Abstract
Objective: To investigate the repairing ability of nano-hydroxyapatite/recombinant human bone morphogenetic protein-2 (Nano-HA/rhBMP-2) composite artificial bone for bone defect and to provide evidence for its application in clinical repair of bone defect. Methods: The animal model of bone defect was made on unilateral radius of 90 New Zealand white rabbits, which were randomly divided into experimental group (group A, bone defect was repaired with Nano-HA/rhBMP-2 composite artificial bone), control group (group B, bone defect was repaired with Nano-HA artificial bone), and blank group (group C, bone defect was unrepaired). The repairing ability for bone defect was evaluated by gross observation, X-ray examination, scanning electron microscope, radionuclide bone imaging and biomechanical analysis at 4th, 8th, and 12th week postoperatively. Results: Both Nano-HA/rhBMP-2 composite artificial bone and Nano-HA artificial bone could stimulate new bone formation, but the former could stimulate more new bone formation and had better repairing ability for bone defect than that of the latter, with statistically significant difference (P <0.05). Conclusion: Nano-HA/rhBMP-2 composite artificial bone has good repairing ability for bone defect and it is hopeful to become an ideal repairing material for bone defect.
Bone grafting has been generally acknowledged as an effective repairing method for bone defect [Citation1], but we still haven't developed an ideal artificial material for bone grafting. Nano-hydroxyapatite (HA, [Ca10(PO4)6(OH)2]), with similar components of human bone tissue and good biomechanical properties, is now considered as the most promising bioactive material, but its application is still limited because of its lack of bone induction effect [Citation2]. Therefore, we developed nano-hydroxyapatite/recombinant human bone morphogenetic protein-2(Nano-HA/rhBMP-2) composite artificial bone with bone induction effect, and attempted to evaluate its osteogenic ability and biomechanical properties in animal experiments, so as to provide evidence for its clinical application.
MATERIALS AND METHODS
Materials
Preparation of Nano-HA Artificial Bone. The Nano-HA artificial bone was jointly developed by Shenzhen Second People's Hospital and Powder Metallurgical Engineering Institute of Central South University; the method of Collosol-Flocculation was adopted to make nano-hydroxyapatite powder and the average diameter of pure powder was smaller than 100nm [Citation3]. As seen in , the powder was made into biological polyporous Nano-HA artificial bone with the Wood-Model method. Its good biocompatibility has been confirmed by testing [Citation4] (). The diameter of the pore was 150∼250µm and the gap rate was more than 90 percent ().
Figure 1. Representations of different artificial bones in reality: (a) powder of nano-HA artificial bone; (b) Nano-HA artificial bone; (c) normal HA artificial bone; (d) X-ray diffraction spectrum: a-human bone, b-nano-HA artificial bone.
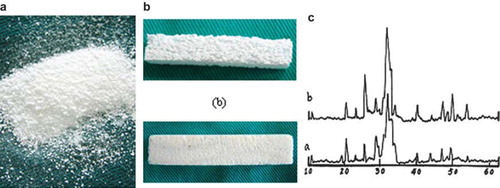
Figure 2. Structure of specimen under invert microscope: (a) Nano-HA artificial bone; (b) Normal HA artificial bone. (×400).
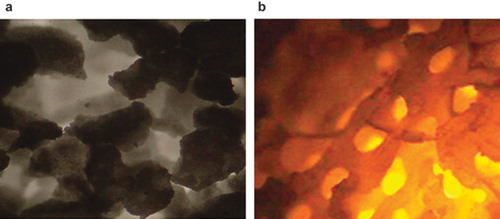
Preparation of Nano-HA/rhBMP-2 Composite Artificial Bone. RhBMP-2 reagent (purchased from Da'an Gene Stock Company of Sun Yat-Sen University, 500ug/ampoule) was dissolved with a proper amount of sodium dihydrogen phosphate solution (1mol/L); the rhBMP-2 solution was then added to the Nano-HA artificial bone in drops to make sure that the binding ratio of rhBMP-2 and Nano-HA was 1:40 (weight); then the artificial bone received aspiration in vacuum negative-pressure aspirator, and after natural drying it was disinfected by epoxyethane for 30 minutes before being placed in an aseptic package [Citation5].
