Abstract
OxyVita-zero-link polymerized hemoglobin (OxyVita Hb) is a novel generation hemoglobin based oxygen carrier (HBOC). Our focus in this paper is to address the question of “Why is OxyVita Hemoglobin different than the previous generation of HBOCs?” Several critical related topics will be discussed: 1) OxyVita's unique chemistry; 2) the introduction of a viable powder form of OxyVita hemoglobin for dissolution in IV water or other IV fluids; 3) the physiochemical characteristics of OxyVita hemoglobin preparations; 4) the ability to formulate different products based upon specific applications; and 5) the important storage properties essential for use in a wide range of geographical locations.
INTRODUCTION
For the past three decades, most research efforts directed towards the design of an alternate therapeutic oxygen carrier for use in the absence of whole blood or packed red blood cells for the delivery of oxygen in emergency or other clinical applications have focused upon the development of acellular (cell-free) hemoglobin-based oxygen carriers (HBOC). Although many of the HBOC employed in phase I to phase III studies have been utilized in an attempt to be effective in the delivery of oxygen in specific clinical situations, continued observations of oxidative and cellular damage associated with their use have prevented full FDA regulatory approval for their clinical use [Citation1–4]. The recent meta-analysis [Citation5] carried out on many of these first and second generation HBOCs based upon available clinical data demonstrated a strong association with a substantial increased risk of subject death and myocardial infarctions (MI).
The evolution of HBOC development over the years has seen important design considerations incorporated into new molecules with the intention of trying to address the many factors responsible for these negative clinical results. Incorporation of new design characteristics began with the introduction of an intramolecular cross-linking approach (HemAssist, M.wt. 64 kD). This was followed by several different approaches involving intermolecular cross-linking polymerization using glutaraldehyde to cross-linked bovine hemoglobins, M.wt. >150 kD, (Hemopure, human application, and Oxyglobin, veterinary applications), raffinose cross-linked human hemoglobin (Hemolink), M.wt. >100 kD, and pyridoxylated glutaraldhyde cross-linked human hemoglobin (PolyHeme) M.wt. >120 kD. In addition, several Optro recombinant human hemoglobins (M.wt. 64 kD) were also created. These approaches were an attempt to eliminate the dimerization of stroma free hemoglobin (small molecular radii), which resulted in rapid elimination from the circulatory system and stress on the glomerulus and kidneys. Large molecular weight cross-linked human and bovine hemoglobins were designed with the advantage that they exhibit limited access to the vascular space and produced increased retention time compared to the intra-cross-linked hemoglobin tetramers. The intention of this approach here is to limit hemoglobin extravasation associated with NO binding and a concomitant increase in vasoconstriction and mean arterial pressure (MAP) [Citation6,Citation7]. In the late 1990s, Hemospan (selected pegylation, M. wt. ∼90 kD) was introduced to reduce extravasation mitigating vasoconstriction and maintain mean arterial pressure [Citation8]. These different chemical modifications resulted in several HBOCs with no dimerization observed and increased molecular sizes to alleviate the earlier clinical problems of rapid elimination from the circulation. Although these modifications did demonstrate reduced extravasation with maintenance of normal mean arterial pressure, these same HBOCs have unfortunately continued to exhibit oxidative and cellular damage during different phase I, II, and III studies [Citation9,Citation10].
Having evaluated these results in regards to the physical and chemical characteristics of these earlier molecular species and their resultant toxicity and oxidative cellular damage, it became apparent that a new design approach for the production of a safe and effective HBOC needed to be developed. This new design and approach was initially carried out in the laboratory of Professor Enrico Bucci at the University of Maryland [Citation11] using a novel zero-linked polymerization technology.
METHODS AND EXPERIMENTAL APPROACHES
Preparation of Hemoglobin
Fresh bovine blood was obtained from USDA-approved facilities providing appropriate documentation on the history of a herd. The purified bovine hemoglobin was produced through a process of red cell lysis using a phosphate buffer, pH, followed by a series of low-speed and high-speed centrifugation to remove cell debris. The isolated hemoglobin was used immediately for production of the polymerized OxyVita Hb product.
