Abstract
A higher production of functional mast cells (MCs) can be generated by co-culturing cord blood-derived CD34+ cells with a human bone marrow stromal cell line under serum-free conditions supplemented with stem cell factor and IL-6. We addressed the question of whether the higher proliferation of MCs in this co-culture system might be due to the higher proliferation of MC progenitors. The stromal cell line increased the cell numbers of MC progenitors derived from cord blood-derived CD34+ cells, in a combination of cell-cell interactions between stromal cells and CD34+ cells, and as yet unidentified soluble factors derived from stromal cells.
INTRODUCTION
Mast cells (MCs) play a central role in type I allergic reactions, as these cells release several bioactive substances, particularly histamine and serotonin, when IgE bound to the high-affinity Fc epsilon receptor I (FcεRI) on the cell surface is cross-linked by a specific antigen [Citation1,Citation2]. Mcs play important roles not only in allergic reactions but also in homeostasis [Citation3,Citation4]. To understand such roles of MCs, substantial numbers of fully matured MCs are necessary. We previously demonstrated that co-culture of cord blood (CB)-derived CD34+ cells with human bone marrow stromal cells, immortalized by the transfection of a human telomerase catalytic subunit gene, in serum-free medium containing stem cell factor (SCF) and IL-6, generated a considerable number of functional MCs with a high basal level of FcεRI expression, compared with the yield obtained without stromal cells (namely, in a liquid culture) [Citation5]. However, the underlying mechanism by which stromal cells support the higher production of phenotypically or functionally mature MCs is uncertain.
To investigate this issue, we hypothesized that the higher proliferation of MCs obtained in our co-culture system might be due to the higher proliferation of MC progenitors. Here, we present data indicating that a bone marrow stromal cell line actually promotes the proliferation of MC progenitors derived from CB-CD34+ cells under serum-free conditions with SCF and IL-6, in a combination of cell-cell interaction between stromal cells and CD34+ cells and soluble factors derived from stromal cells.
MATERIALS AND METHODS
Preparation of Human CB Cells
The use of human umbilical CB cells in these described experiments was approved by the Committee of Hokkaido Cord Blood Bank. CB was obtained from normal full-term deliveries. Only CB units that did not fulfill the criteria for banking were used for this study. CB-CD34+ cells were prepared as described previously [Citation5]. Briefly, after the sedimentation of red blood cells by incubating CB samples with the same volume of 6% (W/V) hydroxyethyl starch dissolved in Ringer's solution (Veen-D Inj.; Nikken Chemical, Tokyo, Japan) at room temperature for 30 min, low-density (<1.077 g/mL) mononuclear cells were collected using Ficoll-Paque (Amersham-Pharmacia Biotech, Uppsala, Sweden). Further enrichment of CD34+ cells was performed using a magnetic activated cell sorting (MACS) CD34 Progenitor Isolation Kit (Miltenyi Biotec, Bergisch Gladbach, Germany) according to the manufacturer's instructions. The purity was then verified using flow cytometry. In all experiments, the purity of CD34+ cells was > 90%.
Co-culture of CB-CD34+ Cells with a Human Bone Marrow Stromal Cell Line
A human bone marrow stromal cell line (hTERT-ST) was established [Citation6]. One week before co-culture, 1×105 hTERT-ST cells, prepared using a long-term culture medium containing minimum essential medium-α, 12.5% horse serum (Gibco BRL, Rockville, MD), 12.5% fetal calf serum (Gibco BRL) and 1×10−6 M hydrocortisone (Sigma, St Louis, MO), were plated in 12.5 cm2 flasks in 2 mL long-term culture medium. When the stromal cell monolayers became confluent, they were washed with phosphate-buffered saline (PBS) three times to remove the serum components. Then, 1×104 CB-CD34+ cells in 2 mL serum-free medium (QBSF60 [Quality Biological Inc., Gaithersburg, MD]) containing 50 ng/mL recombinant human IL-6 and 100 ng/mL SCF (kindly provided by Kirin Brewery Co Ltd., Tokyo, Japan) were added to a flask to start the co-culture at 37°C in a humidified atmosphere of 5% CO2/95% air for the indicated periods. In a previous experiment, we confirmed that this cell line could survive in QBSF60 for at least 8–10 weeks. In the experiment to separate CD34+ cells and stromal cell monolayers, transwells with a 0.45 μm filter in 6-well plates were used. To test the role of β1-integrins in the proliferation of MC progenitors, an anti-β1-integrin antibody (SG19) or control IgG at 1 μg/ml was added to the co-culture at the start of culture. In liquid culture, cells were cultured in the same medium in the absence of stromal cells. In both cases, after one week of culture, 2 mL fresh medium containing the above-mentioned cytokines was added; thereafter, half of the medium was replaced with fresh medium every week.
