Abstract
Exsanguinating hemorrhage and unavailability of blood are major problems in pre-hospital trauma care. We investigated if combining rFVIIa with HBOC-201 reduces blood loss and improves physiologic parameters compared to HBOC alone. Swine underwent liver injury and were resuscitated with HBOC-201 alone or HBOC+90, 180 or 360 μg/kg rFVIIa before hospital arrival at 240 min; animals survived to 72 hours. Blood loss was reduced; MAP, CI, transcutaneous oxygen saturation, and 72-hour survival improved in the 90 and 180 μg/kg rFVIIa groups. Lactate was cleared faster in the HBOC+rFVIIa 90 μg/kg group. Verification in a large, well-powered study is indicated.
Hemorrhage remains the leading potentially preventable cause of death in trauma patients. It has been reported that about 40% of trauma fatalities are due to uncontrolled bleeding [Citation1]. In Operation Iraqi Freedom (OIF) and Operation Enduring Freedom (OEF), more than 80% of potentially survivable fatalities are due to hemorrhage, more than 50% of which occur prior to hospital evacuation [Citation2]. Thus, adequate resuscitation and hemostasis strategies and availability of blood transfusions during pre-hospital care are urgent unmet medical needs.
One method to improve pre-hospital resuscitation are hemoglobin-based oxygen carriers (HBOCs), some of which have characteristics of universal compatibility, ease of administration, and stability without refrigeration. In pre-clinical models of severe hemorrhagic shock (with and without concomitant brain injury), the second generation HBOC, HBOC-201 (Biopure; Cambridge, MA), improves systemic and neurophysiologic parameters and survival [Citation3–10]. Furthermore, in phase II–III trials in surgical patients, HBOC-201 administration resulted in decreased blood transfusion requirements [Citation11–15]. Adding a systemic pro-coagulant such as recombinant Factor VIIa (rFVIIa) has potential to improve hemostasis in the first place. Coagulopathy is seen in approximately one-third of trauma patients, which poses a clinical challenge [Citation16]. Trauma-associated coagulopathy is attributed to the dilutional effect of fluid resuscitation and transfusion, hypothermia, acidosis and release of mediators following tissue injury among other factors, and has been directly linked to mortality [Citation17]. Current treatment recommendations include empirical replacement therapy with low ratios of fresh frozen plasma (FFP), cryoprecipitate, and platelets (to Red Blood Cells [RBCs]), alongside with correcting acidosis and hypothermia [Citation18]. These steps, however, often fail to control life-threatening bleeding in trauma [Citation19]. Hence, we hypothesized that pre-hospital resuscitation of trauma casualties with uncontrolled hemorrhagic shock with a resuscitative fluid containing the oxygen carrier, HBOC-201, and the pro-coagulant, rFVIIa, would improve outcome. Herein, we report the results of a dose-escalation study assessing the physiologic effects of rFVIIa when added to HBOC-201.
rFVIIa (NovoSeven®, Novo Nordisk, Denmark) is a protease that enhances thrombin generation and increases stability of fibrin clot [Citation20]. rFVIIa has shown positive results in control of trauma bleeding. Two phase II randomized trials showed decreased RBC transfusion requirements and trends to reduced mortality and critical complications in blunt trauma patients; similar trends were apparent in penetrating trauma [Citation21–23]. In parallel, clinical trials assessing rFVIIa for intracranial hemorrhage (ICH, stroke) in which phase II trials suggested reduction in hematoma size and increase in survival [Citation24,Citation25], phase III trials confirmed reduced hematoma size, but failed to show decreased mortality and improvement in neurological outcome [Citation26]. We hypothesized that the absence of survival benefit in the ICH and trauma trials with rFVIIa may be due to delayed administration and that modification of treatment paradigms, including pre-hospital rather than in-hospital administration, might improve efficacy. In addition to timing, we noted that optimal dosing had not been previously evaluated rigorously either in preclinical or clinical studies, especially in trauma. Therefore, the objective of this dose-escalation swine study was to optimize rFVIIa dosing (in terms of hemorrhage, physiologic parameters, and tissue oxygenation) when added to HBOC-201 for pre-hospital resuscitation from uncontrolled hemorrhagic shock, in preparation for a subsequent, well-powered efficacy/safety study. Hematologic and immunologic results from this animal experiment were previously published [Citation27,Citation28].