Polymerization Process
The zero-linked polymerization process is fundamentally governed by the use of a chemical “activator” that allows for the intermolecular polymers to be produced. The water soluble carbodiimide EDC [1-ethyl-3-(3-dimethylaminopropyl) carbodiimide] is used to activate the side chain carboxylate groups of the hemoglobin surface, resulting in a highly reactive and short-lived O-acylisourea derivative. The complex is formed from the C-terminal and Glu and Asp side chain carboxylate groups. These activated species react with the side chains of the lysyl residues of another hemoglobin molecule to form a stable amide bond (covalent), referred to as a pseudo-peptide bond [Citation11]. Control of the rate and time of polymerization allows for regulation of the average molecular weight sizes of each preparation.
Physicochemical Characterization Methods
a) Anion-Exchange and Size Exclusion Chromatography: through the use of Anion-Exchange (DEAE) and Size Exclusion (Fractogel, 20–40 μm) chromatographic methods (including HPLC), separation, purification and isolation of discrete molecular weight fractions were achieved for the zero-linked hemoglobin polymerization products.
b) Light Scattering:The average molecular weight and hydrodynamic radius of the solution preparations was estimated using a Dyna-Pro 801 (Protein Solutions, Inc.) dynamic light scattering molecular size detector. Light scattering methods reflect the contribution of the largest molecules in solution (weight-average molecular weight).
c) Oxidation Studies: autoxidation-continuous spectra were recorded from 700 to 490 nm using a HP diode array recording spectrophotometer at specified time intervals. All measurements will be carried out at 37°C. The rate and extent of methemoglobin formation (oxidized form) was determined by the observed changes in the A576/A540 ratio. This method of analysis eliminates light scattering effects that may result from the development of turbidity in the sample.
d) Conformational Stability Studies: determinations of the conformational stability of the different preparations of OxyVita Hb (liquid and powder) were carried out using isothermal solvent perturbation at 37°C. Urea solutions of 1 to 9.5 M concentration were prepared by volumetric dilution using a 10 M stock solution. Using a fixed protein concentration, spectral measurements in the Soret region (450–350 nm) were recorded after 30 minute equilibration. The unfolding of each hemoglobin as a function of increasing urea concentration was followed by changes in absorbance at the Soret maximum wavelength. The unfolding behavior of OxyVita Hb was compared to two natural acellular polymerized hemoglobins isolated from terrestrial (Lumbricus terrestris) and marine (Arenicola marina) invertebrates.
RESULTS AND DISCUSSION
Development of OxyVita Hemoglobin: Unique Chemistry
OxyVita Hb is produced through a polymerization reaction of purified hemoglobin using activators as described in the methods section, which are removed after initial phase of synthesis. This procedure eliminates any chemical linkers remaining in the product, eliminating possible side reaction concerns, such as reversibility and decomposition due to weak chemical bonds, dependency on temperature and pressure, and residual toxicity. The strong pseudo-peptide bonds initiated by the activators between the hemoglobin molecules within the OxyVita Hb polymer, coupled with a thorough physicochemical characterization of the polymer, allow critical control over the production of several different molecular weight products. The preparation of the zero-linked polymeric hemoglobin begins with either cross-linked human or bovine tetramers being polymerized by intermolecular cross-linking utilizing an activation process involving the carboxylic surface residues of these tetramers forming the “zero-linked” molecular species. The polymerization process is basically through surface residues of the hemoglobins. Selective modulation of this reaction allows for specific lysine residue involvement due to the differential pKs exhibited by these residues at pH 6.7. Further modification of the zero-linked approach by OXYVITA, Inc. has resulted in several important physical and chemical properties being incorporated into this “super-polymeric” oxygen carrier leading to a lower P50 (6 mmHg) and a Hill coefficient of n = 1.1–1.2 (non-cooperativity). This lower P50 is consistent with the recently recognized autoregulation of oxygen delivery within the microcirculation [Citation12,Citation13].
The production method employed allows for enhanced manufacturing and quality control resulting in sustained identical batch to batch preparations with a mean molecular weight of 17 MDa (light scattering) and methemoglobin levels of less than 3%. This distinct polymerization process allows for the production of a range of molecular size molecules that may have discrete clinical applications. Present technology allows for the use of any mammalian blood (tetrameric hemoglobin) as the starting material for the hemoglobin source.