Preparation of Conditioned Medium and Analysis of Biological Activity
The supernatant from the various culture conditions was collected and filtered to remove cellular debris. The resulting sample was designated as conditioned medium (CM). CM was obtained from co-cultures or liquid cultures at the various indicated times. CM from stromal cells alone was obtained from the confluent stromal cell monolayer cultured in QBSF60 medium supplemented with SCF and IL-6. The biological activity of each CM was analyzed by adding at a ratio of 25% (v/v) to a liquid culture containing CB-CD34+ cells. For some experiments, CB-CD34+ cells were cultured in the presence of sub-fractionated CM, heat-treated CM (56°C or 100°C for 45 min), or acid-treated CM obtained from co-cultures. For sub-fractionation, 15 mL CM from co-cultures was passed through a 10/30/50/100-kDa molecular mass filter (AMICON CENTRIPREP YM-10/YM-30/YM-50/YM-100; Millipore Corporation, Bedford, MA) by centrifugation. For acid treatment, CM was incubated with 0% (pH7.2), 1% (pH3.5), 2% (pH1.5), or 4% (pH0.5) trichloroacetic acid (TCA) for 10 min, followed by centrifugation. CM was then neutralized by dialysis and used for biological activity analysis.
Flow Cytometry Analysis
Aliquots of cells were stained with monoclonal antibodies in PBS/0.1% BSA at 4°C for 30 min, and analysis was performed using a BD LSR flow cytometer (BD Biosciences Immunocytometry System, San Diego, CA). The following monoclonal antibodies were used: FITC-conjugated CD34 antibody (Nippon Becton Dickinson Co. [BD], Tokyo, Japan), and PE-conjugated CD33, CD117 (c-kit) and APC-conjugated HLA-DR antibodies (BD). FITC- and PE-conjugated mouse IgG1 antibodies (BD) and APC-conjugated mouse IgG1 antibodies (BD) were used as isotype-matched controls. In flow cytometry analysis, dead cells were gated out first with propidium iodide staining and then with a forward versus side scatter window. CD34+CD33+DR− cells and CD117+CD33+DR− cells were designated as MC progenitors and MCs, respectively. After one to two weeks of culture, 5,000 events were collected. For longer culture conditions, at least 10,000 events were collected.
Statistical Analysis
The results are expressed as the mean ± standard deviation (SD). Significant differences were assessed using either Student's t-test or repeated measures ANOVA.
RESULTS
Proliferation of MC Progenitors and MCs
The cell numbers of MC progenitors (CD34+CD33+HLA-DR− cells) and MCs (CD117+CD33+HLA-DR− cells) were examined in either co-cultures with stromal cells or liquid cultures at various periods for up to 10 weeks (, ). In liquid cultures, the number of MC progenitors gradually declined right from the start of culture, and MC progenitors were not detected at 4 weeks. In co-cultures, however, the number of MC progenitors increased significantly from 0.8 ± 0.3 × 103 at Day 0 to 5.5 ± 1.6 × 103 at 1 week and 30.7 ± 12.1 × 103 at 2 weeks. The number of cells then gradually decreased as a function of the culture period. Nevertheless, MC progenitors were still detected even after 8 weeks of culture (0.1 ± 0.1 × 103 cells). At 10 weeks, MC progenitors were no longer detected. The highest number of MC progenitors observed in the co-cultures was noted at 2 weeks, at which time the cell number was 38.5-fold higher than on Day 0 and 182-fold higher than in the liquid culture at 2 weeks.
Figure 1. Proliferation of MC progenitors (A) and MCs (B) in the presence or absence of stromal cell lines. CB-CD34+ cells (1 × 104 cells) were cultured in the presence (closed diamond) or absence (open square) of a stromal cell line. Every week after the start of culture, the numbers of CD34+33+HLA-DR− cells (MC progenitors) and CD117+33+HLA-DR− cells (MCs) were counted using flow cytometry. Data are the mean ± SD of five experiments with five different CB samples (n=5). *, p < 0.01.
