Abstract
A highly sensitive, amperometric uric acid biosensor possessing unique physical, electro-conductive properties of carbon nanotubes is described for determination of uric acid level in serum. A uric acid biosensor was constructed after immobilization of uricase onto a polyaniline/CNT matrix by carbonamide linkage. The scanning electron micrographs confirmed the immobilization of globular enzyme onto the electroploymerized polyaniline/CNT composite. Employment of carbon nanotubes composite in the present electrode leads to a very quick response time of 8 sec with the minimum detection limit of 5 μM along with an increase in shelf-life of electrode system to >180 days with slight loss of enzyme activity. Efficiency of the present amperometric uric acid biosensor was validated by quantitative estimation of uric acid level in biological serum of healthy individuals and the persons suffering from hyperuricemia and gout.
INTRODUCTION
Uric acid (2,6,8-trihydroxypurine), the end product of purine metabolism is present in blood and urine [Citation1,Citation2]. Monitoring uric acid in the blood or urine is important as it is a powerful indicator of early signs of kidney and metabolic disorders. The normal level of uric acid in serum is between 0.13 and 0.46mM (2.18–7.7 mg dl−1) [Citation2,Citation3]. However, abnormal uric acid level in a human body could be caused by several diseases, such as gout, hyperuricemia, Lesch-Nyan syndrome, cardiovascular and chronic renal disease [Citation1–4]. Furthermore, a few other diseases, such as leukemia and pneumonia, are also related with the urate levels. Various techniques, such as spectrophotometric [Citation2], thermometric [Citation5], HPLC [Citation6], etc., have been employed for estimation of uric acid in different biological fluids. Besides being highly sensitive, these methods have some limitations, including being labor-intensive, requiring expert handling, involving pretreatment of the sample, and being time-consuming [Citation7]. With advances in biosensor technology, it has become a popular and reliable technique for the estimation of different metabolites [Citation8,Citation9]. With numerous advances reported in the field of biosensors, the immobilization of enzymes on electrodes in the designing and optimization of biosensors has attracted many researchers. Various electrochemical biosensors have been reported so far for uric acid determination in different biological samples. A large number of studies have been reported where uricases have been immobilized on different transducers by employing various methods of immobilization, such as crosslinking [Citation10–14], entrapment [Citation15,Citation16], adsorption [Citation1], and covalent coupling [Citation17]. They have the advantages of determining uric acid with high sensitivity in less time [Citation3]. But still there are a few issues in which improvement is needed, such as lack of stability, high response time, low reproducibility, and the requirement of high working electrode potential.
Recently, the biocompatible nanomaterials have opened a bright field towards the development of third generation biosensors based on the direct electron transfer between the enzyme and the electrode [Citation18–20]. Among biocompatible nanomaterials, carbon nanotubes (CNT) are one of the popular materials for designing conducting thin films, including those for catalytic membranes actuation and mechanical thin film applications. Currently they are being widely used for electrochemical energy conversion and storage devices due to their unique physical properties, including high electrical conductivity, superior chemical and mechanical stability, and large surface area. Carbon nanotubes have established themselves as a good material for immobilization of enzymes as they could keep enzyme activity due to the desirable microenvironment and enhance the direct electron transfer between the enzyme's active sites and the electrode [Citation21–23].
In the present study, we describe a uric acid biosensor employing covalently immobilized uricase onto MWCNT (Multi Walled Carbon Nanotubes)/PANI (Polyaniline) composites following its application in serum uric acid determination. The performance of this proposed uric acid biosensor showed excellent analytical characteristics along with short response time, good shelf life, and negligible interference of serum metabolites.
MATERIALS AND METHODS
Chemicals and Reagents
Bacillus fastidious uricase (E.C.1.7.3.3) with a specific activity of 16 Umg−1 (Fluka), uric acid (Sigma), distilled aniline (ANI) (Aldrich), indium tin oxide (ITO) coated glass plates (from Biomolecular Electronics and Conducting Polymer Research Group (BECPRG), National Physical Laboratory (NPL), New Delhi) were used in this study. The multi walled carbon nanotubes (MWCNT) were prepared via the catalytic chemical vapor deposition (CCVD) technique [Citation24]. All other chemicals used in the study were of analytical reagent (AR) grade.