Powder Form of OxyVita Hemoglobin
A very significant breakthrough for potentially greater wide spread application of OxyVita Hb is the production of a lyophilized form of this polymerized hemoglobin.
Presently the powder form of OxyVita Hb is produced by specific modification of a dehydration process (patented) using a computer controlled Virtis lyophilization system. This process results in a homogenous amorphous powder that can be chemically adjusted (variable electrolytic salts and other soluble enhancing compounds) to be soluble in different clinical infusion media. Solubility of this powder form has resulted in a hemoglobin solution exhibiting identical characteristics as found with the initial liquid preparation. The advantage of this powder preparation is the absence of a need for any storage facility for its long-term availability and range geographical and climatic usage. Presently, testing to improve the solubility of the lyophilized OxyVita Hb is undergoing further studies.
Physical and Chemical Properties of OxyVita Hemoglobin
clearly demonstrates, using a wide range of physical and chemical analysis, that the polymeric hemoglobin constituting the OxyVita Hb solution, the Small Volume Resuscitation Fluid, and the OxyVita powder are equivalent in all their properties. This polymeric hemoglobin has been specifically modified through chemical steps resulting in a “super-polymer” hemoglobin with a molecular weight of 17 MDa and an average hydrodynamic radius of 360 Å (). An extensive study by Matheson et al. [Citation14] on the vascular response to infusion of this new HBOC, which incorporates the necessary structural and chemical properties, resulted in the elimination of this hemoglobin's extravasation through vessel walls coupled with a dramatic reduction in vasoconstriction due to hemoglobin scavenging of NO, effectively maintaining normal MAP. This study was carried out using anesthetized and awake cats. The large radius of this molecule also allows for greater retention time in the circulatory system with a half-life of 8–12 times longer than unmodified hemoglobins. Initial animal studies with OxyVita Hb resulted in no observable vascular extravasation as indicated by its absence in the renal hilar lymph after exchange transfusion experiments [Citation14]. In addition, no evidence of this hemoglobin was found in the urine of these experimental animals [Citation15] as demonstrated from spectral analysis (), which indicated no evidence of the 540 nm and 576 nm bands associated with the presence of oxyhemoglobin. This behavior is consistent with our present understanding of the complex nature of the mechanism of intercellular extravasation through endothelial capillary walls associated with a wide range of cellular pore sizes [Citation16]. Small pores (radius = 30–35 Å) allow dimeric hemoglobins (radius = 24 Å) to pass through, while tetrameric hemoglobins (radius = 32 Å) are more hindered from movement across the membrane and large pores (radius = 200–250 Å) allow lower molecular weight polymerized hemoglobins to extravasate, leading to NO binding and an increase in MAP. Additional permeability selection is associated with an array of negative charges on the epithelial wall reducing the interaction with OxyVita Hb due to its negative surface charges. The relative hydrodynamic viscosity at 6g% hemoglobin is 1.2 times higher than plasma and exhibits a colloidal osmotic pressure of 3 mmHg (in latctated Ringer, pH 7.4, 23°C) approximately 1/10 that of plasma [Citation14]. This important role of molecular size has also recently been examined by Cabrales et al. [Citation17] in a study wherein increasing the extent of T-state polymerization of bovine hemoglobin was demonstrated to correlate inversely with a decrease in hypertensive response and a lowering of vasoconstriction in Golden Syrian hamsters.
Table 1. Physicochemical Properties of OxyVita Hb.
Figure 1. Molecular size: determination of molecular size homogeneity by HPLC (A) and hydrodynamic radius from dynamic light scattering (B and C).
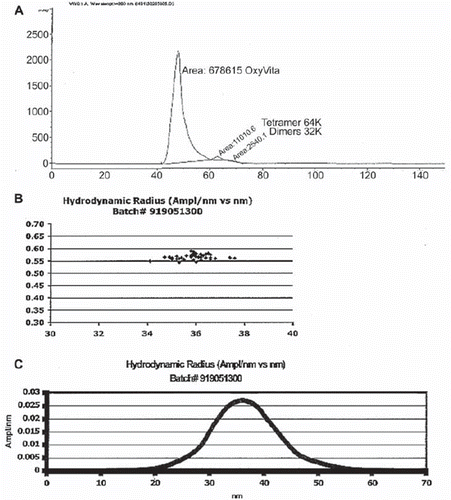
Figure 2. Urine analysis 5 hours after OxyVita Hb infusion using visible spectroscopy. No spectral bands at 540 nm and 576 nm associated with the presence of oxyhemoglobin.
