Abstract
The objective of this study was to establish an effective therapy system for wound management. The present work describes preparation of metronidazole/serratiopeptidase loaded alginate microspheres by emulsification method. In vitro characterizations like particle size analysis, % yield, % encapsulation, and in vitro release were carried out. Wound healing assessment was determined by physical, histological, and biochemical methods. Wound healing performance of experimental formulations was compared with marketed product in rabbits. Result obtained for alginate microspheres showed good loading efficiency with spherical in shape. Experimentation suggests wound healing is improved by using serratiopeptidase and metronidazole in full thickness wounds in rabbits.
INTRODUCTION
In the integration of biotechnology and wound management, we have the opportunity to co-apt the frontiers of realism with those of idealism. With the extensive use of topical antibacterial therapy such as sulfamylon cream, gentamycin ointment, etc., there has been a consequent delay in the separation of full thickness eschars [Citation1]. Most attempts at early removal of eschar have been of mechanical or an enzymatic nature [Citation2]. With mechanical means there may be problems because of the blood loss associated with excision of huge eschars, and it is difficult to accurately assess the depth of every contaminated wound. Enzymatic means have also been unsuccessful because of the unavailability of specific proteolytic enzymes that would adequately debride all the necrotic tissues [Citation3]. Considering all, serratiopeptidase is the most suitable enzyme for tissue debridement. Clinical studies show that serratiopeptidase induces fibrinolytic and anti-inflammatory activity in a number of tissues. To maintain therapeutic blood levels, serratiopeptidase needs to be administered repeatedly. Therefore the objective of this study was to develop a sustained release depot delivery system for the enzyme that would decrease repeated administration and to establish a safe and effective therapy system that would prove superior in terms of patient compliance, therapeutic efficacy, and defined drug delivery. Metronidazole, which has been widely used for many years in the treatment of trichomoniasis, amoebiasis and giardiasis, has been shown to be active against anaerobic bacteria [Citation4]. The local use of metronidazole makes it possible to reach high metronidazole concentration in the tissue in the absence of a toxic effect of the drug resulting from its small doses. The spectrum of activity of metronidazole is very narrow in that it encompasses only anaerobic bacteria and is not active against the aerobic forms that are commonly involved in wound sepsis. However, in the present study, metronidazole is used along with serratiopeptidase that may be improving the activity of metronidazole for better and faster wound healing efficacy. In the present study alginate microspheres as a carrier for serratiopeptidase and metronidazole were developed by internal gelation technique with different concentration of calcium chloride [Citation5,Citation6]. Specific attention will focus on the process of selection of cross linkers, i.e. calcium chloride improves the proteolytic activity of serratiopeptidase.
MATERIALS
Serratiopeptidase and metronidazole were supplied by Solisto-Pharma Sagar; the marketed formulation (povidone iodine) and xylocaine were purchased from a local market. Sodium acetate, citric acid, and glacial acetic acid were purchased from Loba Chemicals. Cloramin–T reagent and dimethyl amino benzyladehyde were obtained from IICT Hydrabad. All other chemicals used were of analytical grade.
METHODS
Preparation of Alginate Microspheres [Citation7,Citation8]
0.8% w/v calcium carbonate dispersion was transferred into sodium alginate solution to give a final alginate concentration of (2% w/w, 2.5% w/w & 3% w/w). The alginate solution containing calcium carbonate was dispersed in isooctane containing 2.54 gm of span 85 using a mechanical stirrer at different rpm (1000, 1200 & 1500). Five grams solution containing 1.36 gm of tween 85 was added after 10 min of stirring. 1.1 ml of glacial acetic acid was added to release the calcium ions for cross-linking the alginate to produce microspheres. The alginate microspheres produced, were collected by gravitational filtration and redispersed in 30 ml of distilled water, and further hardened with 50 mL of cross-linking solutions (40% w/v CaCl2, 30% w/v CaCl2 and 20% w/v CaCl2) for 20 min. Microspheres were collected and re-dispersed in 30 ml of distilled water before freeze-drying.
Characterization of Alginate Microspheres
Particle size analysis [Citation8]. Optical microscopy was used to evaluate the morphological characteristics of the microspheres. Particle size distribution of microspheres was examined using standard sieve no. 60. The particles that passed through sieve no. 60 were then measured microscopically with the aid of a stage, an eyepiece micrometer.
