Abstract
Numerous manufacturing techniques for autogenous fibrin glue used as scaffold material have been described. As there is no consensus regarding the influence of chemical additives on cell biology, it was the aim of this study to establish a method for manufacturing autologous fibrin glue without any additives. The serum part was separated from whole blood. After fibrinogen precipitation, centrifugation was performed to obtain the fibrinogen pellet. Various experimental series were run to examine influences of various temperatures or substituting centrifugation for sedimentation. The method as described here is effective, simple, and performed without any additives, which could potentially influence cell biology.
INTRODUCTION
Generation of autologous cartilage for reconstructive surgery of congenital or acquired cartilage defects is a key issue in tissue engineering research. The use of autogenous tissue components reduces the risk of rejection and the transmission of diseases.
Tissue engineering research is complex and involves both cellular and molecular biology. In addition to the technique of using autologous chondrocytes, mesenchymal stem cells from bone marrow or adipose tissue, with their ability to differentiate into various cell phenotypes, appears to be an alternative cell source for cartilage tissue engineering [Citation7, Citation13, Citation26, Citation35]. Furthermore, tissue engineering research also involves the material and mechanical engineering of suitable cell carrier matrices. Proliferated cells, either autologous chondrocytes or mesenchymal stem cells, are added to three-dimensional scaffolds to form autologous, implantable constructs. These scaffolds require adequate mechanical stability, should be biodegradable and allow cell adhesion [Citation1]. A wide variety of both synthetic and natural polymers exists. Besides macroporous scaffolds [Citation10], hydrogels like alginate, collagen or fibrin are also used as carrier matrices [Citation11, Citation12, Citation31, Citation34]. Hydrogels are very promising scaffolds due to their favorable properties as compared to soft tissue. In addition, they are also characterized by their efficient transport of water and nutrients due to their high water content, and thus may be injected as liquid cell carriers [Citation1].
Numerous techniques have been described for manufacturing autogenous fibrin glue that are designed to avoid disease transmission. However, cryoprecipitation has not proven to be practical for harvesting fibrinogen from small volumes of blood. Alternative precipitation techniques have been applied to obtain autologous fibrin tissue adhesives using ammonium sulfate, ethanol, or polyethylene glycol [Citation2, Citation23]. All of these techniques guarantee that the tissue adhesive produced does not provoke immunological reactions, toxic effects, or increase the risk of disease transmission in clinical use. Recently, dose-dependent genotoxic and cytotoxic effects of compounds released from open-pored polyurethane scaffolds for cartilage tissue engineering could be determined [Citation22]. However, there is no consensus regarding the influence of carrier matrices, especially manufactured autologous fibrin, on cell proliferation or differentiation in tissue engineering research. There are various reports on the lack of influence of fibrin glue on cell differentiation, proliferation, and cell ingrowth [Citation4, Citation28], but others have also described the inhibitory effects of fibrin [Citation5, Citation24]. Recently, Haasper et al. [Citation15] analyzed the in vitro effect of fibrin glue on the proliferation and differentiation of bone marrow stromal cells seeded on a three-dimensional matrix. They found no differences with respect to cell differentiation, but observed decreased cell proliferation using fibrin glue after a one-week observation period. Catelas et al. [Citation6] demonstrated the influence of the fibrinogen complex/thrombin ratio on the osteogenic differentiation of bone-marrow-derived stem cells (BMSC). However, it is not clear whether there is any influence caused by chemical agents such as ethanol, polyethylene glycol (PEG), or ammonium sulphate (which are used for fibrin gel preparation) on the morphology, proliferation, or differentiation of cells seeded in fibrin as a potential scaffold.
Thus, it was the aim of the present study to develop a method for manufacturing autologous fibrin glue without any additives for use in cartilage tissue engineering in an attempt to avoid adverse effects on cell morphology, proliferation, and differentiation. Further important criteria were obtaining an adequate amount of fibrin glue using an acceptable volume of patients' blood, small material consumption, uncomplicated handling, and cost-effectiveness.