MATERIALS AND METHODS
Animal Preparation
The experiments reported in the present study were conducted according to principles set in the “Guide for the Care and Use of Laboratory Animals,” Institute of Laboratory Animals Resources, National Research Council, National Academy Press, 1996. The study was approved by the Walter Reed Army Institute of Research/Naval Medical Research Institute of Research Institutional Animal Care and Use Committee; all procedures were performed in an animal facility approved by the Association for Assessment and Accreditation for Laboratory Animal Care International (AAALAC).
The experimental design has been previously reported [Citation27,Citation28]; because this report focuses on physiology results, it will be described in more detail here.
Thirty-two male and female Yucatan Mini pigs (∼25 kg; n=8 per group; Sinclair Research Center, Inc., Columbia, MO) were used. Feed was withheld 12 hours prior to initiation of experiments. Animals were sedated and anesthesia induced with 33 mg/kg bodyweight (bw) ketamine hydrochloride intramuscular (i.m.) injection, atropine sulfate and mask ventilation with isoflurane 3% and 100% O2 to facilitate endotracheal intubation. Experiments were started with animals breathing spontaneously. If apnea occurred, animals were mechanically ventilated to avoid unnecessary loss of animals using an Ohmeda 7800 series ventilator (Datex-Ohmeda, Madison, WI) at 5–10 ml/kg bw, 15–25 breaths/min and FiO2 at 0.21–1.0. Minute ventilation and FiO2 were titrated to maintain pCO2 between 35 and 40 torr and SaO2 > 92%. SaO2 was monitored by peripheral pulse oximetry (Datascope Corp., Mahwah, NJ). The hemorrhage was allowed to occur freely in the open abdomen, and care was taken to avoid any pressure caused by other organs (intestines, ventilated lungs) that would interfere with the uncontrolled hemorrhage.
Animal Instrumentation
Following appropriate sedation, analgesia, anesthesia, and endotracheal intubation (as described above), the right external jugular vein and carotid artery were dissected and isolated. A 9 French (Fr) introducer sheath was placed in the external jugular vein using the Seldinger technique and a 7.5 Fr pulmonary artery catheter (PAC; Edwards Life Sciences, Irvine, CA) was inserted for continuous hemodynamic and cardiac output (CO) monitoring. A 20 gauge angiocatheter was placed in the carotid artery and blood pressure (systolic, diastolic, and mean) was continuously transduced. Urine was collected via bladder catheterization. An upper midline abdominal incision was made that extended through the fascia. The peritoneum was opened and the liver visualized. The abdominal viscera were then packed with laparotomy pads to provide hepatic exposure. All surgical procedures were performed using aseptic techniques. No pre-operative antibiotics were administered.
Animal Groups and Randomization
Pigs were randomly allocated to one of the following groups (n=8 per group): HBOC-201 alone (control group); HBOC-201+90 μg/kg rFVIIa; HBOC-201+ 180 μg/kg rFVIIa; HBOC-201+360 μg/kg rFVIIa.
Preparation of HBOC/rFVIIa Fluids
HBOC-201 is purified, filtered, stroma-free and heat-treated bovine hemoglobin that is polymerized by gluteraldehyde-crosslinking to form polymers ranging from 130–500 kDa MW. It is prepared in a buffer similar to lactated Ringer's solution (LR) containing 13 g Hb/dL. Recombinant FVIIa is a recombinant form of the activated form of human factor VII (NovoNordisk, Copenhagen, Denmark). Each vial contains 2.4 mg of rFVIIa re-suspended in 4.3 ml sterile water to give a final concentration of 0.56 mg/ml. Each HBOC-201 bag (250 ml) was admixed with 2.25, 4.5, or 9 mg of rFVIIa, resulting in fluid concentrations of 9, 18, and 36 μg/ml, respectively. The appropriate number of bags was prepared under sterile conditions according to animal weight immediately before T0.
Liver Injury and Uncontrolled Hemorrhage
A standardized liver injury was created by placing a ring clamp over the midpoint of the left lobe one-fourth the distance from the left lobe apex, adjusting for relative size of the liver and weight of the pig as previously described [Citation3]. The clamp was completely closed, and an 11 blade was used to lacerate the lobe from the tip of the clamp through the remaining width. This denoted the start of the experiment (Time 0). After one minute, the clamp was removed and the remaining left lobe was sharply excised, resulting in a ∼ 25% lobectomy, consistent with a AAST grade III liver injury [Citation29]. No attempts at hepatic hemorrhage control were made to allow uncontrolled hemorrhage. Hemorrhage volume was directly aspirated below the wound edges and assessed by both volume and weight.
