connective Tissues, Axons, Schwann Cells and Myelin Sheaths
We measured histological changes to characterize regeneration in three distinguishable phases.
Degeneration and matrix phase (Days 1-7). The gap was completely filled with fluid from endoneurial and epineurial vessels during the first few days. There was a gradual increase in cellularity within the chitin conduit as red blood cells were absorbed, with the proximal and distal ends linked by connective tissue about 7 days postoperatively. shows the distance between the two stumps of about 2 mm, proximal nerve fibers degraded near the injury area that recovered within a few days and started to regenerate into the conduit by the end of this period ( and ). Axon loss and myelin debris accumulated along the distal segment, with no positive staining for NF-200, S-100, and MBP by the 7th day ().
Figure 3. Histological examination on day 1 after surgery. Masson and immunofluorescence staining show no significant changes of the nerve structure; the distance between the two stumps was about 2 mm (scale range is 4 mm, n=3).
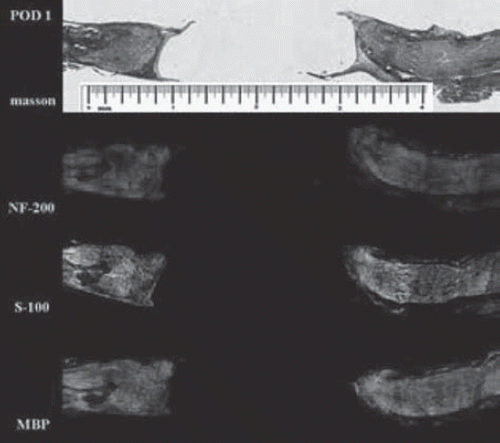
Figure 4. Histological examination on day 7 after surgery. The distal segment of nerve fibers is almost completely degenerated 7 days after surgery; the yellow arrow shows original site of proximal nerve fibers (scale range is 4 mm, n=3).
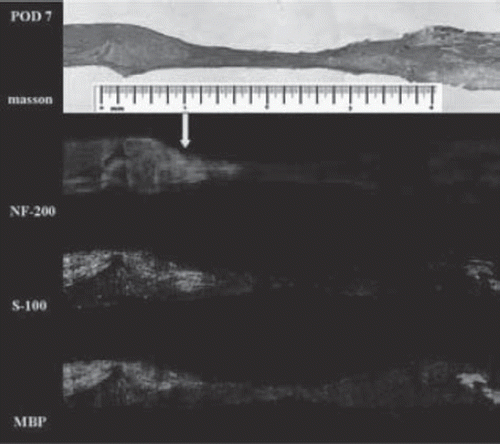
Axonal advancement and Schwann migration phase (Days 7–25). NF-200 staining demonstrates that the nerve fibers began to advance into the conduit from the proximal stump at around the 7th day (). Regenerating nerve fibers were oriented parallel to the length of the conduit and the advancing margin was conical and traveled from 1.6 mm () to 3.6 mm along the conduit () and reached the distal nerve stump about 21 days postoperatively, for an average rate of 1.4 mm/d. Schwann cells, derived from both proximal and distal nerve stumps, migrated into the lumen of the chitin conduit on the 10th day after surgery (). By day 25, the ingrowing proximal and distal Schwann cell fronts had met, and a band of S-100-positive cells spanned the entire conduit in all specimens. MBP staining showed that myelin reconstruction was slow during this period, with the proximal myelin sheath reaching the midpoint of the chamber 21 days after injury (), slightly lagging behind (about 0.2mm) proximal Schwann cells and with no myelin in the distal segments.
Figure 5. Histological examination on day 21 after surgery. The proximal axon advanced into the conduit and Schwann cells migrate into the lumen from both sides of the conduit (scale range is 4 mm, n=3).
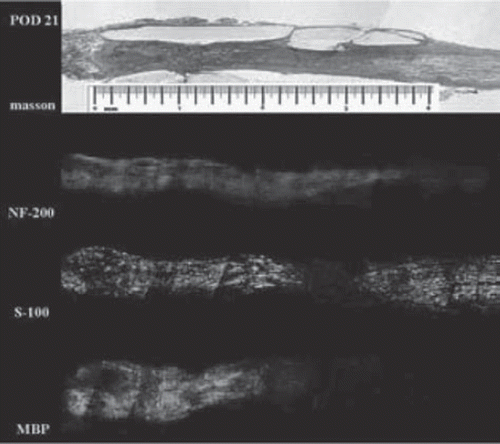
Myelination and maturation phase (Days 25 – 2 months). Masson staining demonstrates that the chitin conduit remained intact throughout the regenerative process and was encapsulated by the surrounding tissues. By day 28, axon and Schwann cells completely filled the conduit and were arranged in an orderly fashion parallel to the length of the conduit (). Distal axonal fibers myelinated quickly as soon as distal axon ends grew into the distal Schwann cells. At 28 days after surgery, the myelin sheath reached the distal outlet of the conduit (), with myelin sheaths and nerve fibers manifesting a more mature morphology 60 days postoperatively ().