MATERIALS AND METHODS
Patients and Blood Samples
40 ml of whole blood was taken from 28 healthy donors who underwent routine operative procedures, e.g., nasal septal plasty, in the ENT Department of the University of Regensburg, Germany. The blood was heparinized in a commercial, disposable 20 ml syringe with 2 ml heparin (5000 IE/ml) and drawn into sterile 50ml PP tubes (Cellstar®, Frickenhausen, Germany). All the patients had given informed consent and the study was approved by the local ethics committee of the University of Regensburg Medical Faculty.
Sedimentation and Centrifugation Procedures
The isolation procedure itself consisted of three steps (). The first isolation step, separation of the serum and cruor parts of the blood, involved either a freezing or a centrifugation procedure. The second step, the fibrinogen precipitation in the remaining serum after discarding the cruor, was again a freezing procedure. Afterwards, to obtain the fibrinogen pellet in the third step, centrifugation of the serum part followed for 15 minutes at 400×g. This third step was performed identically in the various test series.
Figure 1. Fibrinogen isolation consists of three steps: separation of cruor and serum, fibrinogen precipitation, and preparation of the fibrinogen pellet by centrifugation.
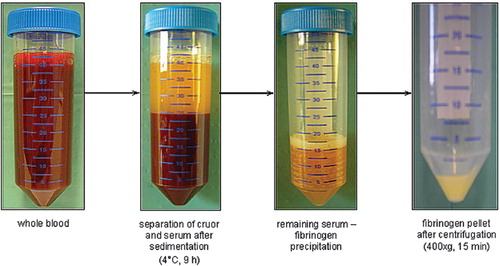
In the first test series (), whole blood samples (n=14) were stored at 4°C for 9 hours to achieve the adequate separation of cruor and the upper serum part. After an almost complete separation of each layer, the supernatant was carefully removed using a disposable pipette, without touching the cruor, and transferred into another tube. Subsequent precipitation of the fibrinogen was obtained by freezing the transferred supernatant at temperatures of −20°C for 18 hours, −86°C for at least 12 hours, and −196°C in liquid nitrogen for 10 minutes. Next, the frozen serum was thawed slowly at 4°C for four hours. Finally, to obtain the fibrinogen pellet, centrifugation for 15 minutes at 400×g followed and the supernatant was discarded carefully. The efficacy of fibrinogen preparation and the further processability of the fibrinogen pellet obtained using these different temperatures for precipitation was compared.
In a second series (), the possibility of reducing the time required for the gravity-sedimentation procedure (first isolation step) was examined. The efficacy of fibrinogen preparation using the procedure of freezing the whole blood samples at 4°C for 9 hours (n=7 per 20 ml) vs. centrifugation at 200×g for 10 minutes (n=7 per 20 ml) to separate the serum part from the cruor were compared. The second and third isolation steps were performed as described above: the freezing temperature and time used for the precipitation procedure (second isolation step) was −86°C for 12 hours. Final centrifugation was performed for 15 minutes at 400×g to obtain the fibrinogen pellet (third isolation step).
Determination of Fibrinogen Content
Determining the fibrinogen content of whole blood samples, as well as the remaining serum part and cruor, was performed in the central laboratory of an institution using Multifibren U (Dade Behring, Marburg, Germany), an automatic standardized procedure. The principle behind this technique is a modification of the Clauss method. Citrated plasma is brought to coagulation with a large excess of thrombin. Here, the coagulation time depends largely on the fibrinogen content of the sample.
Preparation of Fibrin Glue
For manufacturing the fibrin sealant, the fibrinogen pellet was resuspended with 500μl of aprotinin (Trasylol®, Bayer, Leverkusen, Germany) and dissolved homogenously. Afterwards, fibrinogen solution was drawn into one syringe of the duplojet application system and the other was filled with a thrombin solution (Baxter, Unterschleiβheim, Germany), which is mandatory for the formation of biopolymer fibrin by cleavage of the monomer fibrinogen to exposed polymerization sites. The cleavage of fibrinopeptide A and B from the fibrinogen generates the fibrin monomer, which has a strong tendency to self-associate and become insoluble. To obtain a 50 IU solution, thrombin (500 IU) was resuspended in a CaCl2 solution (40mmol/L).