Resuscitation
Fifteen minutes into uncontrolled hemorrhage, animals were resuscitated with 10 ml/kg of control or experimental fluid. At 30 minutes, an additional infusion of 5 ml/kg was administered. Further infusions were provided at 60, 120, and 180 minutes post-injury for hypotension (mean arterial pressure (MAP) < 60 mm Hg) or tachycardia (HR > baseline value [Time 0] + 5%). Fluids were infused over 10 minutes at room temperature.
Simulated Hospital Arrival Care and Interventions
Hospital arrival was simulated at 4 hours. Animals were administered 10 ml/kg allogeneic, type matched fresh whole blood for anemia (Hb < 7 g/dL) or 20 ml/kg normal saline (NS) for hypotension (MAP < 70 mmHg). The liver injury was repaired using Surgicel absorbable hemostat (Johnson & Johnson, Somerville, NJ). The PAC was removed and the jugular vein introducer was secured for postoperative blood sampling and fluid administration. The arterial and bladder catheters were removed and areas repaired as necessary. Surgical incisions were closed and surgical dressings applied. Thirteen mg/kg cephazolin i.v. (HIKMA-Farmaceútica; Terrugen, Portugal) and 0.01 mg/kg buprenorphine i.m. (Rewckitt Benckiser Healthcare Ltd., Hull, England) were administered prior to extubation and recovery from anesthesia. Vital signs and general status were assessed 24, 48, and 72 hours post-injury. Animals received NS or whole blood as needed for hypotension or anemia. Antibiotics and analgesia was continued twice a day during the recovery period. Pigs were euthanized 72 hours post-injury for necropsy and histological analysis.
Transcutaneous Oxygen Monitor (TCOM)
Transcutaneous tissue oxygenation (tcpO2) was measured continuously throughout the experiment. Four Clark-type polarographic electrodes were positioned bilaterally on the upper torso and on the inner thighs and connected to a Radiometer TCM1 transcutaneous O2 monitor (Radiometer, Copenhagen, Denmark) for indirect, noninvasive measurement of tissue oxygenation.
Hematology and Immunology Parameters
In vitro coagulation and immunology assays to assess immunophenotyping, apoptosis and plasma cytokine levels have been performed. The methods and results were previously reported [Citation27,Citation28].
Statistical Analysis
The log-rank test was used to compare the survival functions between the treatment groups; survival proportions were compared using Fisher's Exact Test. Analysis of variance (ANOVA) and Kruskal-Wallis test were used to compare effects between he treatment groups at discrete time points. A two-tailed Student t test was used to assess intergroup differences. P-values < 0.05 (two-sided) were considered statistically significant.
RESULTS
Hemodynamics
There were no statistically significant differences in physiologic parameters between groups at baseline nor at the beginning of resuscitation; there was a trend to higher heart rate in the HBOC+rFVIIa 90 μg/kg group at the beginning of resuscitation. In all groups, MAP decreased during hemorrhage and was restored to near baseline values following resuscitation (). MAP was restored to baseline at 120 minutes in the HBOC group, at 45 minutes in the HBOC+rFVIIa 90 μg/kg group, and at 105 minutes in the HBOC+rFVIIa 180 μg/kg group, whereas it did not return to baseline until 195 minutes in the HBOC+rFVIIa 360 μg/kg animals (p=0.0424). Mean pulmonary artery pressure (MPAP) was similar between groups throughout the pre-hospital phase (p=0.49; ). No groups demonstrated pulmonary hypertension; MPAP surpassed baseline only mildly in all groups. Heart rate (HR) was significantly different between groups over time (p=0.0027; ). HR increased in all groups in response to hemorrhage, but normalized in response to resuscitation in all groups except in HBOC+rFVIIa 180 μg/kg. In this group, HR continued to increase until 120 minutes, at which point tachycardia began to resolve; in the other groups, tachycardia began to resolve by 60 minutes. Cardiac Index (CI) was lowest in HBOC only pigs throughout the pre-hospital phase, and never returned to baseline. CI was similar between other treatment groups and was restored to baseline at 135 minutes in the HBOC+rFVIIa 90 μg/kg group,195 minutes in the HBOC+rFVIIa 180 μg/kg group, and approached baseline in the HBOC+rFVIIa 360 μg/kg group by the end of the pre-hospital phase (p<0.0001; ).