Yield of micro particulates and drug encapsulation efficiency [Citation9]. The yield of micro particulates was obtained by dividing the theoretical weight of polymer and drug used by the weight of the micro particulates obtained. The encapsulation efficiency was determined as follows. An accurately weighed (50 mg) amount of microspheres was dispersed in 10 ml of PBS pH 7.4. sonicated for 2 min. and centrifuge at 5000 rpm for 2 min. The absorbance of the supernatant was measured at 320 nm and 277 nm for metronidazole and serratiopeptidase respectively. Percent encapsulation efficiency has been shown in .
Table 1. Composition and properties of the alginate microspheres
In vitro drug release study [Citation9]. 100 mg microspheres were accurately weighed and dispersed in 10 ml distilled water. Suspension was taken in a hollow glass tube of one inch diameter, which was tied at one end with a treated cellophane membrane. This tube was clamped in a 100 ml of PBS (pH 7.4) and maintained at 37 ± 2°C on a hot plate magnetic stirrer. 5 ml sample was withdrawn at 2 hourly intervals up to 12 hours, followed by at 24 hours interval for 3 days. After each sampling the volume was replaced with an equal volume of fresh buffer. The drug content was determined spectrophotometrically at 320 nm and 277 nm for metronidazole and serratiopeptidase, respectively ().
Wound Healing Assessment
Preparation of full thickness wound model. Twenty male rabbits weighing about 1100 gms (1100 ± 200) were used. After shaving, a two centimeter square was marked on the mobile skin of the right flank lines approximately 5 millimeters apart. After intramuscular xylocaine (2%), four 2 cm. full-thickness skin defects including the panniculosus carnosus were created with fine scissors on the back of the animals, two on each side and equidistant from the midline. The nearest edges of the wounds were separated by a margin of 3 cm of unwounded skin.
After wounding, a calculated dose of serratiopeptidase, a combination of serratiopeptidase and metronidazole and marketed topical antimicrobial product, were applied on different wounds of the same animals, and then the wounds were covered with sterile gauze and fixed with circular adhesive bands and the control was left untreated. The animals had free access to water and standard laboratory pellet and were housed individually.. The dressing was changed every second day until complete wound healing. The capillary permeability was estimated by intravenous injection (via ear vein) of 0.5 milliliter of 1 percent Evans blue with prior injection of xylocaine (2%). The distribution and depth of staining were then noted macroscopically and observations made at 5, 10, 15 and 20 days.
Photodocumentation analysis [Citation10]. Starting from immediately after surgery, each () wound was photographed at a fixed focal distance at every five days interval. Dressings were soaked with sterile saline until they could be removed with minimal disturbance of the surface of the wound. The flank skin of each rabbit was photographed as soon as the dressing had been removed to record the gross appearance of the wounds. The relative extent of contraction of the control and experimental wounds was assessed qualitatively.
Figure 2. Photographs of wounds 5, 10, 15 and 20 days after surgery illustratating the changes in capillary permeability and wound contraction. (A) control; (B) reference; (C) serratiopeptidase; (D) serratiopeptidase and metronidazole.
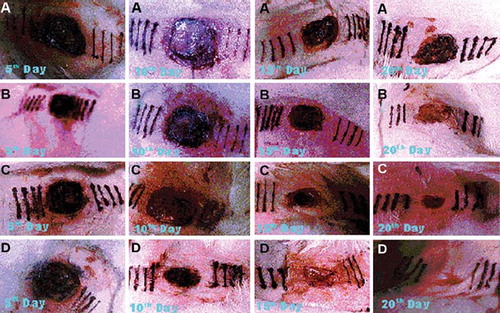
Wet weight and dry weight of skin of wound area [Citation11]. Wet weight was determined immediately after excision and dry weight after desiccation at 110°C in a vacuum in the drying chamber
Histological analysis [Citation12]. On the corresponding postoperative days specified in the observation period, uninjured skin surrounding each wound was incised 0.5 cm from the wound margin, and the wound together with the surrounding skin and a supporting layer of body wall musculature dissected free from surrounding tissue. Each excised sample was cut into two dorsoventrally and the unswabbed half fixed in neutral formalin solution (10%), embedded in paraffin wax, sectioned at 7 micrometer by a microtome, and finally the tissue section was stained with Van Geison stain. After light microscopy examination the wounds were given a histological score ranging from 1 to 20 with 1 corresponding to no healing and 20 corresponding to a scar with organized collagen fibers. The scoring scale was based on granulation tissue, collagen deposition and total cell content.