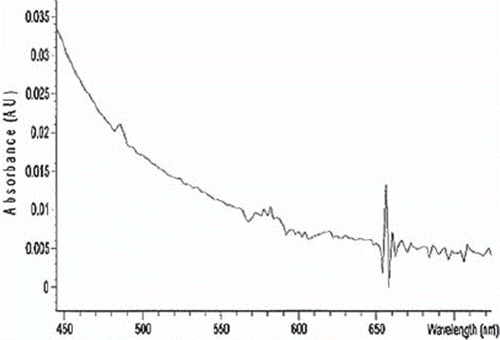
All of these physical and chemical properties as described above must ultimately be reflected in the structural and redox stability of this HBOC necessary for a safe and effective therapeutic oxygen carrying and delivery system. Structural integrity of the “super-polymeric” OxyVita Hb was determined by isothermal unfolding studies at 37°C using urea as the perturbant of the secondary and tertiary structure of this molecule (). The extraordinary resistance of this protein to molecular unfolding in either the liquid or powder form was established through analysis of the Soret spectral region (350–450 nm) due to its absorbance sensitivity to alterations within the heme environment [Citation18]. When heme proteins are exposed to a conformational solvent perturbant, such as increasing concentrations of urea, disruption of the many intramolecular interactions responsible for the maintenance of the native structure occurs. These unfolding studies provide evidence for both the structural integrity and redox stability as required for normal hemoglobin functionality. This functional requirement also involves the maintenance of the heme-iron in the reduced state necessary for the reversible binding/release of molecular oxygen and minimization of methemoglobin (heme-Fe+3) formation. This is particularly important since most acellular hemoglobins are especially susceptible to oxidation and denaturation.
Figure 3. Isothermal unfolding in the presence of urea (T = 37°C) of OxyVita Hb (liquid and powder preparations) compared to natural acellular hemoglobins isolated from Lumbricus terrestris and Arenicola marina. All solutions were equilibrated for 30 minutes prior to spectral runs [Citation15].
![Figure 3. Isothermal unfolding in the presence of urea (T = 37°C) of OxyVita Hb (liquid and powder preparations) compared to natural acellular hemoglobins isolated from Lumbricus terrestris and Arenicola marina. All solutions were equilibrated for 30 minutes prior to spectral runs [Citation15].](/cms/asset/fb7199a1-826c-4be6-b8e4-70612dcfe61b/ianb19_a_501753_f0003_b.gif)
In contrast to other acellular hemoglobins, OxyVita Hb undergoes little change in the Soret maximum (410 nm → 408 nm) during exposure to increasing concentrations of urea as compared to large alterations in Soret maximum [Citation18] for other acellular hemoglobins (413 nm → 398 nm). This significant blue shift in the Soret maxima, indicative of methemoglobin formation (heme-Fe+3), is associated with an increase in the extent of heme exposure upon molecular unfolding (). This increase in methemoglobin formation reduces the oxygen carrying capacity of any hemoglobin. OxyVita Hb resistance to oxidative changes under strong denaturing conditions is an important characteristic for any HBOC applications. Minimizing oxidative changes is vital for the introduction of an acellular hemoglobin into the circulatory system wherein increased membrane and tissue interactions occur. Oxidation associated with methemoglobin formation is often accompanied by hemichrome formation and eventual release of hemeiron responsible for cellular and tissue oxidative stress. In a recent paper, Jia and Alayash [Citation19] demonstrated that the zero-linked OxyVita Hb polymer showed little evidence of heme-iron loss, suggesting that its heme stability is related to the unique compact conformational structure of this large polymer. This observation is consistent with our studies demonstrating OxyVita Hb's significant resistance to structural unfolding [Citation18].