Figure 1. Mean arterial pressure () and mean pulmonary artery pressure () decrease during the first 15 minutes post-injury, when no resuscitation is given. Following resuscitation, MAP was restored to baseline at 120 minutes in the HBOC group, at 45 minutes in the HBOC+rFVIIa 90 μg/kg group, and at 105 minutes in the HBOC+rFVIIa 180 μg/kg group, whereas it did not return to baseline until 195 minutes in the HBOC+rFVIIa 360 μg/kg animals (p=0.0424). Mean pulmonary artery pressure (MPAP) was similar between groups throughout the pre-hospital phase (p=0.49; ). No groups demonstrated pulmonary hypertension; MPAP surpassed baseline only mildly in all groups.

Figure 2. Heart rate in the HBOC+rFVIIa 90 μg/kg group was higher at the beginning of resuscitation and continued to increase until 120 minutes, at which point tachycardia began to resolve; in the other groups, tachycardia began to resolve by 60 minutes (). Cardiac Index (CI) was lowest in HBOC only pigs throughout the pre-hospital phase, and never returned to baseline. CI was similar between other treatment groups and was restored to baseline faster at 135 minutes in the HBOC+rFVIIa 90 μg/kg group than in the HBOC+rFVIIa 180 μg/kg (195 min.), and in the HBOC+rFVIIa 360 μg/kg groups (by the end of the pre-hospital phase (p<0.0001), ).
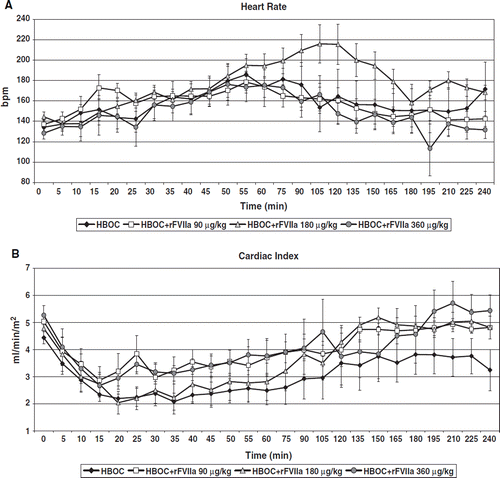
Hemorrhage Volume and rFVIIa Total Dose
There were no statistically significant differences in pre-hospital blood loss between treatment groups despite large differences in rFVIIa dose (p=0.0960; ). There was, however, a trend to reduced blood loss in the groups receiving doses of 90 (19.4±3.7 ml/kg) and 180 μg/kg (19.5±4.8 ml/kg) of rFVIIa compared to the highest dose of 360 μg/kg (33.0±7.5 ml/kg) or no rFVIIa 27.5±5.6 ml/kg).
Table 1. Pre-hospital blood loss, fluid requirements, urine output and rFVIIa doses.
Pre-Hospital Fluid Requirements
Pre-hospital fluid requirements are displayed in , and were not statistically different between groups, but there was a trend towards reduced resuscitation requirement in the HBOC-201 only group compared to rFVIIa groups.
Urine Output
All pigs produced urine throughout the pre-hospital phase. Total urine output was similar between groups ().
Indirect and Direct Measures of Tissue Oxygenation
Lactate increased in all groups in response to hemorrhage and remained above baseline in all groups with the exception of the HBOC+rFVIIa 90 μg/kg group (p=0.01; ). A substantial increase in lactate was observed in the HBOC+rFVIIa 360 μg/kg group at 150 and 180 minutes (p=0.05). Arterial O2 (SaO2) and mixed venous O2 (SvO2) saturations were similar between groups during the pre-hospital period. Arterial blood gas derived base excess (BE) was lowest in the HBOC+rFVIIa 180 μg/kg group from the start of resuscitation to 120 minutes and highest in the HBOC+rFVIIa 90 μg/kg group from 60 minutes. Due to technical problems with the blood analyzer, no BE data was collected for the HBOC+rFVIIa 360 μg/kg group. Although O2 delivery (DO2) was not significantly different between groups, it was consistently higher in the HBOC+rFVIIa 360 μg/kg group during the pre-hospital phase. No differences in O2 consumption (VO2) or O2 extraction ratio (O2ER) were observed. Transcutaneous oxygen saturation (tcpO2) was significantly higher over time in the animals receiving 90 or 180 μg/kg rFVIIa compared to 360 μg/kg or HBOC alone (p<0.0001; ).