Biochemical Estimation
Assay of collagen and hydroxyproline [Citation13]. 19 to 20 mg of defatted tissue were transferred in stoppered tubes with 4 ml distilled water. The tubes were autoclaved for six hours. Tubes were then removed, allowed to cool, and the aqueous extract-containing gelatin was poured into a beaker. Thesamples were placed into glass ampoules and concentrated HCl added to provide a final acid concentration of 6 N.
Hydrolysis was achieved by heating for 6 to 12 hours at 15lb pressure in an autoclave. After cooling the hydrolysate was diluted to 10 ml with water and filtered. 1 ml of hydrolysate was diluted with 5.0 ml assay buffer, followed by the addition of 2.5 ml of chloramine-T feagent and was kept for 20 minutes at room temperature. 2.5 ml of freshly prepared dimethylaminobenzaldehyde reagent was added and mixed thoroughly. We incubated the tubes at 60°C for 15 min and cooled under tap water. The absorbance was measured at 550 nm within 45 minutes using a CINTRA 10 UV-Visible Spectrometer. % collagen was determined using the following formula:
% Collagen = % hydroxyproline × 7.1
RESULTS
Development of Formulation
In this study, alginate microspheres were produced by the internal gelation method using a modified emulsification technique. Although the amounts of calcium carbonate used were more than sufficient for the intended function, they failed to produce microspheres of sufficient strength. Carbon dioxide was liberated from calcium carbonate in the presence of an acid produced porous microsphere matrix.
It was found that alginate microspheres could be successfully produced by the emulsification method using calcium chloride. The microspheres obtained using 20% calcium chloride consisted of lumps with a size of about 1 mm. 30% calcium chloride produced microspheres that could be easily harvested. These microspheres generally had smaller mean size and markedly lower degree of aggregation compared with 20% and 40% calcium alginate microspheres. They had the largest fraction (55%) in the size range of 60μm, while 40% calcium chloride alginate microspheres had the largest fraction (60%) in the size range of 90–100 μm. Above 40% calcium chloride, the mean size of the microspheres increased proportionally with an increase in molar percentage of calcium cations used.
Particle size and size distribution
Microspheres mean diameter depended on the stirrer rotational speed during the second stage of emulsification. Depending on the alginate concentration, mean diameter could be varied between 100 and 400 μm over a range of rpm between 1000 and 1500. Less concentrated alginates resulted in larger diameters for rotational speeds up to 1500 rpm. The observations have been presented in .
Yield of micro particulates and drug encapsulation efficiency. The yield of microparticulates obtained was only about 80%. The loss may be attributed to the polymeric solution sticking to the glass beaker used in transferring the solution to the precipitating medium. Microencapsulation efficiency was determined as the ratio of actual quantity of drug encapsulation within the microspheres to the theoretical quantity of drug added during emulsification phase and is expressed as percentage in . Encapsulation efficiency of M2/S2 batches varied from 25–30% while efficiency of the M3/S3 batches varied from 65–80%. A possible reason for this difference may be due to uncharacteristic gelling efficiency of 20% calcium chloride as cross linkers in M2/S2 batches. Particle size range of M3/S3 formulations were smaller than M2/S2 formulations, and provide larger surface area for drug absorption. High solubility of the drug may be a reason for overall low encapsulation efficiency.
In vitro release study. The drug release profiles of the microspheres indicate that drug release was governed by diffusion of drug molecules through liquid-filled channels of the matrix. It was found that the rate of drug release also decreased with an increase in the molar percentage of calcium ions used. M1/S1 formulations were produced from a higher molar percentage of calcium ions than M3/S3 formulations; their release rate was only slightly lower. This could be attributed to the much larger size and hence smaller specific surface area for drug release from microspheres. Nevertheless, the results suggested that a larger proportion of calcium ions produced a more extensively cross-linked and less permeable alginate matrix. The percentage of calcium ions is an important factor affecting drug release. The use of higher percentage calcium ions would cause formation of lumps instead of microspheres. Release profile is graphically presented in .