Assay of Free Enzyme and Immobilized Enzyme
The uricase assay was performed with slight modifications [Citation25]. The decrease in A293 was read in a UV spectrophotometer for 4 min at an interval of 1 min. The assay of immobilized enzyme was performed similarly, except the free enzyme was replaced by immobilized enzyme electrode. The activity of enzyme was calculated as follows: (λmax = 293)
Here one unit of enzyme is defined as amount of uricase required to convert 1.0 μmol of uric acid to allantoin per minute per ml at pH 8.5 and 25°C.
Construction of Working Uricase/MWCNT/PANI/ITO Electrode
Deposition of cMWCNT/PANI on ITO Coated Glass Plate. Carbon nanotubes were dispersed in the presence of aggressive acids with some modifications [Citation26]. 4mg of MWCNTs were added into 4ml of solution A (mixture of conc. H2SO4 and HNO3 in the ration of 3:1), followed by ultrasonication in a water bath at 35°C for 48 h. The resulting suspension was diluted with 4ml of distilled water and again ultrasonicated for 24hr. One ml of dispersed MWCNT solution was again dissolved in 10ml of solution A to obtain the black-colored, uniformly dispersed, carboxylated MWNTs (cMWNTs) solution. Later on, 1.0 ml of finely dispersed cMWCNT and 50 μl of ten-fold diluted (with I N HCl) aniline solution were added into the glass cell.
Final deposition of aniline and MWCNT onto the ITO coated glass plate (2×1cm2) was done through electroploymerization in an electrochemical cell system using Potentiosatat-Galvanostat, (Prinston Applied Research, U.S.A, Model 273A). The electrochemical polymerization of aniline along with cMWCNT onto the ITO-coated glass plate (working electrode) was achieved by chronoamperometry technique by applying eight polymerization cycles at 0.6-0.8 V with a constant current of 150μA for 960s with intermittent shaking after every 120s [Citation15]. The resulting PANI/MWCNT/ITO electrodes were washed thoroughly with distilled water to remove unbound matter and kept in a dry Petri-plate at 4°C.
Immobilization of Uricase onto cMWCNT/PANI. 5μl (16U) of Bacillus uricase solution (1mg/ml in 50 mM Tris-HCl buffer, pH 8.5) was placed onto surfaces of cMWCNT/PANI/ITO glass plates and kept overnight at 4°C for the immobilization. The resulting electrode with immobilized uricase was washed 3-4 times with a buffer solution (50 mM Tris–Cl buffer pH 8.5) to remove residual unbound enzyme. Protein concentration was calculated in wash-out solution to calculate conjugation yield. The resulting Uricase/MWCNT/PANI/ITO coated glass plates were used as working electrodes and stored at 4°C when not in use.
Scanning Electron Microscopy
The scanning electron microscopy (SEM) was performed to get the microstructure of the surface of PANI electrode, MWCNT/PANI and uricase immobilized MWCNT/PANI electrode.
Cyclic Voltametric Response
Cyclic voltammetry responses were studied using a three-electrode cell comprising of Ag/AgCl as a reference electrode, platinum wire as a counter electrode, and Uricase/MWCNT/PANI electrodes as a working electrode in 50 mM Tris–Cl buffer of pH 7.0. Cyclic voltammograms of Uricase/MWCNT/PANI/ITO electrode were recorded in 50 mM Tris–Cl buffer containing uric acid concentration varying 0.005-0.6 mM at a scan rate of −1V to +1V/s with 30 mVs−1 in Potentiosatat-Galvanostat.
Response Measurement of Uricase/MWCNT/PANI/ITO Electrode and its Optimization
The amperometric response of Uricase/MWCNT/PANI/ITO electrodes were measured and optimized with an electrometer (6517 A/E (Keithley Instruments, Germany) by applying a potential of 0.4V (as determined by cyclic voltametric scan from +1V to −1V).