The same volumes of both fibrin glue components were mixed using the duplojet application system (Baxter, Unterschleiβheim, Germany) and filled into chamber slides.
After polymerization, the fibrin glue constructs were incubated in Dulbecco's modified Eagle medium (DMEM; Sigma, Taufkirchen, Germany) under cell culture conditions (5% CO2, 37°C) to analyze form stability and size of the constructs after two weeks of incubation.
RESULTS
Fibrinogen Yield: Gravity-sedimentation Using Different Temperatures
In the first test series, there were significant differences found with respect to the fibrinogen wet weight obtained when comparing the various freezing temperatures −20°C, −86°C, and −196 °C (). However, additional distinct differences were found regarding the further processability of the fibrinogen pellets. The fibrinogen pellets generated by precipitation at −20°C and −196°C could not be resuspended with aprotinin and thus could no longer be used. But the pellet obtained by freezing the serum part at −86°C for 12 hours could be easily dissolved in aprotinin solution (Trasylol®). Consequently, this temperature and time (−86°C, 12 hours) combination was defined as standard procedure for the subsequent series.
Fibrinogen Yield: Gravity-sedimentation vs. Centrifugation
In the second series, the fibrinogen yield after accelerating the separation procedure using centrifugation for 10 minutes at 200xg was determined. Afterwards, this was compared with the results using sedimentation at 4°C for 9 hours. The fibrinogen amount in the remaining serum part after the final centrifugation (third isolation step) and the cruor of all samples was lower than 0.6 mg/ml, which is the minimum amount that can be measured by the automatic quantification device.
241 mg fibrinogen (wet weight) could be isolated out of 20 ml of patient whole blood using centrifugation and 309 mg fibrinogen (wet weight) using the sedimentation procedure in the freezer as the first step of fibrinogen isolation (). The fibrinogen concentration that could be determined in the whole blood samples before the isolation procedure was 2.88 mg/ml on average. The volume of the separated serum part, which was continued processing, was 10.3 ml and the volume of the cruor was 9.9 ml on average. Furthermore, it was noticeably much harder to separate the cruor and serum part completely after 10 minutes centrifugation than after 9 hours sedimentation in the freezer (4°C).
Figure 5. Fibrinogen yield (wet weight in mg) after the first isolation step using centrifugation at 200×g for 10 minutes vs. sedimentation at 4°C for 9 hours; 241 mg fibrinogen (wet weight) could be isolated out of 20 ml of patient whole blood using centrifugation and 309 mg fibrinogen (wet weight) using the sedimentation procedure (data represent the average + SD).
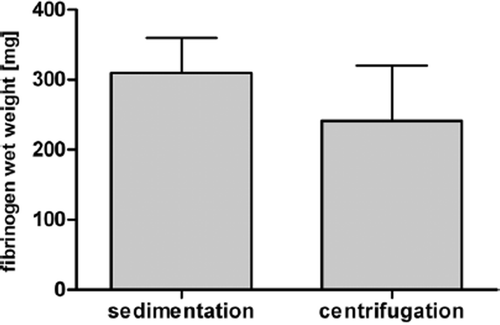
The fabricated fibrin glue constructs maintained their form stability, size, and consistency under cell culture conditions for the two weeks of observation ().
DISCUSSION
Fibrin glue is used as a sealant in surgical practice in order to reduce blood loss during and after surgery [Citation3], e.g., in tonsillectomies, adenectomies and sinus surgery [Citation18, Citation29, Citation32], to avoid leakages [Citation25], to support revascularization after microvascular surgery [Citation20], and to prevent seroma and postoperative bleeding after soft tissue dissection, e.g. salivary gland surgery [Citation8].