Wound Healing Assessment
Photodocumentation analysis. This experiment shows that the contraction force is exerted by factors within the wounded area. In the initial stages the scab is responsible for an early rapid contraction and this is probably due to simple dehydration. After the first five days there is a further abrupt onset of contraction, predominant in the combination therapy over others. This may be attributed to the enzymatic debridement of the collagen that may act as chemotactic factors resulting in attraction of fibroblast cells to the wound site, and in turn produce biomaterials for dermal repair. This can be related to the decreased capillary permeability of Evans blue and dehydration of the wound bed. With combination therapy contraction it proceeds at a rapid rate until epithelial closure is nearly complete when it shows. The wound contraction is depicted in .
Wet weight and dry weight of skin of wound area. The total water content varied with time in the same way as total wound contents. The concentration of water in the whole tissue showed only a 4 percent linear fall from 84 percent to 76 percent at 20 days. Water resorption is therefore not a cause of wound contraction.
Histological analysis. During the histological examination, all wounds showed more or less similar patterns (). In general, the wounds had partial epithelization, inflammatory cell predominance with few fibroblasts, and sparse collagen deposition were noticeable, especially at the wound centers. There were no significant differences between histological scores of the wounds, except the control. Wound healing was accepted as complete when the wound area was totally covered by epithelization and contraction. All wounds healed between the 20 to 25 days, expect the control where complete recovery occurs 40 days after surgery. The wound healing time in combination reduced significantly when compared with others. However, there was no significant difference between serratiopeptidase and marketed treated wounds.
Figure 3. Photomicrographs of histologic sections of 5, 10, 15 and 20 days old wound. (A) control; (B) reference; (C) serratiopeptidase; (D) serratiopeptidase and metronidazole.
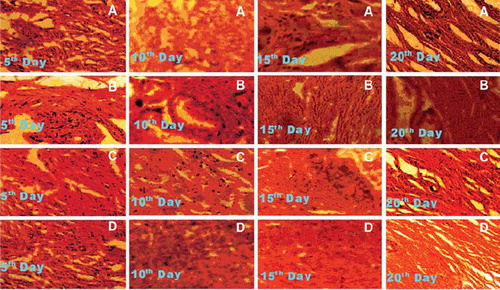
Biochemical estimation. The total amount of collagen began to rise sharply at five days to a maximum at 15 days; then the amount fell rapidly in all the wounds. There is an irreversible correlation between the total mass and the collagen content; it indicates that collagen represented a smaller proportion of the total solids present when total wound content was maximum then it did later when total content fell. A lack of correlation between the contraction process and the total amount of collagen in the wound was evident, as shown in .
Table 2. Distance (cm) between the wound edge and the line two centimeter apart from the wound at different intervals
Table 3. Collagen (mg) content per 100 mg of the wound bed
The unexpectedly rapid decrease in total amount of wound contents after t15 days may have either led to resorption of new-formed tissue or to its being covered by the advancing skin edges or a combination of both.
DISCUSSION
The formulation was optimized on several variables; drug-loading efficiency is a critical aspect for the selection of the desired formulation. Significantly less clumping was observed when the microspheres were freeze-fried, as compared to oven-dried. Additional crosslinking with calcium chloride solution produced less clumping of the microspheres. The microspheres, produced with 40% calcium chloride and 20% calcium chloride, showed less clumping than those produced with 30% calcium chloride. The microspheres with 20% calcium chloride produced distorted microspheres with a higher degree of clumping, and more porous and weaker microspheres matrix. Encapsulation efficiency of M2/S2 batches microspheres varied from 20–25%, M1/S1 bathes microspheres varied from 60–65%, while efficiency of the M3/S3 batches microspheres varied from 65–80%. Microspheres mean diameter depended on the stirrer rotational speed during the second stage of emulsification. Depending on the alginate concentration, mean diameter could be varied between 100 and 400 μm over a range of rpm between 1000 and 1500. A possible reason for higher encapsulation efficiency in 30% calcium chloride may be due to smaller particle size, hence larger specific surface area for drug absorption. High solubility of the drug may be a reason for overall low encapsulation efficiency of experimental formulations. The drug release profiles of the microspheres indicate that drug release was governed by diffusion of drug molecules through liquid-filled channels of the matrix. It was found that the rate of drug release also decreased with an increase in the molar percentage of calcium cations used, as reported by Chan et al. (2002); microspheres cross-linked by different ratio of cross-linker showed different morphology and slower drug release. Although M2 and S2 formulations were produced from lesser molar percentage of calcium cations than M3 and S3 formulations, exhibit slightly delay release rate than M3 and S3. This could be attributed to the larger size and hence smaller specific surface area for drug release from microspheres. Nevertheless, the results suggested that a larger proportion of calcium cations produced a more extensively cross-linked and less permeable alginate matrix. The percentage of calcium is an important factor affecting drug release. Microspheres did not form at low concentration of sodium alginate solution. A highly concentrated solution made the dropping process difficult and microspheres could not readily be formed. Therefore, the concentration of sodium alginate was chosen as 2.5% w/w [Citation8].
