INTRODUCTION
As is known, paclitaxel (PTX), one of the most effective anticancer drugs on the market today, has been demonstrated in clinical trials against a wide variety of tumors, including ovarian carcinoma, breast cancer, head and neck cancers, and non-small cell lung cancer [Citation1–4]. However, its low aqueous solubility is one of the biggest shortcomings of PTX. The current clinical dosage form of PTX is dissolved in a mixture of Cremophor® EL (poly-oxyethylated caster oil) and ethanol (50:50, v/v) and needs to be diluted before injection. However, Cremophor® EL has been associated with serious side-effects and leads to hypersensitivity, nephrotoxicity, and neurotoxicity in many patients [Citation3]. In order to increase the therapeutic efficiency and reduce the side-effects caused by these vehicles, much effort has been devoted to improving the aqueous solubility of PTX without using Cremophor® EL [Citation5].
Liposomal formulations serve as one of the promising approaches since the association of drugs with lipid carriers results in a dramatic improvement of the pharmacokinetics of the drug, resulting in reduced toxicities and improved therapeutic efficacies [Citation6–9]. The extent of liposome accumulation in the tumor interstitium is, amongst others, correlated to the blood circulation time, which can be significantly prolonged by the incorporation of polyethylene glycol (PEG) grafted lipids in the bilayer [Citation10–12]. However, PEG prevents normal interaction of the carrier with cells and other destabilizing factors, or when the drug carrier is intended for intracellular penetration. To this problem there are several approaches, including the use of pH-sensitive pharmaceutical nanocarriers based on the fact that many pathological sites, including tumors, demonstrate acidification. For example, A.A. Kale and V.P. Torchilin developed PEGylated TATp-modified pH-sensitive liposomes comprising a cell-penetrating function, TATp, and a protective pH-sensitive coating formed by PEG-Hz-PE, with lowered pH values and removal of PEG coat, which should result in the enhanced internalization of the nano-carriers into target cells [Citation13]. Junhwa Shin et al. developed a pH-sensitive PEG-PE modified liposomes based on acid-labile vinyl ether with lowered pH values and removal of PEG coat [Citation14].
In our previous study, novel mPEG-Hz-Chol conjugate was synthesized with the hydrazone bond and the physicochemical characterization was tested in vitro pH-dependent degradation. The particle size, transmission electron microscopy (TEM), and in vitro releasing capacity of mPEG2000-Hz-Chol modified liposomes were evaluated. Cytotoxicity assay and cellular uptake of PTX loaded mPEG2000-Hz-Chol liposomes were measured.
In the present study, we report on the development of a novel mPEG-Hz-Chol conjugate modified pH-sensitive liposomes containing PTX. The pH-sensitive liposomes were evaluated in terms of stability, pharmacokinetics, tissue distribution, and anti-tumor efficacy. shows pH-sensitive liposomes following the change of pH value. shows the structure of mPEG-Hz-Chol.
MATERIALS AND METHODS
Materials
Soybean phosphatidylcholine (S100PC) was purchased from Dongshang Co. Ltd. (Shanghai, China). Cholesterol (Chol) was obtained from China Medicine Shanghai Chemical Reagent Corporation (Shanghai, China). PTX was supplied by Taihua Natural Plant Pharmaceutical Co. Ltd. (China). The pH-sensitive conjugate mPEG-Hz-chol was synthesized in our lab. All other chemicals were analytical grade.
Sprague-Dawley (SD) rats and Kunming mice were obtained from the Laboratory Animal Center of Nantong University. BALB/c mice were obtained from Yangzhou University. All the animals were pathogen-free and allowed to access food and water freely. All the animal experiments were in accordance with Guide for the Care and Use of Laboratory Animals published by the National Institutes of Health.
Preparation of Liposomes
The plain liposomes composed of S100PC/chol/PTX (90:10:3) and the pH-sensitive liposomes composed of S100PC/chol/mPEG2000-Hz-Chol/PTX (90:10:3:3) were prepared by the thin-film hydration method. Briefly, the hydrophobic excipients, PTX, cholesterol and lipids were dissolved in chloroform and transferred into a suitable conical flask. The flask was evaporated under reduced pressure. The dry lipid formed was hydrated with phosphate buffer saline (pH 7.4). The liposomal suspension was filtered through 0.2 mm polycarbonate filters and stored at 4°C until use.