Figure 4. Lactate () increased in all groups in response to hemorrhage and remained above baseline in all groups with the exception of the HBOC+rFVIIa 90 μg/kg group (p=0.01). It increased substantially in the HBOC+rFVIIa 360 μg/kg group at 150 and 180 minutes (p = 0.05). Transcutaneous oxygen saturation (tcpO2) was significantly higher over time in the animals receiving 90 or 180 μg/kg rFVIIa compared to 360 μg/kg or HBOC alone (p<0.0001; ).
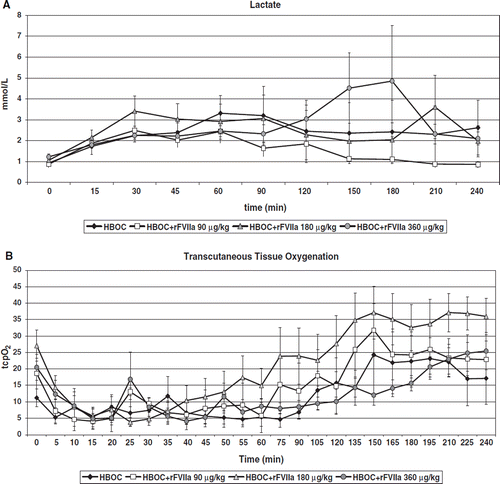
Survival
Pre-hospital survival (to simulated hospital arrival, 240 minutes) was 5/8 (62.5%), 6/8 (75%), 5/8 (62.5%), 6/8 (75%), and 17/24 (70.8%) in HBOC, HBOC+rFVIIa 90 μg/kg, HBOC+rFVIIa 180 μg/kg, HBOC+rFVIIa 360 μg/kg, and all rFVIIa groups-combined groups. Respective survival rates to 72 hours were 2/8 (25%), 5/8 (62.5%), 5/8 (62.5%), 4/8 (50%) and 14/24 (70.8%) (p=0.09 log-rank in the HBOC-201+90 μg/kg rFVIIa group versus HBOC-201 alone, ).
Figure 5. Survival to simulated hospital arrival (240 minutes) 5/8 (62.5%), 6/8 (75%), 5/8 (62.5%), 6/8 (75%) and 17/24 (70.8%) in HBOC, HBOC+rFVIIa 90 μg/kg, HBOC+rFVIIa 180 μg/kg, HBOC+rFVIIa 360 μg/kg and all rFVIIa groups-combined groups. Respective survival rates to 72 hours were 2/8 (25%), 5/8 (62.5%), 5/8 (62.5%), 4/8 (50%) and 14/24 (70.8%), p = 0.09.
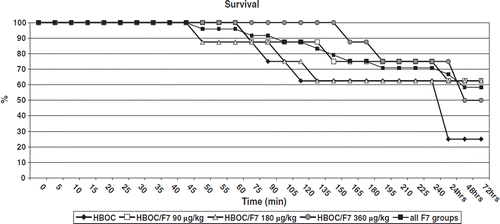
DISCUSSION
An optimal dose of rFVIIA for addition to HBOC-201 resuscitation was identified in this swine model of severe uncontrolled hemorrhage with prolonged delay to hospital arrival. Up to five doses of 90 μg/kg of rFVIIa added to HBOC-201 (totaling up to 270 μg/kg) resulted in improved cardiac output and direct (tcPO2) and indirect measures of tissue oxygenation (lactic acidosis, and base excess). These results add to previously published trends of decreased blood loss, prothrombin time, thromboelastography reaction time, improved survival, and negligible immunologic changes with 90 μg/kg rFVIIa [Citation27,Citation28]. Collectively, the results supported the hypothesis that rFVII added to HBOC-201 decreased blood loss and improved physiologic parameters and survival in this model.
Intrinsic levels of FVIIa are reduced among other factors after fluid resuscitation for massive hemorrhage and plasma levels reliably increase following intravenous administration [Citation27]. Efficacy of rFVIIa appears to be closely related to pH, temperature optima, and severity of hemorrhagic shock. That timing of administration of rFVIIa also supports our approach of early administration [Citation30]. Other factors possibly impacting rFVIIa efficacy remain undefined. Regardless, enabling local clot formation (e.g., with rFVIIa) without depleting O2 content (blood transfusion or HBOC) is a suboptimal resuscitation strategy.