During the histological examination, all wounds showed more or less similar patterns. In general, the wounds had partial epithelization, inflammatory cell predominance with few fibroblasts, and sparse collagen deposition noticeable, especially at wound centers. There were no significant differences between histological scores of the wounds, except the control.
Wound healing was accepted as complete when the wound area was totally covered by epithelization and contraction. All wounds healed between the 20 to 25 days, except the control, where complete recovery occurs 40 days after surgery, which was evident from the . Wound healing time in combination therapy was significantly reduced in comparison with other. However, there was no significant difference between serratiopeptidase and marketed treated wounds.
In conclusion, experimentation suggests wound healing is improved by using serratiopeptidase and metronidazole in full thickness wound in rabbits. Specific enzymatic debridement increased neo-vascularization and increased chemotaxis for fibroblasts and macrophages and effective contraction are the important causes for this finding. Our studies reveled that specific enzymatic debridement is a selective and specific process to degrade immature collagen in wound matrix. Further ultra structural investigations are needed to elucidate the exact mechanisms of the effect of exogen debriding enzyme on the wound healing process.
Declaration of interest: The authors report no conflicts of interest. The authors alone are responsible for the content and writing of the paper.
REFERENCES
- Niedner, R., Schoof, E. (1986). Inhibition of wound healing by antiseptics. Br. J. Dermatol 115 (suppl 31): 41–44.
- Vanscheidt, W., Weiss, J. M. (1994). Proteolytic Enzymes and Wound Healing. Berlin: Springer-Verlag; 59–73.
- Donati, I., Maglino, E., Colonna, M., Garbin, S. (1994). Proteolytic Enzyme and Wound Healing. Berlin: Springer-Verlag; 31–47.
- Wiist, J. (1977). Antimicrobial agents. Chemother 11: 631–37.
- Poncelet, D., Lencki, R., Beaulieu, C., Halle, J. P., Neufeld, R. J., Fournier, A. (1992). Production of alginate beads by emulsification/internal gelation. I. Methodology. Appl. Microbiol. Biotechnol 38: 39–45.
- Quong, D., Neufeld, R. J., Skjak-Break, G., Poncelet, D. (1998). External versus internal source of calcium during the gelation of alginate beads for DNA encapsulation. Biotechnol. Bioengg 57: 438–446.
- Poncelet, D., Poncelet, DeSmet B., Beaulieu, C., Huguet, M. L., Fournier, A., Neufeld, R. J. (1995). Production of alginate beads by emulsification/internal gelation. II. Physicochemistry. Appl. Microbiol. Biotechnol 43: 644–650.
- Chan, L. W., Jin, P. W. S., Heng, F. (2002). Production of alginate microspheres by internal gelation using an emulsification method. Int. J. Pharm. 242: 255–258.
- Stockwell, A. F., Davis, S. S. (1986). In vitro evaluation of alginate gel systems as sustained release drug delivery systems. J. Cont. Rel. 3: 167–175.
- Abercrombie, M., Flint, M. H., James, D. W. (1956). Wound contracture in relation to collagen formation in scorbutic guineapigs. J. Embryol. Exp. Morph. 4: 167–175.
- Paul, H. E., Paul, M. E., Taylor, J. D., Marsters, R. W. (1948). Inhibitory effects of dl-alanine, 1-glutamic. Arch. Biochem 17: 269–274.
- McConkey, B., Walton, K. W., Carney, S. A., Lawrence, J. C., Ricketts, C. R. (1967). A study of histological characteristics and biosynthesis of dermal collagen. Ann Rheum Dis 26: 219–225.
- Murthy, K. N., Reddy, V. K., Veigas, J. M., Murthy, U. D. (2004). Study on wound healing activity of Punica granatum peel. J. Med. Food 7(2): 256–9.