The Spatial-temporal Degeneration and Reconstruction of Myelinated Nerve Fibers
To analyze myelin sheath changes quantitatively, we focused on the myelin structure at the outlet of conduit by which the Wallerian degeneration and regeneration could be clearly observed.
shows an obvious change in the pattern of myelin sheath distribution post-injury at the distal site by which the Wallerian degeneration and regeneration progress can be observed. The average normal nerve parameters were: axon area (12.33 ± 1.87 µm2), myelin thickness (1.49 ± 0.24 µm), and number of nerve fibers (8237 ± 1230), with some variability. Myelin sheath thickness peaked (1.79 ± 0.79 µm) on the 1st day and axon area peaked (13.24± 2.55µm2) on day 5 due to swelling of the myelin sheath (, ), and then decreased immediately, accompanied by the disappearance of the myelin sheath. A few small, regenerating axons were detected at 21-60 days. On day 60, the number of axons was 6452 ± 1048 and reached 78.89% ± 18.21% of the normal level; the thickness of myelin was 0.98 µm ± 0.15 µm and was 65.95% ± 9.34% of normal myelin thickness; the axon area reached 9.75 ± 1.58 µm2 and was 79.31% ± 14.23% of normal levels (, , ).
DISCUSSION
Here we observed the early spatiotemporal progress of sciatic nerve regenerating through a chitin conduit with a 2-mm gap in rats. The conduit remained intact throughout the regenerative process, allowing us an appropriate window to observe and analyze the spatial and temporal regeneration process of myelinated nerve fibers.
Nerve fibers pass through a series of degenerative processes before regeneration [Citation7,Citation8]. Proximal nerve fibers degenerated slightly [Citation9] within 10 days in this study. In contrast, as the osmium tetroxide staining results showed, distal to the injury site experienced a more severe degeneration from day 5 to 21. The distal axon and myelin sheath initially swelled and subsequently broke up into a series of spheres distally through the whole segment [Citation9].
The initial regrowth delay period in this study was calculated to about 7 days. During this period, neurons and microenvironment in the conduit lumen experienced a self-recovery process and prepared energetically for regeneration. [Citation10,Citation11,Citation12]. Successful regeneration seems to depend tightly on the appropriate timing of the cellular and molecular degeneration program [Citation13]. Schwann cells respond to loss of axons by dedifferentiation and proliferation [Citation13,Citation14]. Our study showed that Schwann cells began to regenerate from both proximal and distal sides during regeneration and bridged the gap at the 25th day after surgery. Distal Schwann cells mainly located in the inner surface of the endoneurial sheath, known as the bands of Büngner, express molecules that assist in the degeneration and repair process. Apart from growth factors, distal Schwann cells also provide structural guidance to further enhance regeneration [Citation14,Citation15].
Nerve fibers advanced through the conduit in a conic advancing margin with an average rate of 1.4 mm/d. The axonal regenerative margin moved ahead of the proximal Schwann cells, which differs from spanning of a 10-mm gap bridged with silicone tubes [Citation16]. Small amounts of Schwann cells were distributed around the regenerating axon tip; the Schwann cells and other cells, i.e. fibroblasts, endothelial cells, and perineurial cells, provide factors to move nerve regeneration forward [Citation7,Citation11,Citation17,Citation18].
Re-myelination was slower than axon regeneration, potentially because myelination requires a minimum axon caliber [Citation19,Citation20]. In the first 21 days, many proximal axons were too small for myelination but were surrounded by regenerating Schwann cells in preparation for myelination. Myelination starts when the diameter of axon increases to about 2 µm, and proximal myelination progress started [Citation21]. Furthermore, when axons with larger caliber touched distal Schwann cells, a distal fascicular-arranged Schwann could also myelinate distal axons while proximal Schwann cells myelate proximal axons. Therefore, myelination occured on the distal half of axons between day 21 and 28, with new axons bilaterally myelinated by Schwann cells from both sides. After myelination, myelin and axonal contour increasingly became arrayed and regular, but maturation was slow and prolonged.
The number and area of axons reached about 80% and the thickness of myelin was 66% of pre-injury levels by postoperative day 60 based on osmium tetroxide staining at the distal outlet. The recovery period was different in many other studies with different bridging materials and gaps [Citation22,Citation23].
From these observations, we can draw the following conclusions:
Nerve regeneration through a chitin conduit mainly occurs in three phases.
Bridging nerve injury with a small gap (2 mm) provides an appropriate window to observe peripheral nerve regeneration.
Schwann cells proliferated and migrated into the chitin conduit from the proximal and distal nerve stumps and completely filled the conduit 25 days after injury.
Axons advance into the conduit with a 7-day-delay period and bridge the nerve gap with average rate of 1.4 mm/d.
Regenerating axons were bilaterally myelinated by Schwann cells from both sides of the conduit.
Maturation proceeds at a slower rate and reached only about 80% of normal levels by day 60.
Knowledge remains insufficient to improve functional prognosis significantly, but further understanding of the sequence of events that occur during nerve regeneration will undoubtedly bring forth major advancements in our surgical and medical approaches to the management of nerve injury and repair.