Stability
Due to its affecting the tissue distribution and clearance, the particle size plays an important role in the alternation of pharmacokinetics. However, it is also important to study the stability of the liposomes formulation before the research of pharmacokinetics and tissue distribution. The stability of the liposomes before or after freeze-drying was observed by investigating the aggregation of liposomes in PBS, or PBS with 50% FBS at 37°C. The change of the mean particle size was measured before and after 1 h, 12 h, 24 h incubation [Citation15]. The particle sizes of the liposomes were measured with a particle sizer 3000HS (Malvern Instruments, UK) and analyzed by the Zetasizer 3000H (Malvern software).
Pharmacokinetic Studies
SD rats (220–260 g) were chosen to examine the pharmacokinetics of mPEG2000-Hz-Chol modified liposomes containing PTX (PTX-ML) and unmodified plain liposomes containing PTX (PTX-PL) as the control. Rats were randomly divided into the following three groups (n=6): (1) PTX-ML (7 mg/kg); (2) PTX-PL (7 mg/kg); and (3) Taxol® (7 mg/kg). Drugs were intravenously administrated through the tail vein. The blood samples (0.5 mL) were collected from the plexus venous in the eyeground at 5 min, 15 min, 30 min, 1 h, 2 h, 4 h, 6 h, 8 h, and 12 h. The plasma were obtained by centrifugation at 5000 rpm for 10 min and stored at −20°C. 140 μL of blood sample, 10 μL of internal standard (Diazepard in methanol), and 290 μL of acetonitrile were added into a protein precipitation tube. The mixture was vortexed for 5min and centrifugated at 8000 rpm for 10 min. 20 μL of the solution were injected onto the HPLC system. The HPLC was equipped with a reverse-phase column (4.6mm×250 mm, Hanbon, Jiangsu, China) at 35°C and with a UV spectrophotometer (Agilent Technologies, Palo Alto, CA, USA). The mobile phase was a mixture of methanol and water (72:28 v/v). The samples were delivered at a flow rate of 1.0 mL/min and detected at a wavelength of 227 nm. Drug and Statistics for Windows (DAS ver 2.0) was utilized to analyze the pharmacokinetic parameters of the area under the plasma concentration time curve (AUC0-∞), the apparent volume of distribution (Vd), total body clearance (CL), elimination half-life (t1/2β) and mean residence time (MRT) of PTX for each formulation [Citation16–18].
Tissue Distribution Studies
To assess the effect of pH-sensitive liposomes on tissue distribution of PTX, Kunming mice (18–22 g) were used in three formulations (Taxol®, PTX-ML, and PTX-PL) after intravenous administration via caudal vein at the dose of 10 mg/kg bodyweight. After 0.25, 0.5, 1, 2, 4, 6, 8, 12, and 24 h of injection, blood samples were collected from the eyes of six mice in each group, after which the mice were sacrificed by cervical dislocation. Then the blood was immediately treated as described in Section 2.4. The organs (heart, liver, spleen, lung, and kidney) were removed and washed twice with physiological solution (0.9% NaCl), weighed, and homogenized. 150 μL of homogenate, 10 μL of internal standard (Diazepard in methanol), and 290 μL of acetonitrile were added. The mixture was vortexed for 5 min and centrifugated at 15,000 rpm for 10 min. 20 μL of the supernatant was injected onto the HPLC system. The concentrations of PTX in samples were analyzed under the condition described in Section 2.4. The parameters of blood, heart, liver, spleen, lung, and kidney were evaluated using the statistical moment method.
In Vivo Tumor Efficacy Study
The BALB/c mice model was prepared with inoculation of approximately 1×106 hepatoma solidity (Heps) cell at the right flank of the mice using a 1.0 ml syringe. Animals were kept in an SPF facility and had free access to food and water. When the tumor volume became about 100–200 mm3, pH-sensitive liposomes and Taxol® were intravenously administered (20 mg/kg) to the plain liposomes by tail vein every other day. After 4 times i.v., all the mice were weighed and sacrificed by cervical vertebra dislocation followed by separation and measurement of the tumor. Normal saline was injected for the control group. The tumor volumes of mice were monitored every other day. In order to determine the tumor volume, each individual tumor size was measured with a caliper and the tumor volume was calculated using the following equation: (W2 ×L)/2, where W is the tumor measurement at the widest point and L is the tumor dimension at the longest point.