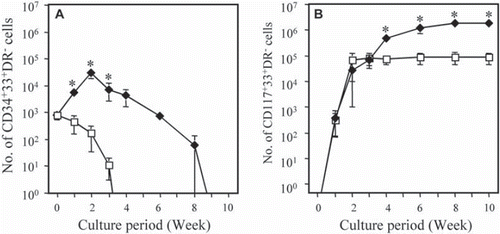
The number of MCs in the liquid cultures increased, reaching 6.7 × 104 cells at 2 weeks, and then was maintained at this level for up to 10 weeks. The number of MCs in the co-cultures also increased, reaching approximately 2.7 × 104 cells at 2 weeks. Thereafter, the number of MCs gradually increased for up to 10 weeks. From 4 to 10 weeks, the numbers of MCs obtained in the co-cultures were significantly higher (p<0.01) than those obtained in liquid cultures. At 10 weeks, the number of MCs obtained in the co-culture was 21-fold higher than in the liquid culture (180 × 104 vs. 8.7 × 104 cells).
Effect of Cell-cell Interactions and CM on the Proliferation of MC Progenitors
The roles of cell-cell interactions between stromal cells and CD34+ cells in the proliferation of mast progenitors were elucidated. shows that the presence of blocking antibody against β1-integrin (SG19) did not reduce the proliferation of MC progenitors, although the adhesion of MC progenitors and stromal cells was almost completely blocked. In contrast, the separation of CD34+ cells and stromal cells by transwells with a 0.45 μm microporous filter significantly inhibited the proliferation of MC progenitors; however, a significant increase of MC progenitors was still observed compared with liquid culture. We then examined whether CM from co-cultures had the ability to stimulate the proliferation of MC progenitors. shows that the cell number of MC progenitors was significantly higher under either liquid culture conditions supplemented with CM than without CM, but was lower under both Liq + CM conditions than under co-culture conditions. Thus, CM samples collected after different periods of co-culture had the ability to increase the proliferation of MC progenitors, although they did not completely replicate the effect of co-cultures.
Figure 2. Effects of cell-cell interaction of stromal cells and CB-CD34+ cells (A) and different conditioned media on the proliferation of MC progenitors in the absence of stromal cells (B). (A) An anti-β1-integrin antibody (SG19) or IgG (Control Ab) was added to cocultures of CD34+ cells and stromal cells at the start of culture. To separate CD34+ cells and stromal cells, transwells with a 0.45 μm filter in 6-well plates were used (TW). As controls, CB-CD34+ cells were cultured in the absence of stromal cells (liquid). After 10 days of culture, the number of CD34+33+HLA-DR− cells was counted. Data are the mean ± SD of three experiments with three different CB samples (n=3). *, p < 0.01. (B) Cell cultures were maintained for 10 days under four conditions: co-culture (Co), liquid culture without CM, and liquid culture supplemented with 25% (v/v) CM prepared from either the supernatant of co-cultures obtained after 2–3 weeks (2–3w) or 10–11 weeks (10–11w). After 10 days of culture, CD34+33+HLA-DR− cells were counted. Data are the mean ± SD of five experiments with five different CB samples (N=5). *, p < 0.01.
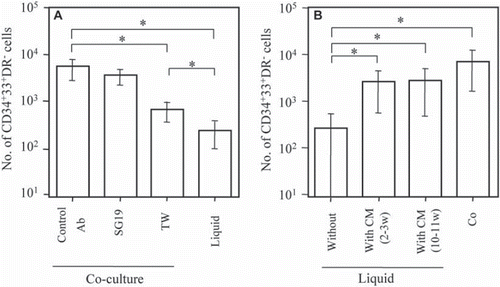
Characterization of CM Derived from Co-culture
CM derived from co-culture was fractionated according to molecular size using a molecular mass filter. No biological activity was found in filtrates obtained using molecular mass cut-off filters of 10 kDa, 30 kDa, or 50 kDa, whereas significant activity was found in the retentate of each filter. In contrast, biological activity equivalent to that in CM derived from co-cultures was found in the filtrate of a 100 kDa filter (). Biological activity was significantly (p<0.05) higher in the filtrate of the 100 kDa filter than in the retentate, although the retentate of this filter also exhibited substantial activity. Thus, proliferation-promoting activity in CM from co-cultures might be attributable to factors with a molecular mass of 50–100 kDa.