Efficient manufacturing of autologous fibrin glue from patient blood should be fast and cost-effective. Commercial products are derived from pooled donors with an inherent risk of pathogen contamination. Complex processes like heating, ultraviolet irradiation, and pasteurization, which are applied to reduce this risk, add to the cost of these commercial tissue adhesives [Citation2, Citation19, Citation32]. Although numerous precautions are taken to avoid transmission of disease, the risk cannot be eliminated completely. There have been reports of one case of HIV transmission and three cases of symptomatic parvovirus B19 transmission after fibrin glue application [Citation17, Citation32].
In tissue engineering research, fibrin alone or as part of a composite is used as a cell carrier matrix for the generation of, e.g., cartilage, bone, adipose or other tissues [Citation1, Citation11, Citation12, Citation14, Citation30]. Tissue engineering carrier matrices have to provide a suitable environment, which allows tissue development with a specific matrix production and enables cell migration, even cell distribution, proliferation, and differentiation. In addition, matrices have to meet defined criteria regarding mechanical stability. Fibrin hydrogel has the disadvantages of shrinkage, increasing instability, and rapid degradation [Citation1, Citation11] over time in vitro and in vivo. This could pose a serious problem for shaped scaffolds. However, by varying the concentrations of fibrinogen as well as the Ca2+ and pH-value, fibrin gels can be optimized with respect to mechanical properties and long-term stability for in vitro cartilage engineering [Citation11]. Furthermore, cross-linking and clot strength can be improved by adding factor VIII [Citation9]. The preparation method described here can be performed without any additional agents and the fibrin gel proved stable in form and size under cell culture conditions for the two-week observation period.
Over the past several years, a variety of techniques using patients' own blood to produce autologous fibrin glue have been developed. Cryoprecipitation, the most common method for isolating fibrinogen from human blood, is not practical for harvesting fibrinogen from small blood volumes and, furthermore, bonding power of cryoprecipitated fibrinogen is not predictable [Citation2, Citation23]. However, automated or semi-automated, customizable systems like the CryoSeal FS System (ThermaGenesis Corp, Rancho Cordova, USA), which uses the cryoprecipitation technique, are reported to require short times and small volumes of plasma for the production of autologous fibrin sealant components [Citation16].
Precipitation of fibrinogen is also possible using various chemical agents such as ethanol, polyethylene glycol (PEG), or ammonium sulfate [Citation2, Citation23]. These methods require shorter times and smaller volumes of patient blood than cryoprecipitation. Ethanol precipitation was shown to be effective and inexpensive for the preparation of autologous fibrin glue [Citation21, Citation33], plus the fibrin tissue adhesive obtained did not induce any toxic or inflammatory effects in an animal model [Citation23]. However, increased levels of ethanol in the fibrinogen concentrate can influence the clotting ability of the fibrinogen, reduce the factor XIII activity and the sealant tensile strength [Citation2].
Others have described the preparation of autologous fibrinogen using protamine, a cationic protein that is usually used to restore hemostasis in heparinized patients. Precipitation by protamine has been described as a rapid method with recovery of almost 100% of the plasma fibrinogen [Citation2]. All of these techniques guarantee that the produced tissue adhesive does not provoke immunological reactions or transmit diseases. However, there is no consensus regarding the influence of carrier matrices on cell proliferation or differentiation [Citation4, Citation5, Citation6, Citation15, Citation22, Citation24, Citation28]. Several authors [Citation4, Citation28] reported no influence of the fibrin glue used as a scaffold on cell differentiation, proliferation, cell ingrowth of chondrocytes and bone-marrow derived cells. Others, however, determined inhibitory effects of fibrin glue used for tissue engineering approaches [Citation5, Citation24]. Catelas et al. [Citation6] demonstrated that bone-marrow-derived stem cells (BMSC) affected morphology, proliferation, and osteogenic differentiation in fibrin gels depending on the fibrinogen complex (FC)/thrombin ratio. BMSCs appeared to undergo osteogenic differentiation when seeded in Tisseel fibrin sealant containing a high FC concentration, but they did not fully differentiate into mature osteoblasts. Recently, Haasper et al. [Citation15] analyzed the in vitro effect of fibrin glue on the proliferation and differentiation of bone-marrow-derived stem cells seeded on a three-dimensional matrix. They found no differences regarding cell differentiation, but cell proliferation decreased using the fibrin glue after one week observation.