Methods
Grouping. Ninety male New Zealand white rabbits of closed colony and ordinary grade, weighing 1.5–2.0 kg, were randomly divided into groups A, B and C.
Group A: Nano-HA/rhBMP-2 artificial bone group, 30 white rabbits with Nano-HA artificial bone implantation.
Group B: Nano-HA artificial bone group, 30 white rabbits with Nano-HA artificial bone implantation.
Group C: blank group, 30 white rabbits without implantation.
Operative Procedures [Citation6]. The white rabbits were anesthetized by ketamine hydrochloride injection (20 mg/kg) via ear vein. Secondly, unilateral forearm was depilated and disinfected routinely. A 2-cm longitudinal incision was made in the middle segment of the forearm to expose the radial shaft. The radius, together with periosteum, was completely sawed off at 2.5cm distal to radius with a wire saw, and then the radius was sawed off again at 1.5cm proximal to the first osteotomy line with the same method. The radius segment between the bone ends was removed together with periosteum. Before suturing layer by layer, Nano-HA/rhBMP-2 artificial bone and Nano-HA artificial bone were implanted in groups A and B, respectively, and the blank group received no implantation. The local limb was not stabilized by internal or external fixation, and conventional feeding was carried out after operation. The white rabbits received intramuscular injection of 800,000 units of penicillin per day for 3 days.
Observing Parameters and Methods
General Data and Gross Specimen. After operation the diet, activity, and wound response of animals were observed. Specimens were taken to observe surface condition of the implants and inflammatory responses and so on.
X-ray Radiograph. X-ray radiograph was performed on experimental limbs at the 4th, 8th, and 12th week postoperatively to evaluate the condition of bone formation and molding according to the Lane-Sandhu X-ray scoring standard.
Scanning Electron Microscope. Two specimens were randomly selected from each group at the 4th, 8th, and 12th week postoperatively to intercept a 0.5 cm fragment at bone defect area and at both junctures of the bone and the artificial material, respectively. The specimens were then fixed with 3% glutaric dialdehyde and cut open in the middle. After dehydration, drying, block repairing and coating with gold palladium, they were observed under scanning electron microscope (HITACH, S450). Compatibility of the interface between bone and material and the repairing of bone defect were observed and photographed.
Radionuclide Bone Imaging [Citation7]. Five white rabbits were randomly selected from each group at the 4th, 8th, and 12th week postoperatively and fixed on ECT detection device after intravenous anesthesia. Bolus injection of radioisotope 99mTc-MDP(18.5MBq/kg) via ear vein was carried out, and special attention was paid to avoid extravasation. Four hours later, acquisition was performed at 1 frame per minute to get a static whole body imaging. Pegasys software was used to take the corresponding contralateral area of bone defect area as a region of interest (ROI) by image method. By ROI counting, the uptake ratio (average ROI count on the operation side/average ROI count on the contralateral side) was calculated after calculating the average ROI count at different time points.
Biomechanical Test. Two white rabbits were randomly selected from each group at 4th, 8th, and 12th week postoperatively and sacrificed. Bilateral radius was harvested and put on a biomechanical testing machine after removal of periosteum and soft tissue to run a three-point bending test (carried out in Laboratory of Biomechanics, Southern Medical University). The bending strength of each sample was measured and the mean value of each group was used for statistical analysis.
Statistical Analysis
All data of testing parameters were collected and statistically processed with SPSS11.0: t test was used to compare the data of X-ray radiograph, histological score, and three-point bending strength.
RESULTS
General Data
All the animals gained normal diet and activity after surgery. No death or wound infection was observed.
X-ray Radiograph
Group A: the material was partially degraded at the 4th week; further degradation was observed at the 8th week, and the boundary between the bone and the material was fuzzy; the material was fully degraded at the 12th week, with complete recanalization of medullary cavity, complete molding, and bone defect repair ().
Figure 3. X-ray photograph for the bone defect of group A: (a) at 4th week, the material has been degraded partly; (b) at 8th week, the interaction surface between new bone tissues and the material was illegible; (c) at 12th week, the material has been degraded completely, and the bone defect has been repaired.