Amperometric response of the working electrode was optimized after optimization of assay conditions like calculation of optimum pH, optimum temperature, response time, etc. To calculate optimum pH, pH of reaction buffer was varied from pH 5.0 to 10.0 using different buffer systems within their effective pH ranges; for example, 0.05 M sodium succinate for pH 4.0 to 5.5, 0.05 M sodium phosphate for pH 5.5 to 6.5, and 0.05 M Tris–Cl for pH 7.0 to 9.0.
Similarly, the optimum temperature was studied by incubating the reaction mixture from 20°C to 50 °C at the interval of 5°C. Optimum response time was checked after taking the readings at different time scales ranging from 2 sec to 12 sec. The effect of substrate concentration was checked at different uric acid concentration ranging from 0.005 mM to 0.7 mM.
The amperometric response was also measured in the presence of potential interfering compounds and metabolites present in biological fluids, such as glucose, urea, ascorbic acid, pyruvate, bilirubin, cholerstrol, KCl, NaCl, CaCl2, MgCl2, CaSO4, ZnSO4 .
Serum Uric Acid Determination by Uricase/MWCNT/PANI/ITO Electrode
Fresh serum samples from apparently healthy individuals, persons suffering from hyperureicemia, gout, and other nephropathies corresponding to different sex/age groups, were collected from the hospital of the local Pt BDS P.G. Institute of Medical Science, Rohtak (India). Later on, these samples were analyzed for uric acid using the present electrode system. The assay procedure was the same as that described for testing of the electrode, except that uric acid was replaced by serum. The uric acid concentration was determined from the standard plot, extrapolated between different uric acid concentration vs. respective current (mA). Results were evaluated using different statistical parameters and correlation was studied using standard enzymic colorimetric kit.
Reusability and Storage Stability of Uricase/MWCNT/PANI Electrodes
The reusability and stability of the working electrode was checked for more than six months by performing the uricase assay on a daily basis. The present electrode system was stored in dried condition at 4°C when not in use.
RESULTS AND DISCUSSION
Electropolymerization of PANI–MWCNT Composite Film
In the formation of PANI–MWCNT composite film using electroploymerization technique, good quality films are obtained on an ITO-coated glass electrode at an applied voltage of 600–800 mV for 960s. During the composite film formation, gentle shaking of the colloidal solution at an interval of 120s was necessary to achieve desired dispersion, leading to the deposition of homogeneous film.
Immobilization of Uricase onto MWCNT/PANI/ITO Electrode
To construct the present electrode, uricase was immobilized on to MWCNT/PANI/ITO electrode using covalent coupling process. Incubation of free enzyme solution with activated MWCNT/PANI/ITO electrode system leads to effective collision between −COOH group of MWCNT and −NH2 groups on the surface of the enzyme (uricase) to form an amide bond (−CONH) in the presence of tin oxide (SnO2). A schematic diagram given below depicts the different reactions involved in the present immobilization (). The immobilized uricase onto a MWCNT/PANI/ITO glass plate retained 69% of the initial activity of free enzymes with a conjugation yield of 1.1mg/cm2..
SEM of Uricase/MWCNT/PANI/ITO Electrode
The surface of the PANI/ITO, MWCNT/PANI/ITO electrode without and with the immobilized enzyme (, , and , respectively) was observed under a scanning electron microscope. High-resolution scanning electron micrographs of MWCNT/PANI/ITO electrode surface in comparison of PANI/ITO describes even ramification of MWCNT onto the PANI/ITO surface. Along with this, micrographs of the MWCNT/PANI/ITO electrode with the immobilized enzyme had some beaded/globular structures, which were not observed in the MWCNT/PANI/ITO electrode without the immobilized enzyme. A change in surface morphology of the support after the immobilization process is evidence of enzyme immobilization [Citation21]. Similarly, in the current study the presence of these structures indicated immobilization of uricase on MWCNT/PANI/ITO electrode surface.