At present, there is no established dose of rFVIIa that can be universally applied to injured patients. Some centers have adopted a nihilistic approach and provide all trauma patients with refractory hemorrhage 2 vials of rFVIIa as a standard dose without adjusting for body weight [Citation31]; the wisdom of either approach remains unclear. The administration of additional doses in our study was appropriate in the setting of uncontrolled hemorrhage, since this is a 4-hour delay to hospital arrival, and this phase exceeded the known half life of rFVIIa (2.5 hours). This study differs from current civilian clinical practice in that rFVIIa was evaluated for pre-hospital use, more than one additional dose was provided within the pre-hospital study period, and urban and suburban transport times are usually short by comparison; the value of repeated dosing has yet to be confirmed. The rationale for delivering subsequent doses of the HBOC/rFVIIa resuscitation solution at 5 ml/kg bw derived from the pharmacodynamics of each agent. This dose is consistent with the colloidal nature of the HBOC (small volume resuscitation due to plasma space retention) as well as the unclear benefit of repeating the initial dose of the rFVIIa within the agent's half-life (t 1/2 ∼ 2.5 hours). Nonetheless, the animals in the rFVIIa arms received a “supra-therapeutic” dose of rFVIIa. Although reduced hemorrhage volume was anticipated, no robust statistically significant changes in pre-hospital blood loss were observed, particularly at the highest doses. This observation may reflect a threshold past which further addition of rFVIIa may have not enhanced clotting kinetics in the absence of traumatic hypothermic coagulopathy and some plasma factors due to hemodilution [Citation27]. However, that there were trends towards reduced blood loss with 90 and 180 μg/kg rFVIIa dosing merits further investigation in a definitive, well-powered study. More detailed discussion about why blood loss was not significantly reduced in this study can be found in a recent publication by Arnaud et al. [Citation27]. The 24-hour mortality in the HBOC only animals was higher, but not statistically significant than in the rFVIIa groups. Some of these animals never recovered their blood pressure adequately. Some animals had cumulative higher blood loss; however, this was not necessarily related to increased mortality. In reviewing the histopathology data we could not find a relation between myocardial ischemia and death in the HBOC animals (data will be published separately). Indirect measure of tissue perfusion with transcutaneous measurement did not reveal significant differences. Hemorrhage was controlled at 240 minutes, and no animal was bleeding after the liver injury was repaired.
While indices of oxygen dynamics (O2 delivery, O2 consumption, lactate, and base excess) were identical between groups at four hours, there was more rapid reversal of anaerobic metabolism in the HBOC+rFVIIa 90 µg/kg group. This group had the lowest lactate levels throughout the pre-hospital phase and improved tcPO2. Similarly notable is the more rapid correction of base excess from negative to positive in this group. Whether the improved CO and direct and indirect measures of tissue oxygenation in the HBOC+rFVIIa 90 µg/kg group were improved due to decreased hemorrhage or other consequences of rFVIIa administration is unclear. Interestingly, in an isolated brain injury experimental model we also observed improved lactic acidosis in swine administered rFVIIa (unpublished data). In another brain injury model in swine, rFVIIa reduced contusion volume and had unexpected neuroprotective effects [Citation32]. While tantalizing to attribute these observed benefits to rFVIIa in a manner analogous to the fortuitously discovered neuroprotective and pleuripotent effects of recombinant human erythropoietin [Citation33], a larger study will need to address these trends.
The current study has several limitations. First, while prospective, the investigators were not blinded to rFVIIa concentrations; however, to minimize bias, administration was protocol-rather than clinician-driven. Second, animals sustained an isolated hepatic tissue crush/laceration injury in the open abdomen. While this may parallel the tissue injury-incited cytokine storm and immunologic and inflammatory changes associated with isolated abdominal injury and hemorrhage, some injured combatants may sustain polytrauma or blunt trauma. Furthermore, this model does not account for blast injury, an injury mechanism that is increasingly prevalent [Citation34]. While the open abdomen is necessary for measuring blood loss, possible tamponade effects in the closed abdomen are not considered in this model. Third, animals were anesthetized when injured, thus abrogating the high catecholamine state that typically precedes violent injury. Lower catecholamine tone may impact hemorrhage and HBOC vasoactivity. This may have made our model less lethal than anticipated, and masked some of the differences between groups. Fourth, animals were maintained at normothermia, requiring rFVIIa to work in a suboptimal microenvironment. It is possible that hypothermia as well as more severe pH derangements may have similarly uncovered group differences. Also, while survival trends seem improved with rFVIIa dosing, the mechanism could not be identified in this study and may be independent of hemorrhage reduction. Finally, although the military relevance of this study could be questioned because the rFVIIa product tested required refrigeration, a room-temperature stable product has been approved for clinical use [Citation35].