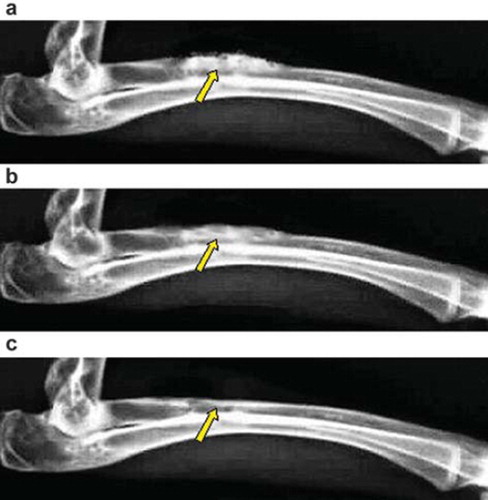
Group B: material degradation began at the 4th week after surgery, and at the 8th week, the material was degraded, and the bone contact at the juncture was getting closer; at the 12th week after surgery, the bone defect area was filled with callus, bone connection formed, and further moulding completed. The medullary cavity was partially recanalized and the bone defect was generally repaired ().
Figure 4. X-ray photograph for the bone defect of group B: (a) at 4th week, the interaction surface between new bone tissues and the material is clear; (b) at 8th week, the interaction surface between new bone tissues and the material is clear; (c) at 12th week, the material has not been degraded completely, and the bone defect has been partly repaired.
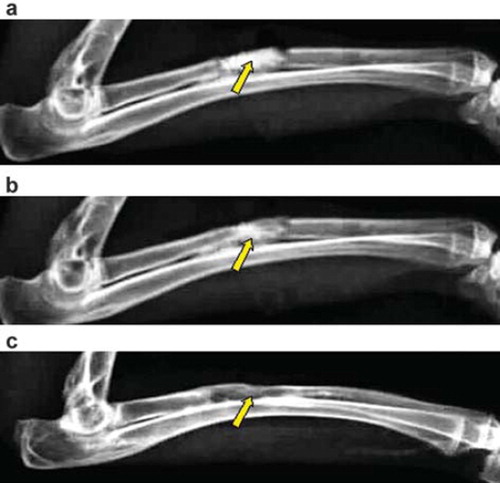
Group C: certain periosteal reaction was observed at the 4th week after surgery, and at the 12th week, when the experiment ended, there was no bone connection in the bone defect area. Capitulum was ossified with medullary cavity blocked, and the bone defect was not repaired.
Statistical analysis showed that at the 4th, 8th and 12th week: group A> group B> group C, with statistically significant difference (P <0.05). Intragroup analysis of group A and B: 4th week< 8th week< 12th week, with statistically significant difference (P <0.05), indicating that Nano-HA/rhBMP-2 artificial bone material had better repairing ability for the bone defect than the Nano-HA artificial bone. Intragroup analysis of the blank group between different periods showed no statistically significant difference (P >0.05), indicating that there was no bone remolding at the bone defect area ().
Table 1. Bone formation and Lane-Sandhu X-ray molding score among different groups (Mean±SD)
SEM Analysis
Group A: material degradation began at the 4th week, and there was a gap between the material and normal bone, inside which was callus; at the 8th week, the material was further degraded, but it also “fused” with normal bone tissue, with a large amount of new osteoid tissue; at the 12th week, the material was fully degraded, and the bone defect area was filled with new mellous bone tissue. The bone defect was completely repaired.
Group B: at the 4th week after surgery, the material was partially degraded, and a tiny gap formed between the material and the bone tissue without abnormal tissue inside. There was contact between the material and the bone tissue; at the 8th week after surgery, the bone and the material were partially combined, with the degradation and absorption of the material. The gap between the bone and the material was less obvious; at the 12th week, the material was degraded, with the medullary cavity partially recanalized and bone defect partially repaired.
Radionuclide Bone Imaging
Most radioactive bone imaging agents deposited in bone, thus clearly displaying joints, kidneys, and bladder with obvious increase of radioactivity. This suggested that bone imaging agents were excreted mostly by the urinary system.
Group A: radioactivity concentration on the operation side at the 8th week after surgery was obviously higher than that of the 4th week, but the radioactivity concentration at the 12th week was lower than that of the 8th week, while the contralateral area showed no obvious change over time ().