Cyclic Voltametery Response
Cyclic voltammograms of the Uricase/MWCNT/PANI/ITO electrode in 50 mM Tris–Cl buffer containing uric acid concentration varying 0.05-0.6 mM at a scan rate of −1V to +1V/s with 30 mVs−1 indicates the peak potential of this present electrode system is at 0.4 volts. This peak potential at 0.4V indicates that in the present electrode system at this electrode potential there will be optimal transfer electrons between the enzyme and electrode system. Therefore this electrode potential will be further used for the amperometric study to get the optimal response of the present electrode system.
Response Measurement with Uricase/MWCNT/PANI/ITO Electrode (Working Electrode)
The amprometric response of the Uricase/MWCNT/PANI/ITO electrode was measured in terms of mA and carried out by applying a potential of 0.4V (as determined by cyclic voltametric scan from +1V to −1V) using an electrometer (6517 A/E (Keithley Instruments Germany). The reaction mixture consisting of 3.0 ml of 50 mM Tris-HCl buffer pH 7.0 was taken in a plastic cell. All three electrodes (working electrode, Ag/AgCl reference electrode, and Pt wire as counter electrode) were immersed into the buffer. Prior to response measurements, the steady state current was achieved by polarizing the working electrode at 0.4V in the reaction buffer. On the addition of 0.05 ml 10 mM uric acid, it was oxidized to allantoin, producing an electroactive H2O2, which was split into 2H+ + O2 +2e− under a potential of 0.4 V. The flow of e−, i.e. current, was measured in mA ().
Optimization of Uricase/MWCNT/PANI/ITO Electrode
As there is change in conformation of the enzyme after immobilization, so various kinetic properties need to be optimized for optimal response of the uricase/MWCNT/PANI/ITO electrode. The optimum pH response was at pH 7.0. which is lower than that of the free enzyme (pH 8.0). The pH activity relationship of any given enzyme depends on the acid base behavior of the enzyme and substrate as well as many other factors that usually are difficult to analyze quantitatively. The H+ concentration present in the microenvironment of immobilized enzyme controls enzyme activity. It is related mainly to the degree of dissociation and electric charge, through which it affects the formation of the enzyme substrate complex. The decrease in optimum pH after immobilization might be either due to the presence of negatively charged matrix in surroundings (carboxylated CNT) or due to alerted conformation of enzyme after immobilization. Along with this change in optimum pH of the immobilized uricase towards the neutral side makes it more efficient in quantitative determination of uric acid in biological fluids like serum (pH 7.4) and urine (pH = 6.5), as there will be no need to pre-treat the samples to adjust their pH to electrode optimal range.
The optimum response of the present electrode was observed within 8s. A very low response time of the present biosensor might be due to the rapid rate of electron transfer from the enzyme to the polyaniline through MWCNT ().
Figure 3. Scheme of electron flow and current generation in uric acid biosensor employing Uricase/MWCNT/PANI/ITO electrode.
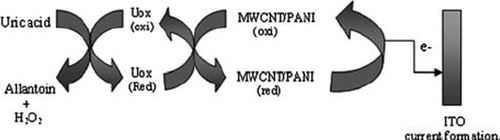
The response of the present electrode system in reference to the change in uric acid concentration was found linear in the range of 0.005 to 0.6 mM () with a Km value of 0.4 mM. The decrease in Km value for uric acid after immobilization indicates increased affinity of immobilized enzyme towards its substrate. This Km value was comparable with those employing pyrrole on Pt electrode (0.44mM) [Citation27], but lower than those of the self-assembled monolayer on Au electrode (0.90 mM) [Citation28], polyaniline uricase with template process (2.31 mol/dm3) [Citation31], Poly-o-aminophenol-aniline copolymer (Km 10.08mM) [Citation29], and polyaniline (7.83 mM) [Citation30]. The effect of biological components on the response of the biosensor was evaluated by exposing the biosensor to some potential interferents in the reaction buffer at pH 7.0. The decrease in the current was calculated as uric acid concentration equivalence (i.e. the concentration of uric acid that can produce the same decrease as the interferents). Negligible interference was observed with glucose, urea, ascorbic acid, pyruvate, bilirubin, cholesterol, KCl, NaCl, CaCl2, MgCl2, CaSO4, ZnSO4 ().