CONCLUSION
We found that in swine with severe hemorrhagic shock due to solid organ injury, low but not high dose rFVIIa, when added to HBOC-201, improves cardiac output and anaerobic metabolism. As previously published [Citation27,Citation28], there were trends to improved hemorrhage volume and survival and minimal immune activation. There appears to be no benefit to increasing the initial dose of rFVIIa above 90 µg/kg when it is followed by repeat dosing within the rFVIIa half-life. If these trends are realized as statistically valid and clinically relevant in a large, well-powered study and subsequent clinical trial, combatant and seriously injured civilian rescue with a mix of oxygen carrier and hemostatic agent may represent the future of injury resuscitation.
Declaration of interest: Dr. Lewis Kaplan is a consultant for BioPure. Dr. Aryeh Shander is a consultant for and received research grants from NovoNordisk. I am a military service member (or employee of the U.S. Government). This work was prepared as part of my official duties. Title 17 U.S.C. §105 provides that ‘Copyright protection under this title is not available for any work of the United States Government.’ Title 17 U.S.C. §101 defines a U.S. Government work as a work prepared by a military service member or employee of the U.S. Government as part of that person's official duties.
REFERENCES
- Butler, F. K., Jr., Holcomb, J. B., Giebner, S. D., McSwain, N. E., Bagian, J. (2007). Tactical combat casualty care 2007: evolving concepts and battlefield experience. Mil Med 172: 1–19.
- Kelly, J. F. . (2008). Injury severity and causes of death from Operation Iraqi Freedom and Operation Enduring Freedom: 2003-2004 versus 2006. J Trauma 64: S21–26; discussion S26–27.
- Gurney, J. . (2004). A hemoglobin based oxygen carrier, bovine polymerized hemoglobin (HBOC-201) versus Hetastarch (HEX) in an uncontrolled liver injury hemorrhagic shock swine model with delayed evacuation. J Trauma 57: 726–738.
- Katz, L. M. . (2002). HBOC-201 improves survival in a swine model of hemorrhagic shock and liver injury. Resuscitation 54: 77–87.
- Manning, J. E. . (2000). Bovine hemoglobin-based oxygen carrier (HBOC-201) for resuscitation of uncontrolled, exsanguinating liver injury in swine. Carolina Resuscitation Research Group. Shock 13: 152–159.
- Patel, M. B., Feinstein, A. J., Saenz, A. D., Majetschak, M., Proctor, K. G. (2006). Prehospital HBOC-201 after traumatic brain injury and hemorrhagic shock in swine. J Trauma 61: 46–56.
- Stern, S. . (2008). Resuscitation with the hemoglobin-based oxygen carrier, Hboc-201, in a swine model of severe uncontrolled hemorrhage and traumatic brain injury. Shock 2009 Jan 31(1): 64–79.
- Rosenthal, G. . (2008). Use of hemoglobin-based oxygen-carrying solution-201 to improve resuscitation parameters and prevent secondary brain injury in a swine model of traumatic brain injury and hemorrhage: laboratory investigation. J Neurosurg 108: 575–587.
- Dudkiewicz, M., Harpaul, T. A., Proctor, K. G. (2008). Hemoglobin-based oxygen carrying compound-201 as salvage therapy for severe neuro- and polytrauma (Injury Severity Score = 27-41). Crit Care Med 36: 2838–2848.
- King, D. R., Cohn, S. M., Proctor, K. G. (2005). Resuscitation with a hemoglobin-based oxygen carrier after traumatic brain injury. J Trauma 59: 553–560; discussion 560–552.
- LaMuraglia, G. M. . (2000). The reduction of the allogenic transfusion requirement in aortic surgery with a hemoglobin-based solution. J Vasc Surg Feb 31(2): 299–308.
- Levy, J. H. (2003). The use of haemoglobin glutamer-250 (HBOC-201) as an oxygen bridge in patients with acute anaemia associated with surgical blood loss. Expert Opin Biol Ther 3: 509–517.
- Levy, J. H. . (2002). Polymerized bovine hemoglobin solution as a replacement for allogeneic red blood cell transfusion after cardiac surgery: results of a randomized, double-blind trial. J Thorac Cardiovasc Surg Jul 124(1): 35–42.