Figure 5. Radionuclide bone imaging photo of group A: (a) at 4th week; (b) at 8th week; (c) at 12th week, respectively.
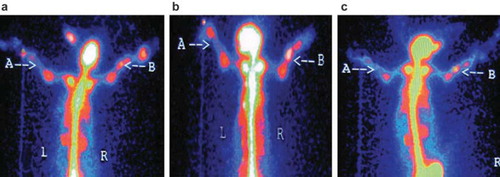
Group B: radioactivity concentration at the 8th week after surgery was higher than that of the 4th week; radioactivity concentration peaked at the 8th week, and radioactivity concentration on the operation side was higher than that of the contralateral side ().
Figure 6. Radionuclide bone imaging photo of group B: (a) at 4th week; (b) at 8th week; (c) at 12th week, respectively.
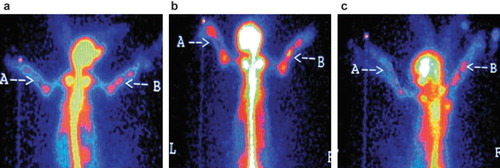
Group C: radioactivity concentration on both the operation and contralateral side showed no obvious change over time.
Statistical analysis indicated that the uptake ratio of groups A, B and C, 8th week> 4th week> 12th week; with statistically significant difference (P <0.05). During the 4th and the 12th week after surgery, the intragroup comparison of the uptake ratio in blank group showed no statistically significant difference (P <0.05), indicating that there was no significant change among the 4th, 8th, and 12th week. At the 4th, 8th, and 12th week postoperatively: group A> group B> group C, with statistically significant difference (P <0.05).
Biomechanical Test
In three-point bending tests, the three-point bending strength of the specimens from the experimental group at different time points was statistically analyzed by non-paired t test (), showing that: at the 4th, 8th and 12th week, group A> group B, with statistically significant difference (P <0.05); intragroup analysis of group A and group B: 12th week> 8th week> 4th week, with statistically significant difference (P <0.05), indicating that Nano-HA/rhBMP-2 artificial bone has better osteogenic ability than Nano-HA artificial bone. But after implanting the material into the bone defect area, the local mechanical strength could be enhanced over time.
Table 2. Intape ratio of operative site in static phase (S, n=5)
Table 3. Biomechanical test results of three different groups (Mean±SD, n = 4)
DISCUSSIONS
Functions of Bone Repair Material and Outcomes
The functions of bone repair material implanted in bone defect area are as follows [Citation8]:
Bone conduction effect: the main function of the bone graft is to help the bone obtain a support, which means the bone graft is a support for the blood vessels and cells for vasiformation in bone graft and new bone formation, then the bone graft necrosis and get absorbed, thus gradually getting replaced by new bone.
Bone induction effect: when implanted into non-bone tissue, some bone graft can induce ectopic osteogenesis, which means the bone graft has the ability to stimulate mesenchymal cells of the host to transform into osteoblasts in order to produce new bone.
During the repairing of bone defect by Nano-HA/rhBMP-2 composite artificial bone, the bone replacement function of its inorganic material Nano-HA is mainly based on its bone conduction effect [Citation9]. At first, the callus around the artificial graft converges by crawling and encapsulates the graft, thus filling and connecting the bone defect; on the other hand, the microspores in the artificial bone are helpful for surrounding blood vessels and cells (osteoblasts) to enter and transform into osteoblasts gradually, then partially or fully fill the pores with new bone. Bone growth factor rhBMP-2 stimulates the formation of new bone mainly by its bone induction effect; that is, by stimulating the formation of new bone or impelling the surrounding mesenchymal cells differentiating into chondroblasts and osteoblasts, which may further produce cartilage and bone tissue [Citation10].
Nano-HA Material is an Ideal Vector for rhBMP-2
This experiment produced new Nano-HA as a vector to combine rhBMP-2, and the result showed that Nano-HA/rhBMP-2 composite artificial bone had better osteogenic ability than Nano-HA artificial bone, proving that Nano-HA is an ideal vector for rhBMP-2, and the main reasons are as follows:
Nano-HA has good biocompatibility, which is easy for cell adsorption and has good affinity for biomacromolecules.