Table 1. Effect of various serum substances on response of Uricase biosensor employing Bacillus and Vigna uricase/MWCNT/PANI/ITO electrode
Serum Urate Determination
Serum samples were tested for the presence of uric acid by replacing the substrate with 0.5 ml of serum sample. The uric acid in the serum of apparently 20 healthy persons and in 20 gout patients was found to be 3.2 to 7.7 mg/dl with a mean of 5.3mg/dl and 9.6 mg/dl to 16.1mg/dl with a mean of 14.2 mg/dl, respectively.
Evaluation of the Biosensor
The minimum detection limit of the present electrode system was 5μM. The percentage recovery of added uric acid in serum (10 mg/L and 20 mg/L) was 86%±0.4 and 81.2% ± 0.4 (mean ± S.D; n=6), respectively, in the reaction mixture. The within and between batch coefficient of variation (CV) for uric acid determination in serum by the present biosensor were <2.7% and <2.3%, respectively. The accuracy of the present method was estimated by comparing uric acid values in 60 serum samples (30 healthy and 30 diseased) by the present method (y) with those obtained by kit for enzymic colorimetric method (x). The values obtained by both methods showed good correlation with r = 0.98 with regression equation, y = 0.969x − 0.046 (). These results indicate the better analytical performance of the present biosensor in biological samples as compared to earlier biosensors [Citation32].
Figure 5. Correlation between serum uric acid values of diseased individuals determined by standard colorimetric method employing free enzyme (x-axis) and the present by biosensor employing Bacillus uricase/MWCNT/PANI/ITO electrode method (y-axis) (n=60), regression equation being y = 0.969x − 0.046.
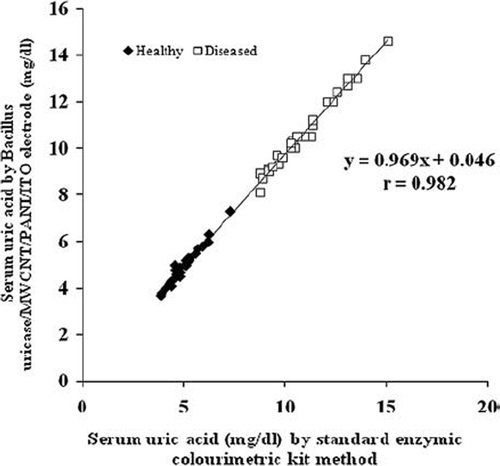
Reusability and Storage Stability of Uricase/MWCNT/PANI/ITO Electrode
Uricase/MWCNT/PANI/ITO electrode stability was measured intermittently every day for a time duration of over 6 months. The uricase/MWCNT/PANI/ITO electrodes showed practically no loss of their initial activity over a period of 3 months after their regular use for 200 times when stored dried at 4°C. Afterwards there was a gradual but low decrease in their response, which amounted to 40% after 6 months () which is found higher than other published studies (). The good shelf-life of Uricase/MWCNT/PANI electrode is attributed to the presence of carbon nanotubes having a large surface area and strong affinity to bind with the enzyme. Comparison of the stability of various uric acid biosensors is made in .
Table 2. A comparison of present uric acid biosensor with earlier reported amperometric biosensors
CONCLUSIONS
The uricase/MWCNT/PANI electrode revealed improved analytical performance in terms of sensitivity, i.e. minimum detection limit (5μM), high recovery (91.0%), precision (<2.7% & <2.3%) within and between batches, coefficient of variation along with excellent reproducibility, stability up to 6 months, and no interference of other serum components. The present electrode system describes the enhanced performance of the electrode system with the application of carbon nanotubes, which indeed describes the application of nano particles in development of highly sensitive, accurate, and stable biosensors.
Declaration of interest: The authors report no conflicts of interest. The authors alone are responsible for the content and writing of the paper.
REFERENCES
- Zhang Y.Q., Shen W.D., Gu R.A., Zhu J., Xue R.Y. (1998). Anal. Chim. Acta. 369(1): 123.