Moreover, it is not clear whether there is any influence of the chemical agents used for fibrin gel preparation, like ethanol, polyethylene glycol (PEG) or ammonium sulfate, on the morphology, proliferation, or differentiation of cells seeded in fibrin glue. All of these potentially influential factors can be excluded with the method described here. The acceleration of the preparation procedure using centrifugation instead of sedimentation is a conceivable but not equally effective alternative since it is much harder to separate the cruor and serum part completely after 10 minutes centrifugation than after 9 hours sedimentation in the freezer.
Furthermore, a portion of the fibrinogen amount in the whole blood samples was apparently lost during the procedures since, on average, 275 mg fibrinogen (wet weight) from a total of 20 ml whole blood could be obtained, while a fibrinogen concentration of 2.88 mg/ml on average could be determined in the whole blood samples. However, the volume of the separated serum part after the sedimentation step, which could be continued processing, was only about 10 ml. We obtained about 1 ml fibrin glue after addition of 50 IU thrombin at a ratio of 1:1, a volume which is comparable with the results of preparation procedures described by others [Citation16, Citation21, Citation23, Citation27, Citation33]. Thorn et al. [Citation27], e.g., obtained 8 ml autologous fibrin glue from 200 ml platelet-rich plasma by ethanol precipitation and centrifugation. Another method of manufacturing autologous fibrin glue based on ethanol was described by Kjaergard et al. [Citation21]. They used 44 ml and 88 ml blood for the isolation procedure and obtained volumes of 3.3 ml and 6.4 ml tissue adhesive, respectively.
The preparation of autologous fibrin gels provides the possibility of using human chondrocytes or autologous mesenchymal stem cells seeded on a self-produced scaffold using blood from a single donor for tissue-engineering experiments. This approach will undoubtedly prove important in future clinical applications of autologous cell-based therapeutic strategies.
In summary, this study presents an uncomplicated, convenient, and cost-effective method for the preparation of autologous fibrin gel by centrifugation. Such gels can be used as scaffolds for cartilage tissue engineering. The amount of tissue adhesive obtained and its stability under cell culture conditions rival commercial glue or other established procedures. However, the major advantage of the described method is that it is not associated with any potential influence of additional agents on cell morphology, proliferation, or differentiation.
Declaration of interest: The authors report no conflicts of interest. The authors alone are responsible for the content and writing of the paper.
REFERENCES
- Ahmed TA, Dare EV, Hincke M (2008). Fibrin: a versatile scaffold for tissue engineering applications. Tissue Eng Part B Rev. 14:199–215.
- Alston, S.M., Solen, K.A., Broderick, A.H., Sukavaneshvar, S., Mohammad, S.F. (2007). New method to prepare autologous fibrin glue on demand. Transl Res. 149:187–195.
- Canonico, S. (2003). The use of human fibrin glue in the surgical operations. Acta Biomed. 74Suppl:21–25.
- Cardon, A., Chakfé, N., Thaveau, F., Gagnon, E., Hartung, O., Aillet, S., Kerdiles, Y., Dion, Y.M., Kretz, J.G., Doillon, C.J. (2000). Sealing of polyester prostheses with autologous fibrin glue and bone marrow. Ann Vasc Surg. 14:543–552.
- Carmagnola, D., Berglundh, T., Lindhe, J. (2002). The effect of a fibrin glue on the integration of Bio-Oss with bone tissue. A experimental study in labrador dogs. J Clin Periodontol. 29:377–383.