It is essential that any acellular HBOC be maintained in the reduced state (heme-Fe+2) to work effectively and safely as an oxygen carrier. Lacking the protective array of intracellular reducing enzymes normally present within the red blood cell, an HBCO ought to be capable of being reduced within the circulatory system for sustained functionality. In contrast to other HBOCs [Citation18], OxyVita Hb can be reduced significantly by ascorbic acid, an endogenous reducing agent of human plasma, providing additional redox stability within the circulatory environment [Citation18,Citation20].
Formulation of Different Products for Unique Applications
During the past five years, several different formulations of OxyVita Hb have been developed. These include liquid phase and powder formulations designed for various clinical applications.
In the liquid phase, OxyVita Hb has been formulated: (i) in a modified Ringer-Lactate medium at pH 7.4 with a hemoglobin concentration of 6g%, the primary formulation for this HBOC, and (ii) as a Small Volume Resuscitation Fluid (SVRF) with an elevated hemoglobin concentration of 9g% and 7% NaCl in a Ringer-Lactate medium [Citation21]. Under certain conditions, it is possible to include 5% albumin in this SVRF formulation. Customized formulations may be useful in addressing other specific clinical situations.
The unique powder form of OxyVita Hb has also been formulated wherein a fixed amount of powdered OxyVita Hb and the components (added as solids) of the modified Ringer-Lactate medium can be dissolved directly in a specified volume of water. This provides a hemoglobin concentration of 6g% and a pH 7.4 solution of this HBOC. A corresponding SVRF powder formulation has also been developed wherein the fixed amount of OxyVita Hb is adjusted to provide a final hemoglobin concentration of 9g% along with 7% NaCl and the components (added as solids) of the modified Ringer-Lactate medium, which is then dissolved directly in a specified amount of water. The SVRF formulations have an osmolality of 2100 mOsm/kg. These preparations can also undergo customized formulation if needed.
Unique Storage Properties of OxyVita Hemoglobin
Due to the unique chemical method of preparation of OxyVita Hb, this polymeric hemoglobin has the ability to withstand a wide range of temperature conditions. From –80°C to over 45°C, the inherent stability of this molecular species is unaltered as reflected by no changes in light scattering data, methemoglobin level and unfolding studies before and after exposure to this wide range of temperature. Under normal everyday operating conditions, the solution preparation of OxyVita Hb has a shelf-life of one year, if container is unopened, at ambient temperature (∼20°C) and can be stored for 21 days refrigerated if the container is opened (∼4–6°C). Unlike other HBOCs, OxyVita Hb can undergo storage at −80°C for up to 5 years. In a significant departure from the storage properties of other HBOCs, OxyVita Hb can undergo a freeze-thaw process through several cycles with no evident change to the physical characteristics of the polymer. The initial parameter that has been used to verify the integrity of the polymer during storage and the freeze-thawing processes has been a determination of any increase in methemoglobin content of the samples. All samples to date have been demonstrated to have methemoglobin levels less than 11%. Further testing will be carried out using dynamic light scattering and atomic force microscopy to analyze for any changes in molecular size distribution and shape, as well as monitoring of the P50 and Hill coefficient (n value, cooperativity activity) of these samples.
SUMMARY
This paper has addressed the fundamental reasons as to why OxyVita Hb, a new therapeutic oxygen carrier and delivery product, is different than previous generations of HBOCs. One important advantage that has enabled movement forward in the HBOC arena is the lessons learned from the historical work and evolution of earlier HBOC studies. The primary aspects in OxyVita Hb development and success to date are based upon its unique chemical technology and the recognition that molecular size, shape, negative surface charge [Citation14] and functional properties all matter. In summary, OxyVita Hb is differentiated from all other HBOCs presently being studied due to: 1) its chemistry: a unique zero-linked polymerization technology; 2) its well characterized physicochemical studies of its molecular properties and functional behavior; 3) its potential for long-term storage under extreme physical conditions; and 4) the ability to create different formulations for either the liquid or powder form of OxyVita Hb.