The aporous structure of Nano-HA is suitable for fibrous connective tissue and blood vessels to enter, providing mesenchymal cells for exerting bone induction effect of rhBMP-2 in the material [Citation11].
Nano-HA provides a large surface area for rhBMP-2 adsorption. The delayed release effect of the material can stop rhBMP-2 from rapid diffusion and decomposition after entering the host [Citation12]. When fibrous connective tissue enters the pores of the material, a certain concentration of rhBMP-2 can induce the differentiation of mesenchymal cells to promote new bone formation.
The porous structure of Nano-HA and specific “nanometer effect” of the nanometer material greatly increase its surface area, providing good support for revascularization of the material, formation of bone matrix, and deposit of calcium salt [Citation9]. Moreover, the bracket structure is very necessary [Citation13].
Advantages of Nano-HA/rhBMP-2 Composite Artificial Bone
In this experiment, the bone defect of the Nano-HA/rhBMP-2 composite artificial bone group was well repaired, indicating that Nano-HA/rhBMP-2 composite artificial bone has some advantages [Citation14]: (1) it can be put into mass production, so the resource is not limited; (2) it has good biocompatibility, without any toxic and side-effects to the host; (3) it has excellent repairing biological properties for bone defect; (4) it has excellent biomechanical properties.
In this experiment, the results of X-ray radiograph, SEM, and ECT showed that Nano-HA/rhBMP-2 composite artificial bone had better osteogenic ability than Nano-HA artificial bone, and the osteogenic effect for repairing of bone defect began at the 4th week after implantation, and peaked at the 8th week. At the 12th week after surgery, the bone defect was repaired. The mechanisms are as follows: (1) “Nanometer small dimension effect” of the material [Citation15,Citation16]. This effect brings the material a larger surface area than HA artificial bone, which is helpful for degradation and absorption, while proper degradation rate makes it match the rate of new bone formation. Meanwhile, absorption of the material makes the aperture larger, which is helpful for the revascularization of local material, the entry of microvessels and fibrous connective tissue, and the crawling of osteoblasts. Furthermore, the “small dimension effect” obviously increases the surface area of unit mass molecules, thus obviously increasing the number of atoms on the surface, then increasing the biological activity of nano-particles and the osteogenic ability of osteoblasts. (2) Bone induction effect of rhBMP-2 in composite materials [Citation17,Citation18,Citation19]. The bone induction effect of composite artificial bone is from rhBMP-2, which acts on two kinds of cells in the bone defect area, including undifferentiated mesenchymal cells in periosteum and other soft tissue, and stromal cells in bone marrow, thereby impelling them to differentiate into cartilage and bone tissue irreversibly, while the Nano-HA in composite artificial bone acts as a sustained release carrier of rhBMP-2, enhancing its bone induction effect. After implantation, it not only helps to conduct the bone tissue of the bone bed to grow inside but also to produce new bone by bone induction, that is, bone defect repairing is achieved by bone conduction and bone induction.
Though rhBMP-2 plays a crucial role in bone induction, it is undeniable that Nano-HA artificial bone provides support in the bone defect area and conducts the bone tissue in the bone bed to grow inside. Its porous structure and its gradual degradation provide space for new bone, which can be seen from the better result of Nano-HA artificial bone than HA artificial bone in bone defect repairing [Citation20]. So, rhBMP-2 and Nano-HA in composite artificial bone can mutually promote and supplement, repairing the bone defect together. Therefore, in our opinion, Nano-HA/rhBMP-2 composite artificial bone has many advantages that HA artificial bone doesn't have, and is a promising substitute material for bone graft.
Declaration of interest: The authors report no conflicts of interest. The authors alone are responsible for the content and writing of the paper.
REFERENCES
- Iwakami, T., Imai, T. (2002). Effect of hydroxyapatite sol on cell proliferation and alkaline phosphatase activity of osteoblastic MC3T3-E1 cells. Biomed. Mater. Eng. 12(3): 249–257.
- Seeherman, H., Li, R., Bouxsein, M., . (2006). rhBMP-2/calcium phosphate matrix accelerates osteotomy-site healing in a nonhuman primate model at multiple treatment times and concentrations. J Bone Joint Surg Am. 88(1): 144–160.