- Catelas, I., Sese, N., Wu, B.M., Dunn, J.C., Helgerson, S., Tawil, B. (2006). Human mesenchymal stem cell proliferation and osteogenic differentiation in fibrin gels in vitro. Tissue Eng. 12:2385–2396.
- De Ugarte, D.A., Morizono, K., Elbarbary, A., Alfonso, Z., Zuk, P.A., Zhu, M., Dragoo, J.L., Ashjian, P., Thomas, B., Benhaim, P., Chen, I., Fraser, J., Hedrick, M.H. (2003). Comparison of multi-lineage cells from human adipose tissue and bone marrow. Cells Tissues Organs. 174:101–109.
- Depondt, J., Koka, V.N., Nasser, T., Portier, F., Guedon, C., Barry, B., Gehanno, P. (1996). Use of fibrin glue in parotidectomy closure. Laryngoscope. 106:784–787.
- Dickneite, G., Metzner, H.J., Kroez, M., Hein, B., Nicolay, U. (2002). The importance of factor XIII as a component of fibrin sealants. J Surg Res. 107:186–195.
- Emin, N., Koç, A., Durkut, S., Elçin, A.E., Elçin, Y.M. (2008). Engineering of rat articular cartilage on porous sponges: effects of tgf-beta 1 and microgravity bioreactor culture. Artif Cells Blood Substit Immobil Biotechnol. 36:123–137.
- Eyrich, D., Brandl, F., Appel, B., Wiese, H., Maier, G., Wenzel, M., Staudenmaier, R., Goepferich, A., Blunk, T. (2007). Long-term stable fibrin gels for cartilage engineering. Biomaterials. 28:55–65.
- Eyrich, D., Wiese, H., Maier, G., Skodacek, D., Appel, B., Sarhan, H., Tessmar, J., Staudenmaier, R., Wenzel, M.M., Goepferich, A., Blunk, T. (2007). In vitro and in vivo cartilage engineering using a combination of chondrocyte-seeded long-term stable fibrin gels and polycaprolactone-based polyurethane scaffolds. Tissue Eng. 13:2207–2218.
- Gimble, J.M., Katz, A.J., Bunnell, B.A. (2007). Adipose-derived stem cells for regenerative medicine. Circ Res. 100:1249–1260.
- Gorodetsky, R. (2008). The use of fibrin based matrices and fibrin microbeads (FMB) for cell based tissue regeneration. Expert Opin Biol Ther. 8:1831–1846.
- Haasper, C., Breitbart, A., Hankemeier, S., Wehmeier, M., Hesse, E., Citak, M., Krettek, C., Zeichen, J., Jagodzinski, M. (2008). Influence of fibrin glue on proliferation and differentiation of human bone marrow stromal cells seeded on a biologic 3-dimensional matrix. Technol Health Care. 16:93–101.
- Haisch, A., Loch, A., David, J., Pruss, A., Hansen, R., Sittinger, M. (2000). Preparation of a pure autologous biodegradable fibrin matrix for tissue engineering. Med Biol Eng Comput. 38:686–689.
- Hino, M., Ishiko, O., Honda, K.I., Yamane, T., Ohta, K., Takubo, T., Tatsumi, N. (2000). Transmission of symptomatic parvovirus B19 infection by fibrin sealant used during surgery. Br J Haematol. 108:194–195.
- Jameson, M., Gross, C.W., Kountakis, S.E. (2006). FloSeal use in endoscopic sinus surgery: effect on postoperative bleeding and synechiae formation. Am J Otolaryngol. 27:86–90.
- Joch, C. (2003). The safety of fibrin sealant. Cardiovasc Surg. 11 Suppl:23–28.
- Jorgensen, S., Bascom, D.A., Partsafas, A., Wax, M.K. (2003). The effect of 2 sealants (FloSeal and Tisseel) on fasciocutaneous flap revascularization. Arch Facial Plast Surg. 5:399–402.
- Kjaergård, H.K., Weis-Fogh, U.S., Sørensen, H., Thiis, J.J., Rygg, I. (1992). Autologous fibrin glue–preparation and clinical use. Ugeskr Laeger. 154:1554–1556.