RESULTS AND DISCUSSION
Stability Studies
An increase in particle size of liposomes generally results in rapid uptake by the reticuloendothelial system (RES) with subsequent rapid clearance and a short half-life. Thus, controlling and maintaining liposomes at small and uniform sizes are critical in developing a viable pharmaceutical product. The particle size distribution of the liposomes prepared in this study showed that there was no significant change in liposome particle size before and after freeze-drying when incubated in PBS or PBS with 50% FBS (), suggesting that the freeze-drying cycle used was optimum and the formulation preserved the integrity of the liposomes. It is interesting to note that the particle sizes of liposomes before and after freeze-drying were almost constant for up to 24 h, which could be used for pharmacokinetics and tissue distribution studies.
Table 1. Stability of plain liposomes and pH-sensitive liposomes with change of particle sizes with different media at 37°C for 1 h, 12 h, 24h
Pharmacokinetic Studies
To assess the pharmacokinetic behavior of PTX loaded in the plain and pH-sensitive modified liposomes, each formulation was at a dose of 7 mg/kg. The pharmacokinetic parameters for PTX in plasma were estimated by the compartmental method. The drug concentrations were detected by HPLC analysis, which has been validated. The plasma concentration profiles of PTX after intravenous injection of Taxol®, the plain liposomes (PL), and the modified liposomes (ML) are shown in . The pharmacokinetic parameters of PTX are shown in . Free PTX in Taxol® was quickly removed from the circulating system after intravenous injection with a rapid distribution phase (t1/2α(h)=0.12h) and a rapid terminal elimination phase (t1/2β(h)=1.93 h), and was under the HPLC detection limit after 6 h. But the plain liposomes and modified liposomes could be detected after 8 h and 12 h. However, liposomal formulations significantly changed the PTX pharmacokinetic parameters in comparison with Taxol®. The values of t1/2β, MRT, and AUC were found to be higher in liposomes than the Taxol®. The total body clearance of PTX incorporated in plain and modified liposomes decreased 1.4- and 2.9-fold, respectively, compared to the Taxol® formulation. The longer t1/2β in the modified liposomes than in the plain liposomes might be about the reduced uptake of liposomal drug by the elements of the mononuclear phagocytic system (MPS) and hence to the reduced clearance.
Figure 1. Plasma concentration profiles of PTX after intravenous administration in rats. Taxol®(PTX-INJ ▀), PTX in plain liposome (PTX-PL •) and modified liposome (PTX-ML ▴). (mean±S.D., n = 5)
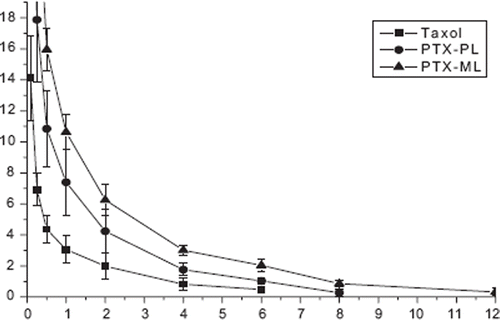
Table 2. The main pharmacokinetics parameters after intravenous administration of injection, PL and ML to rats (mean±S.D. n=5)
Tissue Distribution Studies
The concentration of PTX in each tissue was determined by HPLC analysis. The observed PTX concentrations in blood and tissues were estimated to obtain the pharmacokinetic parameters by statistical moment analysis. The maximum concentration (Cmax) (ug/ml or ug/g) and the time of maximum concentration (Tmax) (h) values were obtained from visual inspection of the data. and summarize the AUC (0-tn) and MRT (0-tn) values of PTX in various tissues and blood. shows the distribution of PTX into various organs after intravenous administration of Taxol® or liposome formulations via tail vein. The plasma concentration of PTX in Taxol® was almost negligible at 8 h, because it may be rapidly uptaken and cleared by the liver and spleen. (). However, when PTX was encapsulated in liposomes, the plasma concentration was maintained for up to 24 h. Moreover, the modified pH-sensitive liposomes showed higher plasma level than that of plain liposomes, which is consistent with the results from the pharmacokinetic study in rats (). As shown in , the PTX concentrations of the PTX-PL group were higher than the Taxol® and PTX-ML group in liver and spleen. and also demonstrate lower AUC (0-tn) values for PTX-ML and Taxol® groups in liver and spleen than the PTX-PL group. Therefore, the liposomes may decrease the elimination by the RES and accumulation in the liver and spleen. The AUC (0-tn) values of PTX-ML in liver and spleen were 1.67- and 2.03-fold lower than that of PTX-PL. This might decrease the accumulation toxic effect in liver and spleen. It is interesting that the pH-sensitive liposomes might have lower accumulation in the liver and spleen than the plain liposomes; thus it is safe and necessary for application of nano-particles in drug delivery system [Citation17,Citation18].