- Wan, J.M. (2000). China Patent: Produce Method of Polyporous Biomaterial. ZL 91106753.1 (2000. 1. 22).
- Jiang, H.P., Wang, D.P. Ruan, J.M., . (2005). Study on toxicity and cytocompatibility of nano-hydroxyapatite artificial bone. China Medical Engineering. 10(5): 458–461.
- Nemoto, K., Takita, H., Yoshimoto, R., George, J., Miyata, T., Kuboki, Y. (2002). Geometry of honeycomb collagen scaffold gives chamber-type micro-environmental units for ectopic osteogenesis when implanted with purified BMP cocktail into rat skin. Tissue Eng. 8: 1148–1156.
- Nakano, M., Hirano, N., Ishihara, H., . (2006). Calcium phosphate cement-based vertebroplasty compared with conservative treatment for osteoporotic compression fractures: a matched case-control study. J Neurosurg Spine 4(2): 110–117.
- Jiang, H.P., Wang, D.P. Zhu, W.M., . (2006). The experimental study on radionuclide bone imaging in repairing bone defect. Chinese Journal of Clinical Anatomy 12(4): 34–36.
- Goldberg, V.M., Stevenson, S., Shaffer, J.W. (1991). Biology of autografts and allografts. Friedlander G. Bone and Cartilage Allografts, American Academy of Orthopaedic Surgeons, Park Ridge, IL, 1: 3–13.
- Jouve, J.L., Mottet, V., Cottalorda, J., Frayssinet, P., Bollini, G. (1998). Reimplantation of growth plate chondrocyte cultures in central growth plate defects: Part 1. Characterization of cultures. J Pediatr Orthop B 7: 167–173.
- Choi, S., Kim, C., Cho, K., . (2002). Effect of recombinant human bone morphogenetic protein22/absorbable collagen sponge (rhBMP22/ACS) on healing in 32 wall intrabony defects in dogs. Periodont 73(1): 63–72.
- Wu, F., Wei, J., Guo, H., . (2008). Self-setting bioactive calcium-magnesium phosphate cement with high strength and degradability for bone regeneration. Acta Biomater. 4(6): 1873–1884.
- Itoh, H., Aso, Y., Furuse, M., Noishiki, Y., Miyata, T. (2001). A honeycomb collagen carrier for cell culture as a tissue engineering scaffold. Artif. Organs 25(3): 213–217.
- Tsai, C.H., Lin, R.M., Ju, C.P., . (2008). Bioresorption behavior of tetracalcium phosphate-derived calcium phosphate cement implanted in femur of rabbits. Biomaterials 29(8): 984–993.
- Zhu, W.M., Wang, D.P., Xiong, J.Y., . (2006). Revascularization study of the Nano-hydroxyapatite artificial bone in repairing the bone defect. Journal of Chinese Microcirculation 10(5): 344–348.
- Zhu, W., Zhang, X., Wang, D. (2010). Experimental study on the conduction function of nano-hydroxyapatite artificial bone. Micro & Nano Letters 1(5): 19–27.
- Zhu, W.M., Xiao, J.D., Wang, D.P., . (2009). Experimental study of nano-HA artificial bone with different pore sizes for repairing the radial defect. International Orthopaedics 2(33): 1345–1350.
- Guo, D., Xu, K., Han, Y. (2009). The in situ synthesis of biphasic calcium phosphate scaffolds with controllable compositions, structures, and adjustable properties. J Biomed Mater Res A. 88(1): 43–52.
- Tatakis, D.N., Koh, A., Jin, L., . (2002). Peri2implant bone regeneration using recombinant human bone morphogenetic protein22 in a canine model: a dose response study. Periodont Res. 37(2): 93–100.
- Marukawa, E., Asahina, I., Oda, M., . (2001). Bone regeneration using recombinant human bone morphogenetic protein22 (rhBMP22) in alveolar defects of primate mandibles. Br J Oral Maxillofac Surg. 39(6): 452–459.
- Wang, X., Ye, J. (2008). Variation of crystal structure of hydroxyapatite in calcium phosphate cement by the substitution of strontium ions. J Mater Sci Mater Med. 19(3): 1183–1186.