As OXYVITA, Inc. moves towards its long-term goal of FDA approval for its products for specific clinical situations, several important pathways have opened up to expedite this process. The most recent pathway is the signed 2009 CRADA agreement (Cooperative Research and Development Agreement) with the U.S. Naval Medical Research Center, Bethesda, Maryland. This study calls upon the Navy to perform final pre-clinical studies with OxyVita Hb in anticipation of its inclusion in the Navy's RESUS program coupled with FDA approval for pre-hospital emergency treatment of hemorrhagic shock. These studies will be conducted by investigators at the Virginia Commonwealth University Reanimation Engineering Shock Center (VCURES) and supported by the Henry M. Jackson Military Medical Research Foundation. In addition, the U.S Army has a submitted contract for product to carry out collaborative studies with specific focus on applications of OxyVita Hb for the treatment of: a) Sickle Cell Anemia, b) CO poisoning, and c) high altitude adaptation. Other requests for product have come from scientists interested in the enhancement of: 1) stem cell survival times, and 2) organ storage time for transplant operations.
Declaration of interest: The authors report no conflicts of interest. The authors alone are responsible for the content and writing of the paper.
REFERENCES
- Lee, R., Neya, K., Svizzero, T.A., Vlahahas, G.T. (1995). Journal of Applied Physiology 78: 236–246.
- Alayash, A.I., Cashon, R.E. (1995). Molecular Medicine Today 1: 122–127.
- Alayash, A.I. (1999). Nature Biotechnology 17: 545–549.
- Hirsch, R.E., Harrington, J.P. (2000). Einstein Quarterly Journal of Biology and Medicine 17: 113–123.
- Natanson, C., Kerns, S.J., Lurie, P., Banks, S.M., Wolfe, A.W. (2008). Journal of the American Medical Association (April 28) E1–E9.
- D'Agnello, F., Alayash, A. (2000). Adv. Drug Deliv. Res. 40: 199–212.
- Olson, J.S., Foley, E.W., Rogge, C., Tsai, A.L., Doyle, M.P., Lemon, D.D. (2004). Free Radical Biology and Medicine 36: 685–697.
- Acharya, S.K., Freidman, J.M., Manjula, B.N., Intaglietta, M., Tsai, A.G., Windslow, R.M., Malavalli, S., Vandergriff, K., Smith, P.K. (2005). Artificial Cells, Blood Substitute, and Immobilization Biotechnology 33: 239–255.
- Alayash, A.I., D'Agnilo, F., Beuhler, P.W. (2007). Biochemical and Physiological Perspectives, Expert Opinion on Biological Therapy 7: 665–675.
- Winslow, R.M. (2008). Biochemica et Biophysica. Acta, Proteins and Proteomics, 1784: 1382–1386.
- Razynska, A., Bucci, E. (1998). Blood Substitutes-Present and Future Perspectives, Ch 21, pp. 265–279.
- McCarthy, M.R., Vandergriff. K.D., Winslow, R.M. (2001). Biophysical Chemistry 92: 103–110.
- Vandergriff, K.D. (2006). Blood Substitutes, Elsivier, Inc., London, pp. 60–71.
- Matheson, B., Razynska, A., Kwansa, H., Bucci, E. (2000). Journal of Laboratory and Clinical Investigation, 135: 459–464.
- Bucci, E., Razynska, A., Kwansa, H., Matheson-Urbaitis, B., O'Hearne, J.A., Utalowski, J.A., Koehler, R.C. (1996). Journal of Laboratory and Clinical Medicine, 128: 146–153.
- Rippe, B., Haraldson, B. (1994). Physiological Review 74: 163–219.
- Cabrales, P., Sun., Zhou, Y., Harris, D.R., Tsai, A.G., Intaglietta, M., Palmer, A. F. (2009). Journal of Applied Physiology 107: 1548–1558.
- Harrington, J.P., Kobayashi, S., Dorman, S.C., Zito, S.L., Hirsch, R.E. (2007). Artificial Cells, Blood Substitutes, and Biotechnology 35: 53–67.
- Jia, Y., Alayash, A. (2009). Biochemica et Biophysica Acta, Proteins and Proteomics, 1794: 1234–1242.
- Harrington, J.P., Wollocko, J., Wollocko, H. (2010). Artificial Cells, Blood Substitute and Biotechnology 38: 64–69.
- Reynolds, P.S., Barbee, R.W., Skaflen, M.D., Ward, K.R. (2007). Shock, 28: 45–52.