Table 3. AUC (0-tn) ((mg/ml)*h or (mg/g)*h) of PTX in blood and tissues (n=6)
Table 4. MRT (0-tn) (h) of PTX in blood and tissues (n = 6)
Table 5. In vivo antitumor effect of Taxol ®, plain liposomes and pH-sensitive liposomes in tumor bearing mice model
In Vivo Anti-tumor Efficacy
The anti-tumor efficacy of plain liposomes and pH-sensitive liposomes in vivo were evaluated in tumor-bearing BALB/c mice at a dose of 20 mg/kg body weight. As shown in and , First reference to and liposomal formulations suppressed the growth of tumor as compared with the saline control group. The tumor volume of the saline control group was excessively enlarged (about 1000 mm3), while other groups were much smaller. The pH-sensitive liposomes suppressed tumor growth most efficiently, followed by the plain liposomes and Taxol® (p<0.05). As shown in , liposomal formulations also suppressed the growth of tumor as compared with the saline control group (A). The pH-sensitive liposomes (D) also suppressed tumor growth most efficiently, followed by the plain liposomes (C) and Taxol® (B). This enhanced anti-tumor activity of the pH-sensitive liposomes could be explained by the increased local concentration of PTX near the tumor via EPR effect [Citation19,Citation20]. These results indicate that PTX loaded in liposomes could be more slowly released from the pH-sensitive liposome than plain liposomes and then diffused passively into the tumor cells or the PTX-loaded liposomes might be directly internalized by endocytosis after delivery to target cells by EPR effect.
Figure 3. Change of body weight after injection in BALB/c mice inoculated with Heps cancer cells. Taxol® (•), plain liposome (▴) and pH-sensitive liposome (▾) was intravenously injected (20 mg/kg). Normal saline (▀) was injected as a control. The zero point of X-axis indicates the first day of PTX injection. Each data represents the mean±standard deviation (n = 5).

Figure 4. The effect of PTX on the inhibition of tumor growth in BALB/c mice inoculated with Heps cancer cells. Taxol® (•), plain liposome (▴) and pH-sensitive liposome (▾) was intravenously injected (20 mg/kg). Normal saline (▀) was injected as a control. The zero point of X-axis indicates the first day of PTX injection. Each data represents the mean±standard deviation (n = 5).

CONCLUSION
In this research, the pH-sensitive liposomes modified by novel mPEG-Hz-Chol conjugate were evaluated in vivo. The stability result indicated that there was no significant change in liposome particle size before and after freeze-drying when incubated in PBS or PBS with 50% FBS. It is interesting to note that the particle sizes of liposomes before and after freeze-drying were almost constant for up to 24 h, which could be used for the pharmacokinetics and tissue distribution studies. Pharmacokinetics and tissue distribution studies certified that pH-sensitive liposomes might prolong the t1/2β and decrease Cl and the accumulation toxic effect in liver and spleen more than the plain liposomes. The pH-sensitive liposomes suppressed tumor growth most efficiently, followed by the plain liposomes and Taxol® (p<0.05). This enhanced anti-tumor activity of the pH-sensitive liposomes could be explained by the increased local concentration of PTX near the tumor via EPR effect.
These results suggest that it is interesting that mPEG-Hz-Chol conjugates could be used to modified pH-sensitive liposomes as a novel potential and promising nano-carrier in the future.
Declaration of interest: The authors report no conflicts of interest. The authors alone are responsible for the content and writing of the paper.
REFERENCES
- Panchagnula, R. (1998). Pharmaceutical aspects of paclitaxel. International Journal of Pharmaceutics 172: 1–15.
- Sharma, A., Sharma, U.S., Straubinger, R.M. (1996). Paclitaxel-liposomes for intracavity therapy of intraperitoneal P388 leukemia. Cancer Lett 107: 265–272.