- Huang S.H., Shih Y.C., Wu C.Y., Yuan C.J., Yang Y.S., Li Y.K., Wu T.K. (2004). Biosens. Bioelectron 19(12): 1627.
- Raj C.R., Ohsaka T. (2003). J. Electroanal. Chem. 540(1):69.
- Ren W., Luo H.Q., Li N.B. (2006). Biosens. Bioelectron. 21(7): 1086.
- Shimohigoshi M., Karube I. (1996). Sens. Actuators B 30(1): 17.
- Dai. X., Fang X., Zhang C., Xu R., Xu B. (2007). J. Chromatogr B 857(2): 287.
- Arora J., Nandwani S., Bhambi M., Pundir C.S. (2009). Anal Chim Acta 647(2): 195.
- Hasebe Y., Nawa K., Ujita S., Uchiyama S. (1998). Analyst 123(8): 1775.
- Hoshi T., Saiki H., Anzai J.I. (2003). Talanta 61(3): 363.
- Arslan F. (2008). Sensors, 8: 5492.
- Nanjo M., Guilbault G.G. (1974). Anal. Chem. 46(12): 1769.
- Zhang Y., Wen G., Zhou Y., Shuang S., Dong C., Choi M.M. (2007). Biosens Bioelectron 2(8): 1791.
- Zen J.M., Lai Y.Y., Yang H.H., Senthil K.A. (2002). Sens Actuator B 84(2–3): 237.
- Zhang F., Wang X., Shiyun A.I., Sun Z., Wan Q., Zlu Z., Xian Y., Jin L. (2004). Anal Chim Acta 519(2): 155.
- Arora K., Sumana G., Saxena V., Gupta R.K., Gupta S.K., Yakhmi J.V., Pandey M.K., Chand S., Malhotra B.D. (2007). Anal Chim Acta 594(1): 17–23.
- Kan J., Pan X., Chen C. (2004). Biosens Bioelectron 19(12): 1635.
- Castillo M.M., Rodriguez D.E., Encinas J.C., Plascencia M., Mendz F.A., Olayo R. (2002). Sens. Actuators B 85(1–2): 19.
- Jia J.B., Wang B.Q., Wu A.G., Cheng G.G., Li Z., Dong S.J. (2002). Anal. Chem. 74(9): 2217.
- Ghindilis A.L., Atanasov P., Wilkins E. (1997). Electroanalysis 9(9): 661.
- Gorton L., Lindgren A., Larsson T., Munteanu F.D., Ruzgas T., Gazaryan I. (1999). Anal. Chim. Acta 400(1): 91.
- Liu S.Q., Yu J.H., Ju H.X. (2003). Electroanal. Chem. 540(1): 61.
- Gu H.Y., Yu A.M., Chen H.Y. (2001). J. Electroanal. Chem. 516(1): 119.
- Xiao Y., Ju H.X., Chen H.Y. (2000). Anal. Biochem. 278(1): 22.
- Mathur R.B., Pande S., Singh B.P., Dhami T.L. (2008). Polym. Compos. 29(7): 717.
- Yasuji K., Toshio I., Eiichi N. (1996). J. Biochem 120(5): 969.
- Tsai Y.C., Li S.C., Liao S.W. (2006). Biosens Bioelectron 22(4): 495.
- Cete S., Yasar A., Arslan F. (2006). Artif Cells Blood Substit Immobil Biotechnol 34(3): 367.
- Kuwabata I.S., Nakminami T.S., Yoneyama H. (1998). Sens Actuator B. 52(1–2): 77.
- Pan X., Zhou S., Chen C., Kan A. (2006). Sens Actuator B 113(1): 334.
- Jiang Y., Wang A., Kan J. (2007). Sens. Actuator B 124(2): 529–534.
- Kan J., Pan X., Chen C. (2004). Biosens Bioelectron 19(12): 1635.
- Luo Y.C., Do J.S., Liu C. (2006). Biosens Bioelectron 22(4): 482.
- Zhang F.F., Wang X.L, Li X.C., Li X.H., Wan Q., Xian Y.Z. Anal Bioanal Chem 382(6) 1368–1373 (2005).