Figure 3. Characterization of CM derived from co-culture. (A) Effects of sub-fractionated CM obtained from co-cultures on the proliferation of MC progenitors. CM obtained from co-cultures after 1–2 weeks was sub-fractionated by passing through a 10/30/50/100-kDa molecular mass filter. CB-CD34+ cells in liquid culture were incubated with the filtrate or retentate from each filter at a ratio of 25% (v/v). As controls, CB-CD34+ cells were cultured in the absence (liquid) of stromal cells, in the presence (co-culture) of stromal cells, or in the CM obtained after co-culture for 1–2 weeks. After 10 days of culture, CD34+33+HLA-DR− cells were counted. Data are the mean ± SD of three experiments with three different CB samples (n=3). *, p < 0.01; #, p <0.05. (B) Effect of heat or acid treatment on the biological activity of CM obtained from co-cultures. CM was sub-fractionated with factors with a molecular mass > 50 kDa, as shown in . CB-CD34+ cells in liquid culture were incubated with either heat-treated or acid-treated CM at a ratio of 25% (v/v). As controls, CB-CD34+ cells were cultured in the absence (liquid) or presence (co-culture) of stromal cells. *, p < 0.01; #, p <0.05.
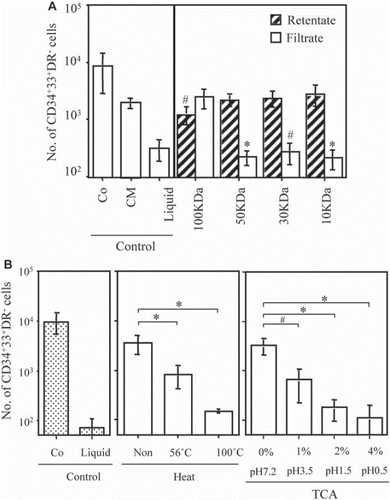
The biological activity of factors with a molecular mass >50 kDa in CM from co-cultures was significantly reduced by heat treatment at 56°C and was almost completely abrogated after treatment at 100°C (). In addition, TCA treatment of CM caused a significant reduction in the biological activity of these factors in a dose- or pH-dependent manner ().
Effect of CM Derived from Stromal Cells Alone on Growth of MC Progenitors and MCs
To further determine whether the CM from stromal cell cultures alone had the ability to stimulate the proliferation of MC progenitors, the supernatant from three culture conditions was obtained after one week as follows: CB-CD34+ cells were cultured in the absence of stromal cells (liquid culture), CB-CD34+ cells were cultured in the presence of stromal cell lines (co-culture), and stromal cells alone were cultured in serum-free complete medium (ST alone). As shown in , the biological activities of CM from co-cultures and CM from stromal cells alone were equivalent, suggesting that humoral factors were generated by stromal cells. This observation agreed with the equivalent biological activity of CM collected after different periods of co-culture (2–3 weeks vs. 10–11 weeks) ().
Figure 4. Effect of CM from stromal cells alone on the proliferation of MC progenitors (A) and MCs (B). (A) CM was obtained from liquid cultures of CB-CD34+ cells (liquid), co-cultures of CB-CD34+ cells and stromal cells (Co), or cultures of stromal cells alone (ST alone) for 1 week. Each CM was added (25% [v/v]) to liquid cultures of CB-CD34+ cells. As controls, CB-CD34+ cells were cultured in the absence (liquid) or presence (Co) of stromal cells. After 10 days of culture, CD34+33+HLA-DR− cells were counted. Data are the mean ± SD of three experiments with three different CB samples (n=3). *, p < 0.01. (B) Cell cultures were maintained under four conditions: co-culture (Co), liquid culture without CM, and liquid culture supplemented with 25% (v/v) CM prepared from either the supernatant of co-cultures (Co) obtained after 1 week or the supernatant of cultures of stromal cells alone (ST alone). After 8 weeks of culture, CD117+33+HLA-DR− cells were counted. Data are the mean ± SD of five experiments with five different CB samples (N=5). *, p < 0.01.