- Singla, A.K., Garg, A., Aggarwal, D. (2002). Paclitaxel and its formulations. International Journal of Pharmaceutics 235: 179–192.
- Rowinsky, E.K., Donehower, R.C. (1995). Paclitaxel (taxol). The New England Journal of Medicine 332: 1004–1014.
- Zhang, G.J.A., Ma, L., Ugwu, S., Xuan, T., Sardone, T., Ahmad, I. (2005). Development and characterization of a novel Cremophor® EL free liposome-based paclitaxel (LEP-ETU) formulation. European Journal of Pharmaceutics and Biopharmaceutics 59: 177–187.
- Allen, T.M. (1998). Liposomal drug formulations. Rationale for development and what we can expect for the future. Drugs 56: 747–756.
- Drummond, D.C., O. Meyer, K. Hong, D.B. Kirpotin, D. Papahadjopoulos. (1999). Optimizing liposomes for delivery of chemotherapeutic agents to solid tumors. Pharmacological Reviews 51: 691–743.
- Tilcock, C. (1999). Delivery of contrast agents for magnetic resonance imaging, computed tomography, nuclear medicine and ultrasound. Advanced Drug Delivery Reviews 37: 33–51.
- Webb, M.S., D. Saxon, F.M. Wong, H.J. Lim, Z. Wang, M.B. Bally, L.S. Choi, P.R. Cullis, L.D. Mayer. (1998). Comparison of different hydrophobic anchors conjugated to poly (ethylene glycol): effects on the pharmacokinetics of liposomal vincristine. Biochimica et Biophysica Acta 1372: 272–282.
- Kono, K., K. Zenitani, T. Takagishi. (1994). Novel pH-sensitive liposomes: liposomes bearing a poly(ethylene glycol) derivative with carboxyl groups. Biochimica et Biophysica Acta 1193: 1–9.
- Woodle, M.C. (1998). Controlling liposome blood clearance by surface-grafted polymers. Advanced Drug Delivery Reviews 32: 139–152.
- Moreira, R.G.J.N., T.M. Allen (2001). Targeting stealth liposomes in a mutine model of human small cell lung cancer. Biochim. Biophys. Acta 1515: 167–176.
- Torchilin, A.A.K.A.V.P. (2007). “Smart” drug carriers: PEGylated TATp-modified pH-sensitive liposome. Journal of Liposome Research 17: 197–203.
- Shin, J., P. Shum, D.H. Thompson (2003). Acid-triggered release via dePEGylation of DOPE liposomes containing acid-labile vinyl ether PEG-lipids. J Control Release 91: 187–200.
- Maeda, N., Y. Takeuchi, M. Takada, Y. Sadzuka, Y. Namba, N. Oku (2004). Anti-neovascular therapy by use of tumor neovasculature-targeted long-circulating liposome. J Control Release 100: 41–52.
- Xu, W.W.G.Z.H., J. Huang, H. Sui, Z.H. Zhou, Y.X. Yang, Z. Yan, Y.P. Li (2005). In vitro and in vivo evaluation of actively targetable nanoparticles for paclitaxel delivery. Int. J. Pharm. 288: 361–368.
- Zhang, C., Yu, L.L., Ping, Q. (2007). Polymeric micelle systems of hydroxycamptothecin based on amphiphilic N-alkyl-N-trimethyl chitosan derivatives. Colloids Surf B Biointerfaces 55: 192–199.
- Zhang, C., Sun, Y., Wu, X., Yao, Z., Guo, Q., Ding, Q., Yuan, S., Shen, Z., Ping, Q., Zhou, H. (2008). Pharmacokinetics, biodistribution, efficacy and safety of N-octyl-O-sulfate chitosan micelles loaded with paclitaxel. Biomaterials 29: 1233–1241.
- Jung, S.H., H. Seong, S.H. Cho, K.S. Jeong, B.C. Shin (2009). Polyethylene glycol-complexed cationic liposome for enhanced cellular uptake and anticancer activity. International Journal of Pharmaceutics 382: 254–261.
- Kajiwara, E., K. Kawano, Y. Hattori, M. Fukushima, K. Hayashi, Y. Maitani (2007). Long-circulating liposome-encapsulated ganciclovir enhances the efficacy of HSV-TK suicide gene therapy. J Control Release 120: 104–110.