- Sprung, J. . (2002). The use of bovine hemoglobin glutamer-250 (Hemopure) in surgical patients: results of a multicenter, randomized, single-blinded trial. Anesth Analg Apr 94(4): 799–808.
- Standl, T. . (1998). Bovine haemoglobin-based oxygen carrier for patients undergoing hemodilution before liver resection. Br J Anaesth Feb 80(2): 189–194 (1998).
- Brohi, K., Singh, J., Heron, M., Coats, T. (2003). Acute traumatic coagulopathy. J Trauma Jun 54(6): 1127–1130.
- MacLeod, J. B., Lynn, M., McKenney, M. G., Cohn, S. M., Murtha, M. (2003). Early coagulopathy predicts mortality in trauma. J Trauma 55: 39–44.
- Borgman, M. A. . (2007). The ratio of blood products transfused affects mortality in patients receiving massive transfusions at a combat support hospital. J Trauma 63: 805–813.
- Rizoli, S. B. . (2006). Recombinant activated factor VII as an adjunctive therapy for bleeding control in severe trauma patients with coagulopathy: subgroup analysis from two randomized trials. Crit Care Dec 21; 10(6): R178 (2006).
- Monroe, D. M., Hoffman, M., Oliver J. A., Roberts, H. R. (1998). A possible mechanism of action of activated factor VII independent of tissue factor. Blood Coagul Fibrinolysis Suppl 1: S15–S20.
- Boffard, K. D. . (2005). Recombinant factor VIIa as adjunctive therapy for bleeding control in severely injured trauma patients: two parallel randomized, placebo-controlled, double-blind clinical trials. J Trauma 59: 8–15; discussion 15–18.
- Felfernig, M. (2007). Clinical experience with recombinant activated factor VII in a series of 45 trauma patients. J R Army Med Corps 153: 32–39.
- Geeraedts, L. M., Jr. . (2005). The role of recombinant factor VIIa in the treatment of life-threatening haemorrhage in blunt trauma. Injury 36: 495–500.
- Mayer, S. A. . (2006). Recombinant activated factor VII for acute intracerebral hemorrhage: US phase IIA trial. Neurocrit Care 4: 206–214.
- Diringer, M. N. . (2008). Risk of thromboembolic events in controlled trials of rFVIIa in spontaneous intracerebral hemorrhage. Stroke 39: 850–856.
- Mayer, S. A. . (2008). Efficacy and safety of recombinant activated factor VII for acute intracerebral hemorrhage. N Engl J Med 358: 2127–2137.
- Arnaud, F. . (2008). Hematologic effects of recombinant factor VIIa combined with hemoglobin-based oxygen carrier-201 for prehospital resuscitation of swine with severe uncontrolled hemorrhage due to liver injury. Blood Coagul Fibrinolysis 19: 669–677.
- Malkevich, N. V. . (2008). Innate immune response after resuscitation with hemoglobin-based oxygen carrier and recombinant factor VIIA in uncontrolled hemorrhagic shock in a swine model. J Trauma 64: 1498–1510.
- Moore, E. E. . (1995). Organ injury scaling: spleen and liver (1994 revision). J Trauma 38: 323–324.
- Stein, D. M., Dutton, R. P., Kramer, M. E., Handley, C., Scalea, T. M. (2008). Recombinant factor VIIa: decreasing time to intervention in coagulopathic patients with severe traumatic brain injury. J Trauma 64: 620–627; discussion 627–628.
- Stein, D. M., Dutton, R. P., O'Connor, J., Alexander, M., Scalea, T. M. (2005). Determinants of futility of administration of recombinant factor VIIa in trauma. J Trauma 59: 609–615.
- Zhang, J. . (2008). Hemostatic and neuroprotective effects of human recombinant activated factor VII therapy after traumatic brain injury in pigs. Exp Neurol 210: 645–655.
- Ehrenreich, H., Bartels, C., Sargin, D., Stawicki, S., Krampe, H. (2008). Recombinant human erythropoietin in the treatment of human brain disease: focus on cognition. J Ren Nutr 18: 146–153.
- Owens, B. D. . (2008). Combat wounds in operation Iraqi Freedom and operation Enduring Freedom. J Trauma 64: 295–299.
- Nedergaard, H. . (2008). In vitro stability of lyophilized and reconstituted recombinant activated factor VII formulated for storage at room temperature. Clin Ther 30: 1309–1315.