![Figure 4. Effect of CM from stromal cells alone on the proliferation of MC progenitors (A) and MCs (B). (A) CM was obtained from liquid cultures of CB-CD34+ cells (liquid), co-cultures of CB-CD34+ cells and stromal cells (Co), or cultures of stromal cells alone (ST alone) for 1 week. Each CM was added (25% [v/v]) to liquid cultures of CB-CD34+ cells. As controls, CB-CD34+ cells were cultured in the absence (liquid) or presence (Co) of stromal cells. After 10 days of culture, CD34+33+HLA-DR− cells were counted. Data are the mean ± SD of three experiments with three different CB samples (n=3). *, p < 0.01. (B) Cell cultures were maintained under four conditions: co-culture (Co), liquid culture without CM, and liquid culture supplemented with 25% (v/v) CM prepared from either the supernatant of co-cultures (Co) obtained after 1 week or the supernatant of cultures of stromal cells alone (ST alone). After 8 weeks of culture, CD117+33+HLA-DR− cells were counted. Data are the mean ± SD of five experiments with five different CB samples (N=5). *, p < 0.01.](/cms/asset/c80924b9-0db6-4240-abcf-6e5c597c625f/ianb19_a_501754_f0004_b.gif)
In addition to the effect on MC progenitors, the addition of CM derived from the culture of stromal cells alone to liquid culture of CD34+ cells significantly increased the cell number of MCs 8 weeks after culture (). This activity was the same as that of CM derived from co-culture, but was lower than that under the co-culture condition ().
DISCUSSION
In this study, we examined the possibility that the higher proliferation of MCs obtained in our co-culture system might be due to the higher proliferation of MC progenitors. MC progenitors are reportedly present in CD34+CD38+HLA-DR− cell populations in serum-containing culture systems [Citation7]; however, under our serum-free conditions, CD34+CD33+HLA-DR− populations were designated as MC progenitors. The reasons for this were as follows: (1) CD38+ cells were undetectable under our serum-free conditions; (2) CD34+CD33+ populations reportedly produce MC colonies in serum-containing culture systems [Citation7]; and (3) CD33 is reportedly expressed early during mastopoiesis using human CB, and the CD33-targeting drug gemtuzumab ozogamicin downregulates the SCF/IL-6-induced development of MCs from CB progenitors [Citation8].
Our data showed that the number of MC progenitors increased during the first 2 weeks of co-culture and then gradually decreased thereafter, continuing for up to 8 weeks. This transient increase and subsequent maintenance of the number of MC progenitors appeared to contribute to the increased proliferation of mature MCs for up to 10 weeks under co-culture conditions. In contrast, the number of MC progenitors in liquid cultures decreased from the very start of the culture period and became undetectable at 4 weeks. The number of mature MCs increased for up to 2 weeks and was sustained for up to 10 weeks in liquid culture. Thus, in liquid culture, the increase in mature MCs during the early stage (i.e., 2 weeks) seemed to reflect the differentiation and maturation of pre-existing MC progenitors among CD34+ cells at the start of culture. The lack of increase of MC progenitors in the liquid culture could not support the increase in mature MCs after 2 weeks of culture. Together, our results suggested that the increase and longer maintenance of MC progenitors under co-culture conditions was at least partly attributable to the larger number of functional MCs.
In this study, we have shown that the increased cell numbers of MC progenitors depended on cell-cell interactions between stromal cells and CD34+ cells, and on soluble factors derived from stromal cells. The adhesion of mouse bone marrow-derived MC progenitors is shown to be mediated by the binding of β1-intergrins, such as VLA4, VLA-5, and VLA-6, and their ligands, such as fibronectin and laminin [Citation9–11]. Our present data suggested that the adhesion of MC progenitors to stromal cells via β1-integrins was not essential for the proliferation of MC progenitors in co-cultures. This was also supported by our observation that the use of a culture flask coated with fibronectin, laminin, or collagen did not increase the cell number of MC progenitors in comparison to liquid culture (data not shown).
To search soluble factors other than SCF and IL-6, we carried out a semiquantitative protein array of CMs derived from stromal cells alone and co-culture for 1 week; however, there were no detectable levels of GM-CSF, IL-4, IL-5, IL-9, IL-10, IL-11, and β-NGF, which have been reported to have proliferative activities in conjunction with SCF on MC progenitors [Citation12–17] (data not shown). This was in agreement with our previous results in which CM derived from co-culture at 8 weeks failed to express these cytokines [Citation5]. IL-3 is also shown to have a survival effect on cultured mast cells [Citation12,Citation13]. Array data showed that all CMs derived from stromal cells alone, liquid culture alone, and co-culture for 1 week had similar levels of IL-3, suggesting that IL-3 was not a factor for proliferation-promoting activity in CM from stromal cells alone or co-culture (data not shown).