- Lotz, A.S., Havla, J.B., Richter, E., Frölich, K., Staudenmaier, R., Hagen, R., Kleinsasser, N.H. (2009). Cytotoxic and genotoxic effets of matrices for cartilage tissue engineering. Toxicol Lett. 190:128–133.
- Park, J.J., Cintron, J.R., Siedentop, K.H., Orsay, C.P., Pearl, R.K., Nelson, R.L., Abcarian, H. (1999). Technical manual for manufacturing autologous fibrin tissue adhesive. Dis Colon Rectum. 42:1334–1338.
- Schmal, H., Mehlhorn, A.T., Kurze, C., Zwingmann, J., Niemeyer, P., Finkenzeller, G., Dauner, M., Südkamp, N.P., Köstler, W. (2008). In vitro study on the influence of fibrin in cartilage constructs based on PGA fleece materials. Orthopäde. 37:424–434.
- Stojanović, M., Jeremic, M., Stojanović, P., Stojiljković, M., Gmijović, D., Stanojković, Z., Savić, V., Djordjević, V., Cvetković, Z., Kostov, M., Colović, R. (2002). Use of fibrin glue in the prevention of leakage in pancreatico-jejunal anastomoses. Acta Chir Iugosl. 49:39–45.
- Strem, B.M., Hicok, K.C., Zhu, M., Wulur, I., Alfonso, Z., Schreiber, R.E., Fraser, J.K., Hedrick, M.H. (2005). Multipotential differentiation of adipose tissue-derived stem cells. Keio J Med. 54:132–141.
- Thorn, J.J., Sørensen, H., Weis-Fogh, U., Andersen, M. (2004). Autologous fibrin glue with growth factors in reconstructive maxillofacial surgery. Int J Oral Maxillofac Surg. 33:95–100.
- Ting, V., Sims, C.D., Brecht, L.E., McCarthy, J.G., Kasabian, A.K., Connelly, P.R., Elisseeff, J., Gittes, G.K., Longaker, M.T. (1998). In vitro prefabrication of human cartilage shapes using fibrin glue and human chondrocytes. Ann Plast Surg. 40:413–420.
- Vaiman, M., Eviatar, E., Shlamkovich, N., Segal, S. (2003). Effect of modern fibrin glue on bleeding after tonsillectomy and adenoidectomy. Ann Otol Rhinol Laryngol. 112:410–414.
- Vinatier, C., Gauthier, O., Masson, M., Malard, O., Moreau, A., Fellah, B.H., Bilban, M., Spaethe, R., Daculsi, G., Guicheux, J. (2009). Nasal chondrocytes and fibrin sealant for cartilage tissue engineering. J Biomed Mater Res A. 89:176–185.
- Yan, J., Qi, N., Zhang, Q. (2007). Rabbit articular chondrocytes seeded on collagen-chitosan-GAG scaffold for cartilage tissue engineering in vivo. Artif Cells Blood Substit Immobil Biotechnol. 35:333–344.
- Yoo, J., Chandarana, S., Cosby, R. (2008). Clinical application of tissue adhesives in soft-tissue surgery of the head and neck. Curr Opin Otolaryngol Head Neck Surg. 16:312–317.
- Weis-Fogh, U.S. (1988). Fibrinogen prepared from small blood samples for autologous use in a tissue adhesive system. Eur Surg Res. 20:381–389.
- Zhang, L., Tang, P., Wang, Y., Zhang, W., Xu, M., Han, J., Wang, Z. (2009). Comparisons of collagen gel and collagen matrix as cell growth microenvironment: the physical-chemical properties. Artif Cells Blood Substit Immobil Biotechnol. 37:166–172.
- Zuk, P.A., Zhu, M., Mizuno, H., Huang, J., Futrell, J.W., Katz, A.J., Benhaim, P., Lorenz, H.P., Hedrick, M.H. (2001). Multilineage cells from human adipose tissue. Tissue Eng. 7:211–228.