Declaration of interest: The authors report no conflicts of interest. The authors alone are responsible for the content and writing of the paper.
REFERENCES
- Jiang, B.G. (2008). Dynamic observation of biomechanic properties of sciatic nerve at the suture site in rats following repairing. Artif Cells Blood Substit Immobil Biotechnol 36:45–50.
- Jiang, B.G. (2006). Small gap bridging peripheral nerve defects using biodegradable chitin conduits. Artif Cells Blood Substit Immobil Biotechnol 34:55–57.
- Jiang, B.G., Zhang, P.X. (2008). The experimental study of absorbable chitin conduit for bridging peripheral nerve defect with nerve fasciculur in rats. Artif Cells Blood Substit Immobil Biotechnol 36:360–371.
- Jiang, B.G., Zhang, P.X. (2008). The electrophysiology analysis of biological conduit sleeve bridging Rhesus monkey median nerve injury with small gap. Artif Cells Blood Substit Immobil Biotechnol 36:457–463.
- Voinesco, F., Glauser, L., Kraftsik, R., Barakat, W.I. (1998). Local administration of thyroid hormones in silicone chamber increases regeneration of rat transected sciatic nerve. Experimental Neurology 150:69–81.
- Wiklund, P., Ekstrijm, P.A.R., Edbladh, M., Tonge, D., Edstrijm, A. (1996). Protein kinase C and mouse sciatic nerve regeneration. Brain Research 715:145–154.
- Johnson, E.O., Zoubos, A.B., Soucacos, P.N. (2005). Regeneration and repair of peripheral nerves injury. Int. J. Care Injured 36S:S24–S29.
- Koike, T., Yang, Y., Suzuki, K., Zheng, X.X. (2008). Axon & dendrite degeneration: Its mechanisms and protective experimental paradigms. Neurochemistry International 52:751–760.
- Cheepudomwit, T., Guzelsu, E., Zhou, C., Griffin, J.W., Hoke, A. (2008). Comparison of cytokine expression profile during Wallerian degeneration of myelinated and unmyelinated peripheral axons. Neurosci Lett. 430:230–235.
- Takagi, T., Nakamura, M., Yamada, M., Hikishima, K., Momoshima, S., Fujiyoshi, K., Shibata, S., Okano, H.J., Toyama, Y., Okano, H. (2009). Visualization of peripheral nerve degeneration and regeneration: Monitoring with diffusion tensor tractography. Neuro Image 44:884–892.
- Brandt, J., Dahlin, L.B., Kanje, M., Lundborg, G. (1999). Spatiotemporal progress of nerve regeneration in a tendon autograft used for bridging a peripheral nerve defect. Experimental Neurology 160:386–393.
- Makwana, M., Raivich, G. (2005). Molecular mechanisms in successful peripheral regeneration. FEBS Journal 272:2628–2638.
- Benavides, E., Alvarez, J. (1998). Peripheral axons of Wlds mice, which regenerate after a delay of several weeks, do so readily when transcription is inhibited in the distal stump. Neurosci Lett. 258:77–80.
- Sondell, M., Lundborg, G., Kanje, M. (1998). Regeneration of the rat sciatic nerve into allografts made acellular through chemical extraction. Brain Research 795:44–54.
- Frostick, S.P., Yin, Q. (1998). Schwann cells, neurotrophic factors, and peripheral nerve regeneration. Microsurgery 18:397–405.
- Akassoglou, K., Akpinar, P., Murray, S., Strickland, S. (2003). Fibrin is a regulator of Schwann cell migration after sciatic nerve injury in mice. Neurosci Lett. 338:185–188.
- Radtke, C., Akiyama, Y., Lankford, K.L., Vogt, P.M., Krause, D.S., Kocsis, J.D. (2005). Integration of engrafted Schwann cells into injured peripheral nerve: axonal association and nodal formation on regenerated axons. Neurosci Lett. 387:85–89.
- Fukuyamal, R., Takeda, H., Fushiki, S., Yamamotol, T. (1998). Muramyl dipeptide injected into crushed sciatic nerve, activates macrophages and promotes recovery of walking locomotion in rats. Restorative Neurology and Neuroscience 13:213–219.
- Chamberlain, L.J., Yannas, I.V., Arrizabalaga, A. (1998). Early peripheral nerve healing in collagen and silicone tube implants: Myobroblasts and the cellular response. Biomaterials 19:1393–1403.
- Garbay, B., Heape, A.M., Sargueil, F., Cassagnea, C. (2000). Myelin synthesis in the peripheral nervous system. Neurobiology 61:267–304.
- Peles, E., Salzer, J.L. (2000). Molecular domains of myelinated axons. Current Opinion in Neurobiology 10:558–565.
- Nave, K.A., Salzer, J.L. (2006). Axonal regulation of myelination by neuregulin. Current Opinion in Neurobiology 16:492–500.
- Chen, Y.S., Hsieh, C.L., Tsai, C.C., Chen, T.H., Cheng, W.C., Hu, C.L., Yao, C.H. (2000). Peripheral nerve regeneration using silicone rubber chambers filled with collagen, laminin and bronectin. Biomaterials 21:1541–1547.