Multifunctional phospholipid mediators, such as lysophosphatidic acid (LPA) [Citation18] and shingosine-1-phosphate [Citation19], are also reported to induce the development of MCs from CB-derived progenitors. LPA is shown to act through LPA receptors and peroxisome proliferator-activated receptor (PPAR)-γ-dependent pathways to accelerate proliferation and differentiation of hMCs [Citation18]. In our preliminary experiments, VPC-32179 and VPC-32183, competitive antagonists of LPA receptors, and GW9662, a selective antagonist of PPAR-γ, had no effect on the proliferation of MC progenitors under co-culture conditions (data not shown). Hence, further experiments to identify heat- and acid-sensitive factors having MW 50–100 kDa in CM released from stromal cells are needed.
In this paper, we stated that stromal cells and CM from stromal cells promote MC/MC progenitor proliferation, based upon simply an increase in cell number. The increased cell number of MC/MC progenitors might be due to not only increased proliferation but also to decreased apoptosis. In fact, human intestinal fibroblasts have been shown to prevent apoptosis in human intestinal MCs, and this mechanism is independent of SCF, IL-3, IL-4, and NGF [Citation20]. In addition, human endothelial cells are reported to promote the survival of human MCs via cell-cell interactions of membrane-bound SCF/c-kit and vascular cell adhesion molecule-1/VLA-4 [Citation21]. In this regard, we collected MCs grown in a co-culture system at 8 weeks, and then further cultured them in serum-free complete medium in the presence or absence of stromal cells. We observed no difference in the survival of MCs regardless of the presence or absence of stromal cells (data not shown). Thus, the survival of MCs appears to be independent of the presence of stromal cells, but the effect on the survival (i.e., reduced apoptosis) of MC progenitors remained to be clarified.
In conclusion, a bone marrow stromal cell line increased the cell numbers of MC progenitors derived from CB-derived CD34+ cells under serum-free conditions with SCF and IL-6, which seemed to contribute to the higher proliferation of functional MCs. This increase in the cell numbers of MC progenitors required a combination of cell-cell interactions between stromal cells and CD34+ cells and as yet unidentified soluble factors derived from stromal cells.
Declaration of interest: The authors report no conflicts of interest. The authors alone are responsible for the content and writing of the paper.
REFERENCES
- Ishizaka, T., Ishizaka, K. (1984). Activation of mast cells for mediator release though IgE receptors. Prog Allergy 34: 188–235.
- Galli, S.J., Wershil, B.K. (1996). The two faces of mast cells. Nature 381: 21–22.
- Galli, S.J., Grimbaldeston, M., Tsai, M. (2008). Immunomodulatory mast cells: negative, as well as positive, regulators of innate and acquired immunity. Nat Rev Immunol 8: 478–486.
- Kalesnikoff, J., Galli, S.J. (2008). New developments in mast cell biology. Nat Immunol 9: 1215–1223.
- Yamaguchi, M., Azuma, H., Fujihara, M., Hamada, H., Ikeda, H. (2007). Generation of a considerable number of functional mast cells with a high basal level of FcεRI expression from cord blood CD34+ cells by co-culturing them with bone marrow stromal cell line under serum-free conditions. Scad J Immuno. 65: 581–588.
- Kawano, Y., Kobune, M., Yamaguchi, M., Nakamura, K., Ito, Y., Sasaki, K., Takahashi, S., Nakamura, T., Chiba, H., Sato, T., Matsunaga, T., Azuma, H., Ikebuchi, K., Ikeda H., Kato, J., Niitsu, Y., Hamada, H. (2003). Ex vivo expansion of human umbilical cord hematopoietic progenitor cells using a coculture system with human telomerase catalytic subunit (hTERT)-transfected human stromal cells. Blood 101: 532–540.
- Kempuraj, D., Saito, H., Kaneko, A., Fukagawa, K., Nakayama, M., Toru, H., Tomikawa, M., Tachimoto, H., Ebisawa, M., Akasawa, A., Miyagi T., Kimura, H., Nakajima, T., Tsuji, K., Nakahata, T. (1999). Characterization of mast cell-committed progenitors present in human umbilical cord blood. Blood 93: 3338–3346.
- Krauth, M.T., Böhm, A., Agis, H., Sonneck, K., Samorapoompichi, P., Florian, S., Sotlar, K., Valent, P. (2007). Effects of the CD33-targeted drug gemtuzumab ozogamicin (Mylotarg) on growth and mediator secretion in human mast cells and blood basophils. Exp Hematol 35: 108–116.
- Fehlner-Gardiner, C.C., Uniyal, S., von Ballestrem, C.G., Chan, B.M. (1996). Differential utilization of VLA-4 (α4β1) and -5 (α5β1) integrins during the development of mouse bone marrow-derived mast cells. Differentiation 60: 317–325.
- Fehlner-Gardiner, C.C., Uniyal, S., von Ballestrem, C.G., Dougherty, G.J., Chan, B.M. (1996). Integrin VLA-6 (α6β1) mediates adhesion of mouse bone-marrow-derived mast cells to laminin. Allergy 51: 650–656.
- Lorentz, A., Schuppan, D., Gebert, A., Manns, M.P., Bischoff, S.C. (2002). Regulatory effects of stem cell factor and interleukin-4 on adhesion of human mast cells to extracelluar matrix proteins. Blood 99: 966–972.
- Rennick, D., Hunte, B., Holland, G., Thompson-Snipes, L. (1995). Cofactors are essential for stem cell factor-dependent growth and maturation of mast cell progenitors: comparative effects of interleukin-3 (IL-3), IL-4, IL-10, and fibroblasts. Blood 85: 57–65.
- Yanagida, M., Fukamachi, H., Ohgami, K., Kuwaki, T., Ishii, H., Uzumaki, H., Amano, K., Tokiwa, T., Mitsui, H., Saito, H., Iikura, Y., Ishizaka, T., Nakahata, T. (1995). Effects of T-helper 2-type cytokines, interleukin-3 (IL-3), IL-4, IL-5, and IL-6 on the survival of cultured human mast cells. Blood 86: 3705–3714.
- Saito, H., Ebisawa, M., Tachimoto, H., Shichijo, M., Fukagawa, K., Matsumoto, K., Iikura, Y., Awaji, T., Tsujimoto, G., Yanagida, M., Uzumaki, H., Takahashi, G., Tsuji, K., Nakahata, T. (1996). Selective growth of human mast cells induced by Steel factor, IL-6, and prostaglandin E2 from cord blood mononuclear cells. J Immunol 157: 343–350.
- Yuan, Q., Gurish, M.F., Friend, D.S., Austen, K.F., Boyce, J.A. (1998). Generation of a novel stem cell factor-dependent mast cell progenitor. J Immunol 161: 5143–5146.
- Ochi, H., Hirani, W.M., Yuan, Q., Friend, D.S., Austen, K.F., Boyce, J.A. (1999). T helper cell type 2 cytokine-mediated comitogenic responses and CCR3 expression during differentiation of human mast cells in vitro. J Exp Med 190: 267–280.
- Welker, P., Grabbe, J., Gibbs, B., Zuberbier, T., Henz, B.M. (2000). Nerve growth factor-β induces mast-cell marker expression during in vitro culture of human umbilical cord blood cells. Immunology 99: 418–426.
- Bagga, S., Price, K.S., Lin, D.A., Friend, D.S., Austen, K.F., Boyce, J.A. (2004). Lysophosphatidic acid accelerates the development of human mast cells. Blood 104: 4080–4087.
- Price, M.M., Kapitonov, D., Allegood, J., Milstien, S., Oskeritzian, C.A., Spiegel, S. (2009). Sphingosine-1-phosphate induces development of functionally mature chymase-expressing human mast cells from hematopoietic progenitors. FASEB J 23: 3506–3515.
- Sellge, G., Lorentz, A., Gebhardt, T., Levi-Schaffer, F., Bektas, H., Manns, M.P., Schuppan, D., Bischoff, S.C. (2004). Human intestinal fibroblasts prevent apoptosis in human intestinal mast cells by a mechanism independent of stem cell factor, IL-3, IL-4, and nerve growth factor. J Immunol 172: 260–267.
- Mierke, C., Ballmaier, M., Werner, U., Manns, M.O., Welte, K., Bischoff, S.C. (2000). Human endothelial cells regulate survival and proliferation of human mast cells. J Exp Med 192: 801–811.